The sequential EEG patterns
Definition of EEG patterns
1. Discrete seizures
Focal low-voltage fast activity which increases in amplitude, spreads across the midline, and then slows in discharge frequency.
Generalized muscle artifacts during the tonic seizure are followed by periodic muscle artifacts as the tonic activity converts to clonic jerks. With the end of the last clonic jerk, low-voltage slow activity appears and continues until the development of the next discrete seizure.
2. Merging seizures with waxing and waning amplitude and frequency of EEG rhythms
Rhythmic but frequently asymmetric sharp wave or spike/wave patterns with recurrent build-up and subsequent slowing of frequency and waxing and waning of amplitude.
There is no intervening low-voltage slow activity, as in the discrete seizure pattern. The rhythmic build-up is sometimes associated with overt generalized seizures. More commonly focal intermittent tonic and/or clonic convulsive activity is seen.
3. Continuous ictal activity
Rhythmic, relatively constant and frequently asymmetric, sharp wave or spike/wave discharges.
Such discharges are associated with either continuous generalized clonic jerks, or only subtle clonic movements. Rarely, only diffuse, continuous, rhythmic slowing appears during status.
4. Continuous ictal activity punctuated by low-voltage ‘flat periods’
The continuous ictal discharges described in continuous ictal activity can be punctuated by brief (0.5–8 s) episodes of generalized flattening on the EEG. Although the epileptiform discharges sometimes were asymmetric, the flat periods are always generalized.
This EEG pattern can be associated with overt or subtle focal clonic movements or no motor symptoms at all.
5. Periodic discharges on a suppressed background
Bilateral, sometimes asymmetric, high-voltage, monomorphic, repetitive sharp waves on a relatively flat background.
This can rarely be the initial pattern, and can also be seen in partially treated patients before status is completely controlled.
Etiology
The underlying causes for GCSE are diverse and cannot be determined in up to a third of subjects [16]. Nonetheless, there is a large range of well-studied factors that can lead to GCSE. Etiology is recognized as the most important prognostic factor (see Chap. 10). Studies of the clinical impact of SE etiology face several challenges, not least because in early studies, agreement on definitions of epilepsy and acute seizures was lacking. Also, etiologic classifications promulgated by the International League Against Epilepsy (ILAE) for seizures and epilepsy syndromes [31] were not applied, or were used incorrectly. Hence, variations in analyses of risk factors, etiologies, and outcome predictors in SE and GCSE have led to confusion, and epidemiologic measures are often misstated. Subsequently, the ILAE Commission on Epidemiology and Prognosis has established guidelines for epidemiologic research in epilepsy [31], classifying SE into acute symptomatic, remote symptomatic, progressive symptomatic, idiopathic, or cryptogenic types, based on determination of the underlying etiologies.
In general, two main etiologic groups of GCSE can be differentiated: GCSE on the basis of a known epileptic seizure disorder and GCSE as a consequence of (in decreasing frequency) earlier cerebrovascular disease, degenerative disorders, or metabolic, hypoxic, or infectious illnesses. In a systematic review of the frequency and outcome of GCSE, the most common underlying causes were cerebrovascular disease and low antiseizure drug levels [4].
In a population-based study in Virginia [1] and other investigations in SE [13], patients with anoxia or with several comorbidities have the highest mortality rates. Patients with other acute etiologies such as ischemic strokes, tumors, and traumatic brain injury, have an intermediate mortality rate. Alcohol-related GCSE seems to have an intermediate or low mortality, and patients with previous epilepsy in whom GCSE develops due to an exacerbating factor generally have the lowest mortality [4, 13].
According to a recent study of 126 patients with GCSE, acute symptomatic etiology was found in 59% [15]. Patients with treatment-refractory GCSE had a higher proportion of underlying CNS infections than patients with nonrefractory GCSE (44.4% vs. 23.5%), and notably, viral encephalitis was significantly more common in refractory GCSE than in nonrefractory cases (31% vs. 6.2%).
Less frequent but still significant etiologies of GCSE are: uncontrolled epilepsy, intoxication (especially with alcohol and illicit drugs), brain tumors, and traumatic brain injury [1, 6, 16, 32]. Table 7.2 presents the causes of GCSE in relation to outcome in patients with and without epilepsy.
Table 7.2
Etiology of generalized convulsive status epilepticus in relation to outcome in patients with and without epilepsy
Etiology of generalized convulsive status epilepticus | Outcome | ||
---|---|---|---|
Favorable | Increased morbidity | Increased mortality | |
Prior epilepsy | +++ | + | + |
Acute symptomatic | + | ++ | ++ +++ for hypoxic brain injury |
Remote symptomatic | ++ | + | + |
Progressive neurologic disease | ++ | ++ | + |
Unknown | +++ | ++ | + |
In critically ill patients, myoclonus is observed frequently and often raises concerns for underlying seizures or SE, but some types of myoclonus do not represent or end in seizures. While myoclonus generated from subcortical, spinal, and peripheral sites usually does not transform into seizures, cortical myoclonus can convert into seizures and GCSE regardless of etiology [33, 34]. There is evidence that the antiepileptogenic potential or effectiveness of the most frequently used drugs, such as diazepam, lorazepam or phenytoin may vary especially in the context of symptomatic seizures [35] and that treatment directed towards the underlying etiology can be key to seizure control.
Prior Epilepsy
One of six patients with epilepsy will suffer from at least one episode of SE in the course of a lifetime [8, 9]. Almost half of patients with SE and known epilepsy have already had a previous episode of SE [3]. According to different studies, the proportion of patients with SE who have previously known epilepsy ranges between 40 and 70% [1, 3, 36, 37].
In patients with GCSE, there is a prior history of epilepsy in more than two-thirds of cases [16]. Mortality in patients with GCSE and a history of epilepsy is 6% [16]. SE in adults is rarely the initial manifestation of epilepsy [3, 8, 36]. In particular, elderly SE patients usually have no seizure history [1, 3].
While NCSE in the form of absence or complex partial SE usually occurs in patients with known epilepsy [32], GCSE commonly emerges in association with causes other than prior epilepsy, such as with trauma, cerebrovascular, metabolic, anoxic or neoplastic insults, even in patients with known epilepsy [16]. Interestingly, there are differences in outcome between GCSE patients with and without an underlying epileptic disorder: patients with earlier epilepsy had favorable neurologic outcomes in over 80% of cases, and a mortality of <10% [16], while patients without reported epilepsy had favorable outcomes at discharge in less than 60% of cases, with a mortality exceeding 20% [16].
In patients with known epilepsy and no acute structural brain insult, several different scenarios may trigger GCSE. Alcohol use or withdrawal, intercurrent illness, sleep deprivation, and noncompliance with antiseizure medication appear to be the most common [1, 16]. Although many cases of GCSE in epilepsy patients are due to antiseizure drug reduction or withdrawal [4], acute neurologic diseases should also be considered in this context; cerebrovascular insults, CNS infections, or traumatic brain injury can be precipitants or causes for GCSE in patients with epilepsy [3, 16].
Acute and Remote Neurologic Illness
Cerebrovascular Disease. Strokes (either ischemic or hemorrhagic) are an uncommon but serious precipitant of GCSE, accounting for up to 20% of all types of SE [36, 38, 39]. In patients with GCSE, acute strokes are the precursor in 8% [16]. In the elderly, in whom vascular disease is more common, strokes account for >50% of SE and should be sought as the cause [1, 9, 32, 40, 41]. SE after strokes is usually categorized according to the time between the stroke and SE onset: “early-onset” SE emerges within 7 days of the stroke; “late-onset” SE occurs at least 8 days after the stroke. Early-onset SE following stroke has a prevalence between 2 and 6% of patients with SE. In a study of SE following acute stroke, ischemic and posterior cerebral artery strokes were the most frequent stroke types in patients with late-onset SE, while stroke types were evenly spread among patients with early-onset SE [41]. Of 121 patients in one study, post-stroke SE occurred in 30 patients with SE (24.8%), and 30% of SE was GCSE. In the early-onset SE group, however, NCSE was more common than GCSE. Another study showed that SE more commonly occured in stroke patients with higher disability scores, with 9% of post-stroke seizure patients having SE. In that study, the risk of SE was not associated with type or cause of stroke, or with lesion size or cortical involvement [38]. In a study using the US Nationwide Inpatient Sample over an 8-year period with 718,531 hospitalizations for acute ischemic stroke, 1415 patients (0.2%) developed GCSE [42]. Of 102,763 patients admitted to hospital with intracranial hemorrhage, GCSE developed in 266 (0.3%). In-hospital mortality was significantly higher in those with GCSE and acute ischemic stroke or intracranial hemorrhage [42].
In contrast to subarachnoid hemorrhage, intracerebral hemorrhage (ICH) led to a significant risk of early-onset seizures [39]. ICH-related SE can be seen in up to 20% of patients [42–44]. Cortical involvement and hemorrhagic transformation of an ischemic infarct were predictive of early seizures [45, 46]. SE is a potentially lethal complication of acute stroke, but early seizures are not clearly associated with increased mortality [39, 46–51]. A study analyzing the types of stroke-related SE showed that NCSE was the predominant SE type in the early-onset group and frequent in the late-onset group, underscoring the need for clinical awareness and the importance of EEG monitoring in stroke patients with altered levels of consciousness, especially in the early post-stroke phase and even in the absence of convulsions [41].
Brain Tumors. Status epilepticus can originate from a variety of cerebral tumors, with inconsistent impact on morbidity [36, 52, 53]. There are scant data regarding the incidence and prevalence of GCSE linked to brain tumors, and most derive from cohorts with a large variety of types and locations of tumors. In a review of malignant gliomas, tumor-related SE was often refractory but was not generalized convulsive in type [52]. Primary brain tumors are more likely to generate focal SE than GCSE [4]. Conversely, a large epidemiologic study identified tumors accounting for almost 2% of GCSE [6]. Another large epidemiologic investigation found brain tumors linked to 7% of all SE [54]. Patients with brain tumor-related epilepsy appear less likely to develop tumor-associated SE than are other epilepsy patients to develop SE [53]. Tumor-associated SE occurs more commonly when the tumor is in the frontal lobes. In contrast to tumor-associated epilepsy, where seizures commence early in the course of the disease, tumor-associated SE more often appears later. In patients with malignant gliomas, seizures are common, in 20–40% at presentation, and patients with seizures at presentation appear to have a greater risk of subsequent SE [52].
Head Injuries. Craniocerebral injuries are responsible for up to 26% of all types of SE [55], but this may include both remote and acute traumatic brain injuries (TBI). In up to 10% of patients with GCSE, TBI is thought to be the main etiology [16]. The risk of seizures after TBI correlates with the severity of the trauma and the time since the injury. Even unprovoked seizures emerging several years after serious injuries may be attributable to the trauma, but data from several studies suggest that mild TBI accompanied by a brief episode of unconsciousness or amnesia (but not associated with significant brain contusion, intracranial hematoma, or fracture) does not increase the risk of posttraumatic seizures [56–58].
Numerous studies have examined the effectiveness of posttraumatic antiseizure prophylaxis. There is evidence that antiseizure medication reduces early symptomatic seizures effectively, but a favorable impact on mortality was not detected [35, 59]. Also, the development of late posttraumatic seizures and SE cannot be prevented by prophylactic administration of antiseizure drugs [35, 60]. Further studies are needed to clarify if early and intensive seizure suppression may improve long-term outcome in this population.
Metabolic Disorders. Acute metabolic derangements account for 11.5% of all causes of SE in general, and SE with this cause has a mortality of about 30% [61]. Acute metabolic derangements are the presumed causes of about 4–26% of SE [13, 62–64], with a mortality of up to 25% [64]. Patients with acute metabolic disorders and GCSE were significantly more likely to require mechanical ventilation, and patients who required mechanical ventilation had a 7.43% mortality, compared to 2.22% for those who did not [17].
Systemic Illness
Infection and Inflammation. Meningitis and encephalitis are relatively rare causes of GCSE. In two large population-based series, primary CNS infections accounted for only 0.6% and 3% of SE in general [6, 54]. In a study of patients with GCSE, encephalitis and meningitis were the presumed underlying etiology in 3% [16]. Diagnosis of CNS infections in patients with GCSE can be challenging because patients may have blood and cerebrospinal fluid leukocytosis unrelated to an underlying infectious disease [36]. Peripheral leukocytosis is often caused by the demargination of white blood cells as a consequence of SE.
The mechanisms of neuronal damage and further seizure promotion are closely related to the immune response to pathogens, mediated by the release of cytokines. In brief, clinical and experimental studies have uncovered several mechanisms as integral parts of a bidirectional relationship between SE and inflammation. Systemic and local inflammation caused by SE and CNS infection or inflammation, or the cytotoxic effect of accumulation of the neurotransmitter glutamate as the result of ongoing seizures may contribute to sustaining SE [65–67]. Systemic inflammatory reaction induced by prolonged seizures is mirrored by increased serum cytokine levels, circulating immune cells, and the disruption of the blood–brain barrier (BBB) [67, 68] independent of infections [69]. Altered BBB increases the permeability for ions and proteins, facilitating transmigration of inflammatory cells that contribute to sustained seizures [70]. Furthermore, cytokines that promote the release of neuroactive molecules (e.g., glutamate, nitric oxide, neurotrophins) from glia or the endothelium [71, 72] or activate neuronal receptors in the CNS may modulate neuronal activity [73, 74].
Infectious Encephalitides. Data regarding GCSE associated with infectious encephalitis are scant. In one study of 236 GCSE patients, encephalitis or meningitis was diagnosed in 7% [16]. Other series have very limited sample sizes, so further characterization of patients with GCSE and infectious encephalitides is lacking. In another study of prognosis and predictors of outcome of refractory GCSE, viral encephalitis was significantly more common in refractory SE than in nonrefractory SE [15], confirming the findings of another study of GCSE treatment in which viral encephalitis was associated with treatment refractoriness [75].
Autoimmune Encephalitides. Along with the recent increased interest in antibody-mediated autoimmune encephalitis, current investigations often focus on the analysis of immune-mediated SE, but data regarding GCSE associated with autoimmune encephalitis in adults are scarce. Seizures in patients with multiple sclerosis (MS) are more frequent than are seizures in the general population [76, 77]. Partial-onset seizures are likely related to the focal or multifocal nature of the subcortical demyelinating lesions. In contrast, GCSE related to MS appears to be rare—with about 1% of patients with GCSE having MS [6, 16]. In a review of 268 MS patients, one of 20 patients with seizures had GCSE [76]. In contrast, in a large study of more than 5000 patients with MS, 1% had seizures, and GCSE was not detected [77]. While focal motor SE can be a serious complication of MS, GCSE appears to be rare.
Another treatable form of autoimmune encephalitis sometimes accompanied by refractory GCSE is characterized by antibodies against the GABAA-receptor. In a study analyzing serum and cerebrospinal fluid (CSF) samples of 140 encephalitis patients with seizures or SE, high titers of serum and CSF GABAA-receptor antibodies were associated not only with severe encephalitis, but also with treatment-refractory GCSE; the mechanism is thought to be reduction of synaptic GABAA-receptors [78]. The recognition of this disease is important, as it is potentially treatable and often occurs with GABA-ergic and other co-existing autoimmune disorders [79]. (See also Chap. 8, “Unusual Causes of Status Epilepticus”.)
Intoxications. Alcohol is probably the toxin most commonly associated with GCSE. The immediate effect of ethanol is mediated by its interference with glutamate, increasing the binding of glutamate to N-methyl-d-aspartate (NMDA) receptors. Long-term abuse causes adaptive changes within the NMDA receptor and leads to inhibition. Homocysteine levels, which increase during alcohol intake, increase further during abrupt alcohol withdrawal, possibly promoting alcohol withdrawal seizures [80]. In addition, alcohol interferes with antiseizure medication by inducing liver metabolism, leading to more rapid metabolism of some antiseizure drugs [80]. Alcohol was identified as a potential trigger for SE in 13% of patients in one large population-based study [54], and chronic alcohol abuse was reported in more than 8% of cases in another population-based GCSE study [6]. In another study of 249 GCSE patients, 11% had alcohol abuse as the only identifiable SE trigger [81]. Most patients with alcohol-related GCSE recovered without new neurologic deficits [82]. Benzodiazepines are the most effective antiseizure drugs for the primary and secondary prevention of alcohol withdrawal seizures [83].
Overdose of several prescription drugs can result in SE (e.g., theophylline, isoniazid, amitriptyline, thioridazine, pentazocine, lithium, and baclofen) [36, 37], but data from large studies are lacking. Several recreational drugs, such as cocaine, amphetamine, and heroin are additional but rare causes of SE. Seizures may occur not only in association with chronic abuse but also with first-time intake [37, 84]. In contrast to NCSE [85], data regarding GCSE in relation to other intoxications are scarce.
Precipitants
The Range of Potential Provoking Factors
Distinguishing between causative and triggering factors of GCSE is often difficult. Acute strokes, for example, are serious events characterized by especially poor outcome compared to that of other etiologies and may cause SE in patients with or without prior seizures [37, 39]. In contrast, patients who develop SE in the context of alcohol withdrawal or an acute head injury are more likely to have a history of seizures [3]. These observations call for differentiating between precipitants and etiologic factors. Events that, in the absence of a predisposition, would presumably not have caused SE by themselves could be labeled as “precipitants” in association with an underlying epilepsy. Sometimes more than one acute precipitant can be present in a case of SE [3, 9]. The observation that patients with SE from an acute neurologic insult have a history of epilepsy more frequently than do those in the general population, suggests that a history of seizures accompanies these etiologic risk factors [3]. Furthermore, there is a change in the types of precipitants according to age; children more frequently have infections and fever [9, 86].
Precipitants in Patients with Epilepsy
In patients with epilepsy, some triggering risks are low antiseizure drug levels, sudden discontinuation of antiseizure medication or insufficient therapeutic dose adjustment, alcohol intake, and acute critical illness and fever [1, 16, 37, 87]. Nevertheless, the definite precipitant remains unknown in a third of patients [16].
Acute Neurologic and Systemic Complications
Acute manifestations and complications of patients with GCSE can be categorized as neurologic or systemic. While most neurologic manifestations of GCSE result from ictal or postictal brain dysfunction or both as a direct consequence of cytotoxic damage to glia and neurons during seizures or seizure-related brain inflammation, the clinician should also be aware of other causes including structural, infectious, and metabolic disturbances of the CNS.
Acute systemic complications are frequent and can have serious impact on the course and outcome of GCSE. Pathophysiologically, most acute systemic complications ultimately result from the persistence of generalized convulsions. Intense muscle contractions may lead to an increase in body temperature, increased serum potassium levels, a dysfunction of accessory respiratory muscles with resultant hypoxia, respiratory acidosis and, if prolonged, increased oxygen consumption, metabolic acidosis, and a dramatic decrease of adenosine triphosphate (ATP). Further, with increased muscle breakdown, serum concentration of creatine phosphokinase and myoglobin may increase dramatically, leading to acute kidney failure. Marked increase in plasma catecholamines can cause a decay of skeletal muscle cells and cardiac dysfunction [88], including stress cardiomyopathy [89]. Potentially lethal complications are those of a systemic nature, especially with metabolic, respiratory, and cardiac involvement [8, 16]. Figure 7.1 displays possible acute neurologic and systemic complications and their complex, often bidirectional, and self-sustaining interactions. Because of the complexity of these interactions, it can be difficult not only to identify the complications with the strongest impact on the course and outcome of GCSE, but also to distinguish complications attributable to the underlying etiology from the direct consequences of GCSE [8].


Fig. 7.1
Acute systemic and neurologic effects of generalized convulsive status epilepticus. CNS = central nervous system; ATP = adenosine triphosphate; TNF = tumor necrosis factors
Acute Neurologic Manifestations
Acute Encephalopathy. Convulsions aside, acute brain dysfunction is a major feature of GCSE. The transient disturbance of neurotransmitters and cerebral metabolic homeostasis may lead to acute neuronal damage representing the principal pathological mechanism of SE-related acute ictal and postictal brain dysfunction (i.e., postictal encephalopathy; see Fig. 7.1). Acute neuronal damage in patients with prolonged GCSE is caused primarily by the cytotoxicity from increasing interstitial concentrations of excitatory neurotransmitters such as glutamate and aspartate [90–92]. Further, epileptogenesis and neuronal hyperexcitability may be increased by the activation and proliferation of astrocytes and microglia changing the neuronal architecture [69, 90, 93, 94]. In addition, underlying pathologic conditions, such as ischemic stroke, hypoxia, and infections may further promote the neuroglia activation [90]. Animal studies provide evidence that the neuroglia activation is accompanied by an increased release of cytokines, such as tumor necrosis factor alpha (TNF-α) and interleukins, both associated with neuronal damage [93]. Moreover, astrocytes have an important role in the maintenance of the BBB as they are closely related to the endothelial cells and their tight junctions [90]. Increased astrocyte-mediated angiogenesis may also impair the normal function of the BBB, increasing its permeability by the release of cytokines [90, 95]. This mechanism may be paralleled by the activation of glutamate receptor subtypes on the surface of endothelial cells by the excessive release of glutamate, another contributing factor to the impairment of the BBB. The BBB breakdown, together with a drop in blood pressure after an initial episode of arterial hypertension during GCSE, leads to a significant dysregulation of cerebral circulation. Decreased cerebral perfusion, which does not meet the current metabolic cerebral demands, contributes further to the disruption of the BBB [92]. There is also a growing body of evidence that neurons, neuroglia, and endothelial cells in the CNS are capable of releasing cytokines themselves and may thereby contribute further to seizure-related brain inflammation [69].
Although there is no doubt that SE-related brain injury and subsequent acute encephalopathy result at least partially in and from CNS inflammation, it remains unclear to what extent neuronal injury is attributable to the seizure itself [94, 96, 97].
Besides seizure-induced brain inflammation, systemic hypoxia during GCSE plays an important role in accelerating seizure-related brain injury (see Fig. 7.1). Animal models with pharmacologically induced SE demonstrate that systemic hypoxia is not the only cause of seizure-related ischemic brain damage but also that hypoxia is a contributing factor, together with increased and disturbed neuronal metabolism [91, 96, 98]. Evidence was provided by Meldrum and colleagues using paralyzed, artificially ventilated baboons compared with nonparalyzed baboons [99]. For the same seizure durations, the observed neuronal damage was more severe in the nonparalyzed baboons, indicating that respiratory management and reduction of muscle action in patients with prolonged seizures and GCSE might reduce neuronal damage, and hence the acute encephalopathy [91, 98].
Acute Focal Neurologic Deficits—The Task of Distinguishing Structural from Nonstructural Genesis. The patterns of brain injury associated with prolonged seizures and their underlying mechanisms have been investigated well in animal models. Neuronal necrosis occurs in several cerebral areas such as the neocortex, hippocampus, basal ganglia, and the cerebellum [98]. Similar patterns of neuronal damage, including in the neocortex, hippocampus, thalamus, and cerebellum, have been described in children and adults dying shortly after an episode of GCSE [100, 101]. In a case-control study of neuronal loss in five hippocampal regions after GCSE in humans, DeGiorgio and colleagues identified the most severe neuronal loss in GCSE cases, and less cell loss in healthy controls, and in controls matched for age, hypoxia/ischemia, previous epilepsy, and alcohol abuse, suggesting a direct impact of GCSE on neuronal cell loss in humans [102].
Distinguishing acute focal neurologic deficits that result from structural or nonstructural causes is especially challenging in the early postictal state. In many cases it remains unclear to what extent acute neurologic deficits can be attributed to the SE itself or to the underlying structural lesions. This conundrum is especially evident in animal models where the substances administered to induce SE can also cause brain lesions and deficits. In patients with transient postictal focal neurologic deficits, the distinction between a Todd’s paresis and persisting deficits from underlying structural brain lesions is challenging. Usually, postictal coma and neurologic deficits improve within minutes to hours, but recovery of neurologic function can take several days. Close clinical observation and appropriate brain imaging are essential for correct diagnosis and appropriate treatment. Finally, postictal impairment of movement by bone fractures during GCSE may mimic a Todd’s paresis [103] and should be suspected in patients with prolonged weakness without lesions on brain imaging.
Acute Systemic Manifestations
Hypoxia. Systemic hypoxia occurs frequently in SE patients and is induced primarily by the impairment of ventilation and simultaneous increased oxygen demand during prolonged or recurring seizures, with the intense muscle contractions of GCSE. Hypoxia contributes significantly to the derangement of cerebral metabolism [98] and subsequent brain injury, usually resulting from a mismatch between substrate supply and demand in cerebral areas involved in the seizure [104]. During GCSE, compensatory factors are unable to meet the considerable metabolic demand of the seizing brain [104, 105]. Vulnerable brain regions may demonstrate hypoxic neuronal injury within 30–60 min after GCSE onset [104]. In this vulnerable phase, acute failure of cerebral oxygenation inhibits the Na+–K+ pumps of the neurons, leading to the loss of cellular integrity and further release of excitotoxic glutamate (see Fig. 7.1), followed by overstimulation of N-methyl-d-aspartate (NMDA) receptors. This overstimulation further increases cellular calcium loss, mediated by second messengers, and damage to the mitochondrial respiratory chain [106]. In this stage, the severity of injury depends on the duration and degree of respiratory or circulatory dysregulation or both, body temperature, and serum glucose levels. The increased glutamate-mediated excitatory neurotransmission and simultaneously decreased GABA-mediated neuroinhibition may further promote epileptogenesis and contribute to neuronal cell death.
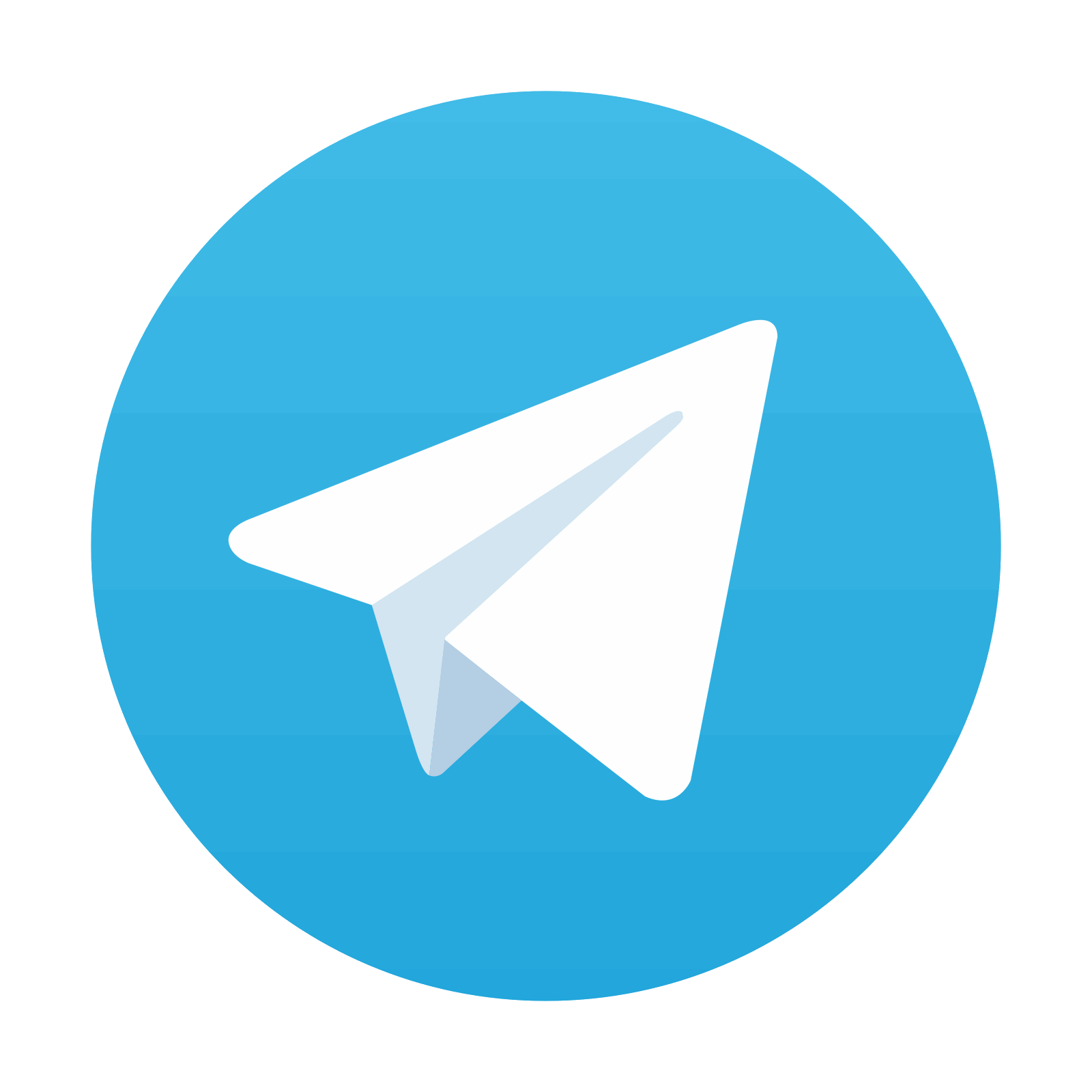
Stay updated, free articles. Join our Telegram channel

Full access? Get Clinical Tree
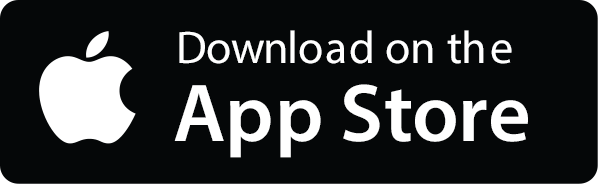
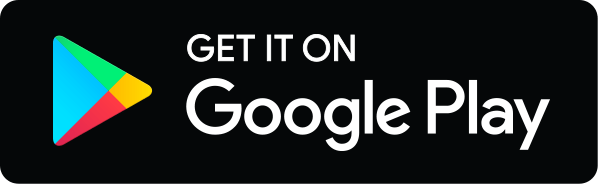