5 Eugenio Pozzati Cavernous malformation (CM) (and its synonyms cavernous angioma and cavernoma) occurs in an estimated 0.45 to 0.9% of the population and in two forms, sporadic and familial. Mutations in KRIT1, a gene located at the CCM1 locus on chromosome 7q21 that helps determine endothelial cell shape and function, are responsible for the majority of inherited cases.1,2 Until recently, CM was considered congenital in relation to a developmental but yet unsettled event disrupting local capillary-venous pattern formation between the third and eighth week of gestation1–4: The finding of intrauterine CMs confirms this origin.5 However, an increasing number of “de novo” lesions have recently been reported suggesting different mechanisms of induction.6–12 The relationships and reciprocal prevalence between congenital and acquired CMs are still obscure. Whatever the origin, the end result is a lesion consisting of closely packed sinusoids lined by endothelium and filled with slow-flowing blood, thrombotic material, and hemorrhagic cysts separated by reactive connective strands. This fibrosis of the walls of the cavernomatous vessels suggests that other cells in the subendothelial layer must be present to produce this reaction and participate in the pathophysiology of CMs.13–15 Substantial interendothelial cell gaps evidenced by electron microscopy may explain the tendency for CMs to undergo repetitive microhemorrhages, which constitute the basic, but not unique, mechanism leading to the main clinical manifestations (headache and seizures) and ignite a cascade of pathologic events responsible for the progression of the disease.15,16 If compared with the other cerebrovascular malformations (arteriovenous malformation [AVMs], telangiectasis, and venous angioma), CMs demonstrate unique dynamic peculiarities (growth and de novo occurrence) and changeable hemorrhagic propensity, which reflect their uncertain behavior suspended between a vascular malformation and a hamartoma. With telangiectasias and thrombosed AVMs, CMs traditionally belong to the old subgroup of “angiographically occult” vascular malformations characterized by an almost imperceptible and elusive flow; computed tomography (CT) scanning partially made up for this diagnostic failure, but only magnetic resonance imaging (MRI) proved to be very sensitive for detecting CMs and has greatly increased accuracy of their diagnosis. The concept of an “acquired” cavernoma arose only when the de novo appearance of this lesion was observed in patients with previously normal MRI. It is likely that MRI does not cover the full spectrum of the disease. It is possible that at an early and fugitive stage of their development, CMs do represent a discrete functional abnormality that may be “MRI occult” and may appear only later as a “de novo” lesion after initial bleeding.17 When CT scanning began to detect an increasing number of CMs at the end of the 1970s, the impression was that of a solitary, epileptogenic, and indolent lesion. The first retrospective studies indicated a bleeding rate of 0.5 to 0.7 per year.3,4 However, the increased experience gained with the introduction of MRI, the better knowledge of the familial form of the disease, the unpredictable hemorrhagic course, the better understanding of neoangiogenesis, and the de novo appearance of some lesions have greatly changed our thinking. Are we facing a lesion that for environmental, genetic, or host factors is changing its behavior? Is the familial form not only better diagnosed but also expanding? Are de novo lesions more the rule than the exception? The role of altered angiogenesis in the behavior of CMs has been recently explored: A complex disturbance involving vascular growth factors and several structural and matrix proteins (fibronectin in particular) contributes to the formation and growth of these lesions.18 Genetic factors, irradiation, and infection have all been implicated in the genesis of CMs.9 Thus, their origin is not only multifactorial but also modulated by host elements (age, sex, location) that greatly influence the hemorrhagic tendency of the malformation. Cavernomas represent primary malformative lesions made up of abnormal vessels formed in response to injury occurring both during embryogenesis and, as also demonstrated, during one’s lifetime.18,19 Besides a genetic origin, cavernomas may represent a “convergent” vascular disease consisting of a labyrinthic aggregate of endothelial-lined connective tissue channels resulting from chronic hemorrhages and recanalization events20,21 or from thrombosis of small veins and/or venous hypertension triggering the formation of a capillary proliferation.22 Compared with the spectrum of cerebrovascular malformations, the CM is a primitive entity consisting of immature proliferating vessels, and it reasonably represents inappropriate vasculogenesis without subsequent angiogenesis.23 This original and discrete endothelial structure may be targeted by an array of intra- and extravascular factors leading to the mature malformation7 as is commonly believed and imaged.24 The slow growth and hemorrhagic potential of CMs is mainly related to the interplay between passive processes (blood-flow and osmotic changes) and active factors both on the hematic component (fibrinolytic activity), the vascular wall (endothelial proliferation and angiogenesis), and the connective matrix. The growth patterns of CMs were mainly attributed to repeated intralesional hemorrhage and thrombosis, to recanalization of thrombotic material, to the expansion of hemorrhagic cyst cavities, to the deposition of hemosiderinic pigments, to calcification, and to reactive gliosis.6,7 At the same time as hemorrhage stimulates fibroblasts and connective tissue proliferation leading to “mature” CMs, fibrous “pseudopodes” and connective laces may also connect CMs to satellite telangiectasias25,26 providing further recruitment of new vascular spaces. Even though it generally represents a slow reparative process, growth by active connective tissue proliferation with reendothelialization and neovascularization may occur very rapidly.27 In this form, some CMs complete the development to true vascular hamartomas, with strong growth potential and low hemorrhagic risk. Frim et al.28 demonstrated that the fibrinolytic activity of tissue plasminogen activator in the endothelial cells of CMs may account for rebleeding and progressive growth. More recently, some immunohistochemical studies have addressed the influence of neoangiogenesis and endothelial proliferation in the development of CMs in response to hemodynamic stress, ischemia, or hemorrhage and mediated by several vascular growth factors in the lesion, the surrounding parenchyma, and serum.29–34 A proliferative activity was confirmed by the staining of endothelial cells with proliferating cell nuclear antigen (PCNA), an antibody used as a proliferation marker, in the walls of CMs.29 In cavernomas, different areas are generally observed: PCNA-positive areas, composed of thin-walled capillaries, can be opposed to PCNA-negative and less cellular thick-walled areas. Lacy walls arranged in small and dense blood cavities are believed to precede thick walls in the evolution of cavernomas.29 The concept of different compartments in the same lesion is also confirmed by the different expression of angiogenic factors in some caverns but not in others.18 Sure et al.29 have recently demonstrated the expression of the vascular endothelial growth factor (VEGF) and its receptor fck1 in CMs, suggesting angiogenic activity and potential de novo generation in these lesions. Neoangiogenesis and hemorrhage of cavernomas may be interconnected in a continuum of growth and hemorrhage. A trend toward an increased Ki67 proliferative index has been found in the endothelium of CMs with recent hemorrhage as compared with nonhemorrhagic lesions.30 Endothelial proliferation and neoangiogenesis occur not only within the malformation but may also be triggered at the periphery of the lesion. A strong expression of basic fibroblast growth factor, a potent mitogen inducing angiogenesis, was found in surrounding astrocytes and might lead to the proliferation of endothelial cells and formation of the vascular spaces typical of cavernomas.32 In extreme cases, intracranial tumors may induce erratic angiogenesis and the development of de novo vascular anomalies.33 The powerful impact of vascular growth factors in the pathophysiology of CMs was demonstrated in one of our cases with a de novo CM inside a glioblastoma in a patient affected by the multicentric disease. The complex pathologic hematic turnover and fibrous proliferation of CMs are most clearly seen in MRI, which is able to detect CMs mostly by the clotting or hemorrhage that has taken place in the malformation. Zabramski et al.35 divided CMs into four types, which probably also represent different and changeable stages of evolution and clinical significance in an ordered progression of hematic changes.35 De novo lesions offer the opportunity to better study the kinetics of these lesions and a possible clinico-radiologic correlation, both in the familial and nonfamilial (in particular postirradiation) subgroups, which differ in their evolution. MRI of a de novo CM is varied and reflects the pathogenesis of the lesion. For example, type I lesions are typical of postirradiation CMs, whereas type III lesions are more common in the familial form of the disease. In general, if type I and II correspond with dynamic expressions of the disease, type III signal characteristics might represent chronic lesions that are no longer hemorrhaging.35 Recently, increased VEGF expression has been found in a type III lesion, suggesting that the CM may also be dynamic at this stage.36 It is unclear if the changes from type I to III correspond with passive turnover of the hemorrhagic and thrombotic material or to remodeling with the regression of supernumerary vessels and the intervention of apoptotic factors in the biological behavior of this lesion.37 Type IV lesions represent the basic entity of the known pathologic spectrum of CMs and possess the potential of leading to the other types of lesions. Thus, they may represent capillary telangiectasias, true minute CMs, or a basic “hemorrhagic capillary malformation.”38,39 They appear in MR images as punctate hypointensities due to the susceptibility of microscopic deposits of hemosiderin and the original hemorrhage. MRI studies must include gradient-recalled echo (GRE) sequences documenting the absence of nascent lesions en route to a full-blown CM. Contrast enhancement may further help differentiate telangiectasia from CM.39 Dynamic signal changes in serial follow-up MR images have demonstrated that type IV lesions are rather stable and only rarely complicated by overt bleeding.38 This supports the theory that de novo lesions generally result from an abrupt hemorrhagic event in a differing pathogenetic context. A precursor or nascent CM that may evolve into a mature lesion is often alluded to. This undefined structure has never been imaged, probably because it represents a functional phase of the malformation, and an intact CM may initially demonstrate extremely subtle signal changes not detected by MRI.17 This hypothesis has been confirmed in patients with seizures and normal MRI who later develop a CM40 supporting the existence of a “cryptic” cavernomatous state, a sort of hypothetic and fugitive “type V” lesion. We may have observed one of these cases, characterized by appearance and involution of a de novo thalamo-mesencephalic CM in a woman with multiple bleeding lesions (Fig. 5-1). In a retrospective evaluation of MRIs, a faint, almost imperceptible, roundish hypointensity preceded (by a few days) the hemorrhagic onset and the late growth of the malformation, followed by its complete regression over 6 months. It is difficult to say if this course represented a vascular engorgement preceding the hemorrhagic onset or some endothelial build-up of the malformation. In keeping with this evolution, CMs may subsequently arise at the site of resolved intracerebral hematomas,41 emphasizing their deceptive autodestruction and possible late formation. Figure 5-1 (A) Axial MR image of a 36-year-old woman with recurrent seizures secondary to a hemorrhagic CM of the right motor strip (not shown). The right thalamic region was considered initially normal, but further retrospective consideration disclosed a faint marginated hypointensity in the thalamo-mesencephalic region (arrow) at the site of the subsequent hemorrhage. (B) CT scan 15 days later, performed after sudden diplopia and left arm dysmetria, showing a subthalamic hemorrhage. (C, D) Axial MRI 1 month later showing the growth and hemorrhage of a thalamo-mesencephalic CM. The patient was managed conservatively: MRI at 1 year showed chronic hemorrhage with hemosiderin staining at the affected sites (not shown). All these factors are the necessary foundation for the in-depth discussion of de novo CMs. We have divided de novo CMs into two groups on the basis of their differing pathogenesis: familial and nonfamilial (postirradiation, hemodynamic, “convergent,” and cryptogenetic). The familial form is treated elsewhere in this book, and it will be considered here only for its relevance to de novo formation of CMs. Its prevalence is increasing and may account for 30 to 50% of all CMs.35 Zabramski et al.35 were the first to document the de novo appearance of new lesions in the familial form at a rate of 0.36 new lesions per patient per year in families of mostly Hispanic-American origin. Many of these new lesions were classified as type III and were asymptomatic. Type I lesions were found in 25% of the patients and were clinically silent. The incidence of symptomatic hemorrhage was 1.1% per lesion per year and 6.5% per patient per year.35 Labauge et al. in their French series found a similar de novo formation rate of 0.4 new lesions per patient per year,42 confirming a similar clinical trend in a different ethnic group. Conversely, MRI of de novo nonfamilial (postirradiation in particular) CMs is often represented by a type I appearance indicating a prevalent hemorrhagic onset of the malformation with neurologic disturbances occurring in about half of the cases.7,8,12 At this stage, the “mural” angiomatous nodule is minimal, and the main bulk of the lesion is constituted by blood. An escalation of hemorrhagic risk is found when sporadic, familial, and acquired CMs are compared. In one series of prospective MRI of CMs, type I characteristics occurred in only 6% of the lesions on initial examination.43 In a series of familial CMs, type I CMs occurred in 16% of the cases,35 and de novo lesions in the familial form had the type I pattern in 26% of the cases.44 In our patients with de novo nonfamilial CMs, type I lesions occurred in around 70% of the cases, suggesting that a type I CM is highly indicative of the hemorrhagic onset of a de novo lesion. However, in some cases de novo CMs appear as a true growth of a compact tissue made up of a tangle of fibroendothelial spaces without apparent hemorrhage, the so-called cavernomatous matrix,25 characterized by a peculiar “honeycomb” appearance without a dark ring on MR images and definitely supporting the intervention of angiogenic factors. An open question in the familial form is the impact of “anticipation,” which refers to the decrease of age at symptom onset in an inherited disease, increased severity in subsequent generations, or both.45 The International Familial Cavernous Angioma Study (IFCAS) group found anticipation in age at symptom onset but not in the severity of the disease and suggested that, although one gene determines the occurrence of CMs, a second gene could affect the growth and size of angiomas, and, therefore, the onset of clinical manifestations. It is unclear if anticipation corresponds with a de novo occurrence or to an early clinical onset of a preexistent lesion. The observation of an early and aggressive onset in childhood may contrast with minor symptoms in relatives who are carriers of the mutation and should warn of the possible intrusion of an environmental factor and of a “modifying” gene.1,45,46 Some authors have also noted an unusual increase in the number of de novo lesions after the age of 50, suggesting a modification of the epidemiology47 and clinical activity in older patients. We have found a de novo type III lesion and a hemorrhagic CM in an 86-year-old patient with multiple CMs, confirming the continuing progression of the disease throughout one’s lifetime. Multiplicity, which is a landmark of the familial disease, occurs not only in the inherited form. Multiple and de novo lesions have also been described in patients with apparently sporadic CMs.46,48 Although low penetrance in some families cannot be excluded, de novo mutations in the KRIT1 gene have been demonstrated in patients with multiple and nonhereditary CMs.48 The patient described by Lucas et al.46 had an inheritable condition, representing a “founder” for a new lineage of individuals with familial CMs. This subgroup of multiple nonfamilial CMs is again poorly defined in terms of natural history and epidemiology. The rate of de novo lesions may be higher than that in familial cases. In eight patients with multiple and de novo nonfamilial CMs we have recently studied, new lesions were detected at a mean interval of 1.6 years and were sometime characterized by simultaneous activity (bleeding and growth) at multiple sites in a “crescendo” of hemorrhages, which we have called cavernomatous crisis.49,50 This finding may suggest that multiple nonfamilial CMs tend to occur synchronously, whereas the familial form is characterized by a more gradual progression. A case of multiple sporadic CMs showing aggressive behavior has recently been reported. In this case, endothelial proliferation induced by a spike of serum VEGF has been suggested as a possible mediating factor36 and disease progression was positively altered by the administration of glucocorticoids. Surgery is problematic in patients with multiple symptomatic CMs, and the suppressive effects of glucocorticoids on serum VEGF levels may provide a useful strategy in the management of CMs. The second group of de novo CMs represents an etiologic spectrum and encompasses lesions that appear in patients without familiarity and are mainly attributable to host and external agents (irradiation, infection, iatrogenic seeding, and hemodynamic factors).7–11,51,52 De novo formation of CMs without a family history, previous cranial radiation, or other apparent reason has also been reported (cryptogenetic CMs).7,9,40 Overall, we have treated 25 patients with de novo nonfamilial CMs. Most of them are postirradiation, and a minority encompass hemodynamic and cryptogenetic malformations, including lesions that appeared during and after pregnancy and were hypothetically attributed to hormonal factors.7 It has recently been demonstrated that therapeutic irradiation of the central nervous system (craniospinal, whole brain, local field, and focused), with its effects on the cerebral vasculature (endothelial injury and proliferation, fibrinoid necrosis, and capillary telangiectasia induction) plays a role in the delayed genesis of a vascular entity mimicking a CM, unaccompanied by other manifestations of radiation damage.6,8,10–12,53–58 Telangiectasia has previously been described as a result of radiation to the brain at the site of the transition between radiation necrosis and the normal parenchyma. In contrast, this acquired vascular entity occurs in an otherwise normal brain, more often near the gray-white junction.11 Probably, this lesion is not a typical CM but instead a pathologic variant deranged by irradiation.7 Other terminologies have been used (telangiectasia, occult cerebrovascular malformation, hemorrhagic vasculopathy)8,11,56 to describe the same entity, which likely represents a spectrum of postirradiation vascular changes and proliferations with the same radiologic and pathologic characteristics. The most significant confirmation is represented by one case of de novo lesion in a patient with multiple nonfamilial CMs who was irradiated using a Gamma Knife for an expanding CM of the basal ganglia and later operated on, indicating the powerful effect of the irradiation over a hidden cavernomatous focus. The new lesion had some peculiar characteristics: pluri-hemorrhagic presentation, cystic expansion with fluid level, and a lacy pathologic configuration.8 Since the first descriptions at the end of the 1980s,6,10 several cases have been reported, and now postirradiation CMs constitute the best-known group of de novo nonfamilial lesions. Such occurrences are very rare, and the precise prevalence is poorly understood. Some centers having a large referral have not reported de novo lesions,59 whereas an accrual of cases occurs elsewhere, without apparent reason.8,12 In one retrospective study, children with a medulloblastoma who received whole-brain radiation therapy had a 4.8% incidence rate of CM development occurring on the average 5.5 years after irradiation.53 Probably, the true prevalence of postirradiation CMs is similar to this figure in childhood, but it lowers to ~1% when adults are included11 due to the greater deleterious effect of the radiation on the developing nervous system and its vasculature.12 A release of VEGF promoted by irradiation has been found to be expressed mostly in children and may result in the induction of angiogenesis.58 Postirradiation CMs have occurred in patients treated for a great variety of neoplasms, particularly in childhood. Craniospinal and whole-brain irradiation for acute lymphocytic leukemia (ALL) plays a preponderant role in induction of CMs in infancy (Fig. 5-2), and the extensive irradiation certainly amplifies the basic damage responsible for the disease. In the patients who underwent whole-brain radiotherapy, CMs seem to occur more frequently in the temporal lobe.57 The majority of postirradiation CMs seem to appear within 5 years of the treatment and particularly in children irradiated before 10 years of age.58 Multiple CMs are more likely to occur in patients who were irradiated at a younger age53 and may have a different latency in the same individual, with new lesions occurring at different times even several years apart: careful MRI follow-up is necessary in these cases, particularly in children receiving whole-brain irradiation. It is believed that no significant increase in the frequency of CMs is associated with chemotherapy55; only one report refers to the possible occurrence of a CM associated with chemotherapy.60 The pathogenesis of postirradiation CMs is still debated and may range between direct induction and formation from a preexistent lesion8,11,12 considering that CM and telangiectasia may represent a spectrum within a single pathologic entity. Gaensler et al.11 suggested that radiation of the brain may induce a telangiectasia and varying amounts of hemorrhage. These thin-walled vessels appeared to be ectatic venules and capillaries, which may represent collateralization of venous drainage from areas of postirradiation congestion or occlusion.24 Radiation may preferentially affect the endothelium of veins, producing a veno-occlusive disease with subsequent development of venous hypertension and promotion of ischemia, microhemorrhages, and angiogenic factor production.61 These changes may compact and originate a vascular entity with the overall effect of mimicking a cavernoma. The report of a postirradiation CM formed at the site of a venous angioma confirms this mechanism.62 Otherwise, Larson et al.12 have suggested that radiation may trigger a proliferative endothelial pathway, causing a preexistent capillary telangiectasia to evolve into a CM. Alternatively, irradiation may trigger a genetic or other type of latent predisposition. CMs can arise as a result of radiation-induced mutation in the KRIT1 pathway, a “second hit” in an area of genetically predisposed vascular tissue.44,55 We were surprised that none of the cases of postirradiation CMs manifested any family history of the lesion also at sites with high familial occurrence of the disease,9 as if a different type of host predisposition and genetic aberration might be operative in these patients. Some relationship with latent hereditary hemorrhagic telangiectasia (HHT) has been suggested59 considering that brain irradiation may induce an erratic proliferative vasculopathy and interfere with the basic genetic mechanism of this condition. It is intriguing that both HHT and cerebral CM are characterized by disruption of normal vascular morphogenesis.63 Interestingly, in children, radiation therapy for acute lymphocytic leukemia constitutes a large percentage of postirradiation CMs, as if neoplasms and vascular malformations might represent two different manifestations of a same disease characterized by a high sensitivity to irradiation and a predisposition to malignancies, as occurs in ataxia-telangiectasia or Louis-Bar syndrome. Postirradiation CMs initially reflect a prevalent endothelial structure lacking the typical aging changes and often assume an en dentelles configuration characterized by closely packed sinusoids with scarce fibrous tissue8,26 associated with telangiectatic foci and a thrombosed venous drainage.8 Some inhibition of fibrous proliferation may characterize postirradiation CMs and differentiate the evolution of this subgroup from its spontaneous counterpart. Clinical features of postirradiation CMs are related to the onset of a moderate cerebral hemorrhage and include epilepsy, headache, and vomiting: Focal signs occur more often in patients with brain-stem lesions. Massive intracerebral bleeding is rare. A discrete percentage of patients (four in our series, or 30% of cases) remain asymptomatic during the course of the disease, but it is possible that this percentage may even be higher.57 An increased risk of hemorrhage is generally reported in radiation-induced CMs compared with spontaneous lesions, and “opening” intracerebral bleeding appears to be associated with postirradiation CMs in 25 to 50% of cases8,11,12 with a risk of hemorrhage varying from 3.6 to 6.7% per year, as calculated in one series.12,55 Our experience in 13 patients is less severe, with an overall risk of bleeding of 1% per year, which increases to 1.4% per year in children. We have also found hemorrhagic accrual during the first 2 years in three cases with recurrent bleeding. These findings may probably also be extended to the overall subgroup of de novo CMs and reinforce the recent observation of clustering hemorrhages in the first years after presentation.64 This bleeding pattern adapts well to a relative clinical instability of the lesion after its formation and to the progressive quiescence related to the later acquisition of a stable histologic structure. It is interesting to document other histopathologic differences between congenital and postirradiation CMs. Challa et al.65 believe that the presence of small amounts of neural parenchyma may help differentiate postirradiation from true cavernous angiomas. The intralesional parenchyma probably reveals a relatively recent formation of the lesion, well before substitution of intervening neural tissue by progressive reactive fibrosis and gliosis. This conformation may increase the interfacing brain-cavernoma and the possible interaction with vascular growth factors expressed in the adjacent brain32 with the recruitment of new endothelial vessels. This microenvironment may represent a structural distinction in the central nervous tissue that may predispose to different lesion activity as suggested by Porter et al.66 Irradiation may promote cyst formation with a fluid level in relation to permeability-inducing factors, osmotic properties, and alterations of the blood-brain barrier in the nascent malformation.8,16,56 Sedimentation of red blood cells may represent the equivalent of a rebleeding in a resolving hematoma, similar to the hemorrhagic events in chronic subdural hematomas and possible expression of an altered fibrinolytic system resulting from irradiation.53
Cavernous Malformations as Dynamic Lesions: De Novo Formation, Radiologic Changes, and Radiation-Induced Forms
De Novo Familial Cerebral Cavernous Malformations
De Novo Nonfamilial Cerebral Cavernous Malformations
Radiation-Induced Cerebral Cavernous Malformations
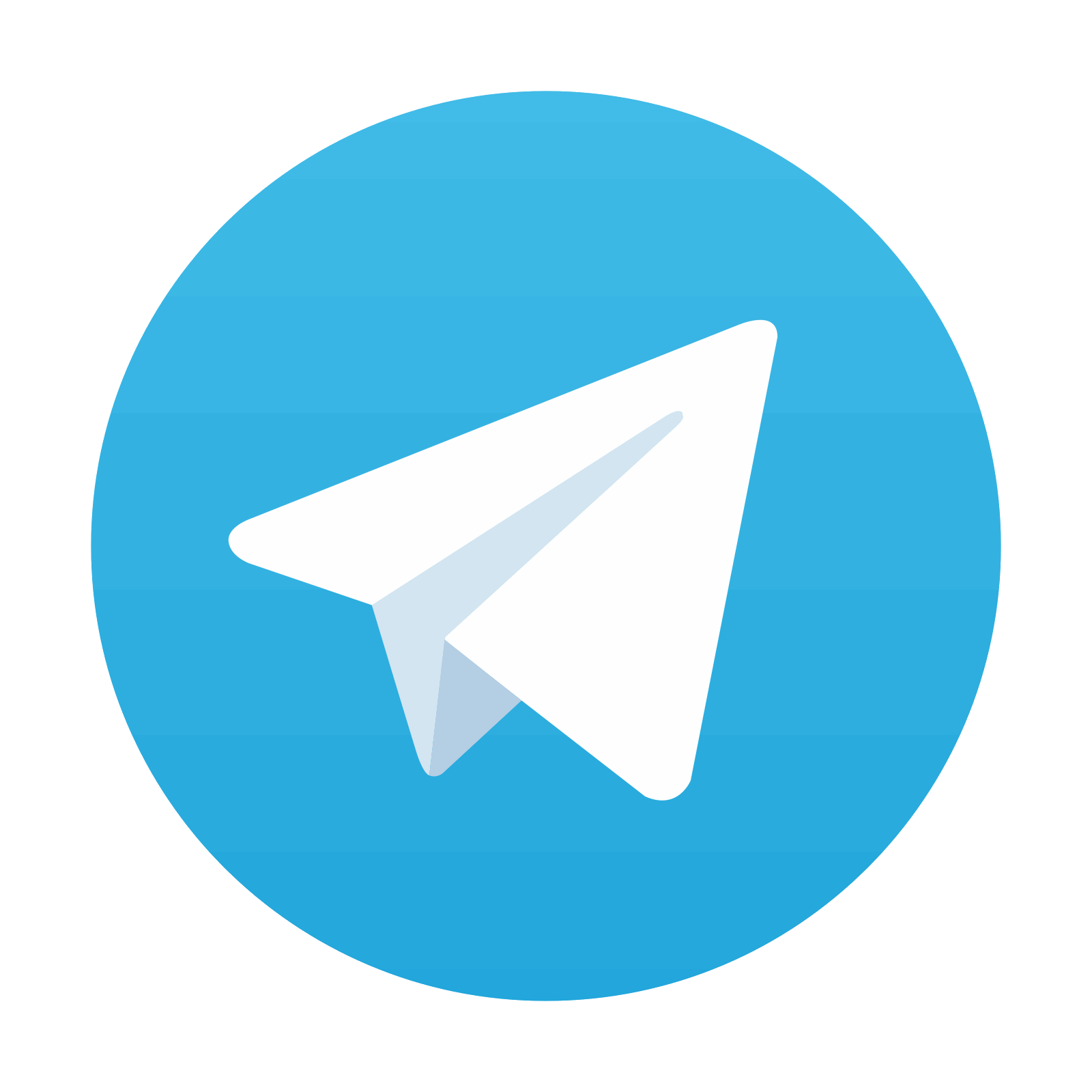