Fig. 3.1
The neuron
Neuronal Membrane
Like all mammalian cell membranes, the neuronal membrane is constructed of a phospholipid bilayer conferring upon the membrane the property of selective permeability (Fig. 3.2). Ions, charged atomic particles, are by nature hydrophilic and thus unable to freely cross the cell membrane. This sets up a gradient across the neuronal membrane resulting in a separation of ions according to concentration and electrical charge. This is called an electrochemical gradient. Separation of ions is synonymous with separation of charge and is the basis of the neuronal membrane potential. Neurons and muscle cells are able to move charge (ions) across the membrane and are thus termed electrically excitable cells. This movement of ions is accomplished through transmembrane proteins that serve as ion channels. We will discuss the role of ion channels in maintaining the resting membrane potential as well as generation of the action potential.
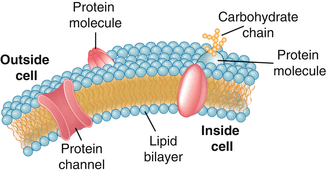
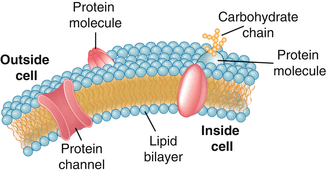
Fig. 3.2
Phospholipid bilayer. The neuronal cell membrane is composed of a phospholipid bilayer studded with transmembrane protein molecules. Among the varied functions of the transmembrane proteins is transporting ions across the cell membrane, an activity necessary for electrical excitability
Ion Channels
Ion channels are transmembrane proteins which provide a hydrophilic pore through which ions may pass between the cytoplasmic and extracellular sides of the membrane. Ion channels may be selective for either cations or anions or even for a specific ion. Ion channels are opened and closed in response to some event such as a change in membrane potential or the binding of a neurotransmitter.
Voltage-gated ion channels open and close in response to changes in the membrane potential and are termed voltage-gated ion channels. These ion channels are involved in propagation of the action potential. Each voltage-gated ion channel has a different activation range, defined as the range of voltages at which the channel remains open. The change in membrane potential that accompanies the opening of voltage-gated ion channels ironically results in their closure and opening of other voltage-gated channels. Such an interplay occurs between voltage-gated sodium and potassium channels during the propagation of the action potential.
Ion channels that are gated by the binding of a molecule (ligand) are termed ligand-gated ion channels. Neurotransmitter receptors are a type of ligand-gated ion channel. The binding of a neurotransmitter to its receptor is responsible for the initiation of the action potential or other postsynaptic signaling events. Signaling between neurons is accomplished through neurotransmitter receptors. When a neurotransmitter binds its receptor, it induces a conformational change (opening) in the receptor protein allowing the passage of ions across the membrane. The result is a change in membrane potential that can lead to an action potential. We’ll discuss this in more detail below.
Excitatory neurotransmitters such as glutamate or acetylcholine bind to and open nonselective cation channels that allow Na+, K+, or Ca2+ to move across the membrane. Inhibitory neurotransmitters such as GABA and glycine bind to and open chloride channels.
Glutamate is the main excitatory neurotransmitter of the central nervous system. Acetylcholine (ACh), while found in the brain, is also the neurotransmitter of the neuromuscular junction. GABA is an inhibitory neurotransmitter found mainly in the brain, while glycine is the main inhibitory neurotransmitter of the spinal cord.
The Membrane Potential
The separation of ions across the membrane results in a recordable (nonzero) membrane voltage (Fig. 3.3). This resting membrane potential is approximately 60–70 mV in a typical neuron. By convention we indicate the polarity of the potential with respect to the charge on the inside of the cell. The intracellular space bears a negative charge with respect to the extracellular space; therefore, we say that the usual resting membrane potential of a neuron is approximately −70 mV.
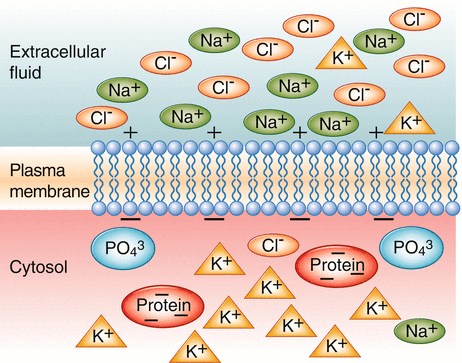
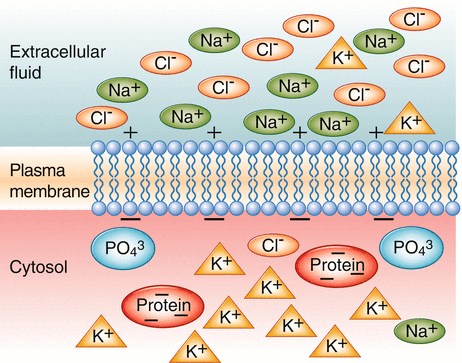
Fig. 3.3
Membrane potential. The separation of ions across the neuronal membrane is the basis of the membrane potential. Sodium is more abundant extracellularly and potassium is in greater concentration on the inside of the cell. Potassium leak channels and the sodium–potassium pump create a local negative charge along the intracellular membrane
The term “potential” in membrane potential indicates that there exists the drive for ions to move across the membrane. Remember that these ions are separated by the selectively permeable membrane and are unable to move freely. Ions want to move down their electrochemical gradient. If we were to allow ions to move freely across the membrane, the membrane potential would reach a value at which the ion was at equilibrium and there would be no net movement across the membrane. In other words we would say the ion would be “happy.” The membrane potential at which this would occur is called the equilibrium potential, and each ion has its own equilibrium potential. The potassium equilibrium potential is approximately −85 mV, while the sodium equilibrium potential is nearly +35 mV. Remember that the typical resting membrane potential is −70 mV. This means that potassium is much “happier” than sodium at rest; however, if allowed to move, it would still try and change the membrane potential to −85 mV.
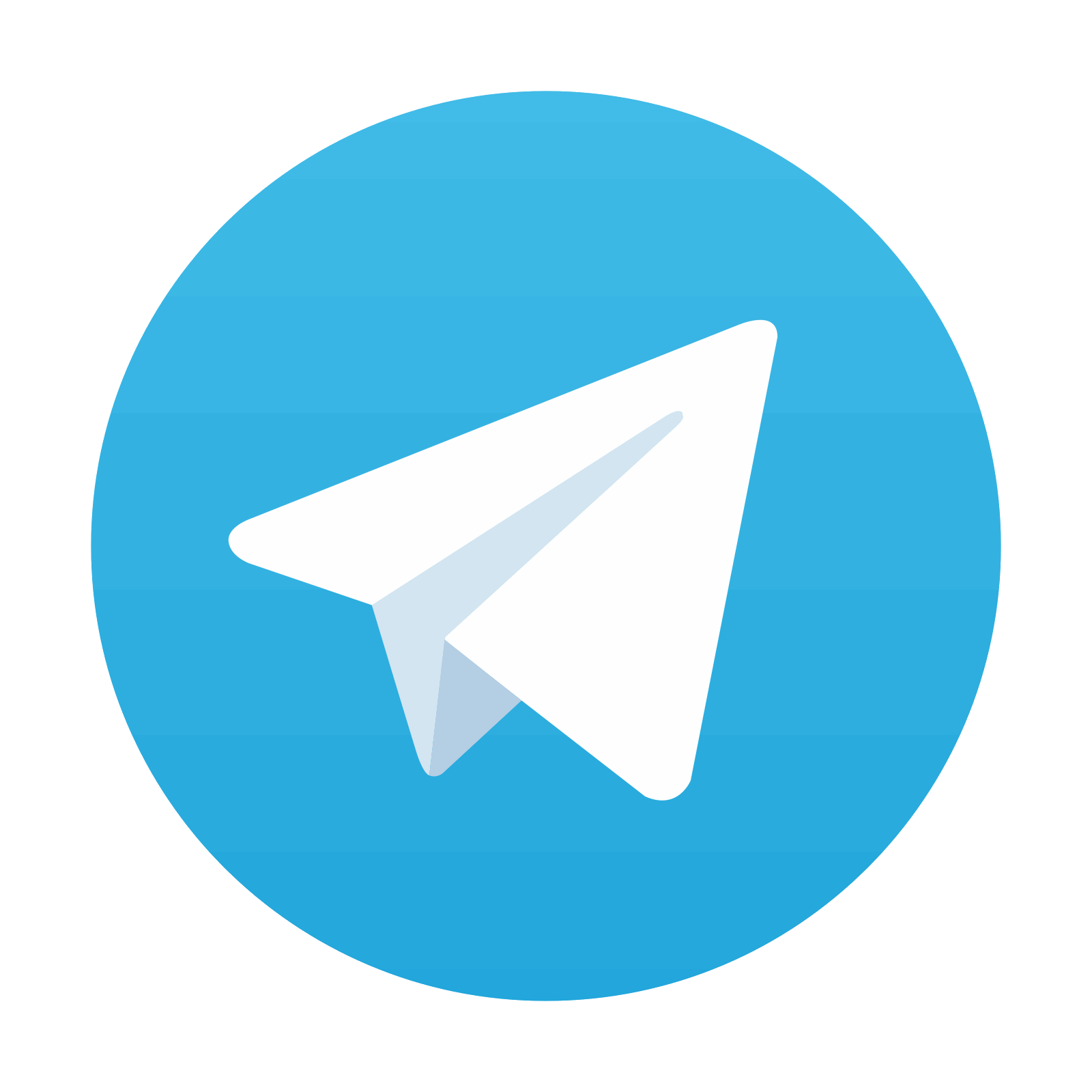
Stay updated, free articles. Join our Telegram channel

Full access? Get Clinical Tree
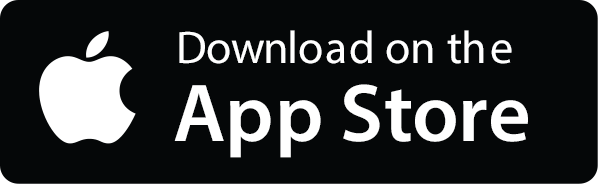
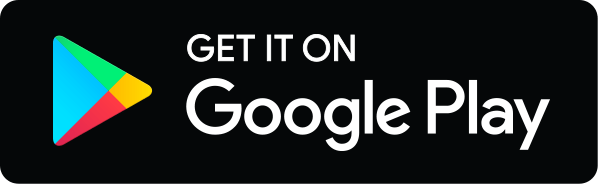