Study guidelines
- 1.
Describe the functional organisation of the cerebellum and the relationship to the deep cerebellar nuclei.
- 2.
Classify the microscopic organisation of the cerebellar cortex and the cell types found in each layer.
- 3.
Contrast the two types of afferents to the cerebellar cortex with respect to origin, termination, and neurotransmitter.
- 4.
Characterise the origin of output from the cerebellum, the neurotransmitter released, and the effect on the deep cerebellar nuclei.
- 5.
List the cerebellar efferent pathways from the deep cerebellar nuclei to their eventual point of ‘termination’.
- 6.
Provide examples of the expected clinical manifestations of dysfunction of the vestibulocerebellum, spinocerebellum, and pontocerebellum.
- 7.
Be able to define posturography and what insights it provides with respect to cerebellar function.
Phylogenetically, the initial development of the cerebellum (in fish) took place in relation to the vestibular labyrinth. With the development of quadrupedal locomotion, the anterior lobes of the cerebellum (in particular) became richly connected to the spinal cord. Assumption of the erect posture and achievement of a whole new range of physical skills have been accompanied by the appearance of massive linkages between the posterior lobes of the cerebellum and the cerebral cortex. In general, cerebellar connections with the vestibular system, spinal cord, and cerebral cortex are arranged such that each cerebellar hemisphere is primarily concerned with the coordination of movements ipsilaterally.
The gross anatomy of the cerebellum is described briefly in Chapter 3 , where it may be reviewed at this time.
Functional anatomy
Phylogenetic and functional aspects can be combined (to an approximation) by dividing the cerebellum into functional regions ( Figure 25.1 ). The flocculonodular lobe is also known as the vestibulocerebellum because it receives input and directs its output to the vestibular nuclei; it controls eye movements through the vestibular system. (Other contributions from the brainstem and through the pons from the parietal and occipital cortex provide further input and also contribute to eye movement control.) Portions of the vermis (dorsal) receive input from the reticular formation, frontal eye fields, and superior colliculus and then project to the deep cerebellar nuclei (the fastigial nuclei ) in the white matter close to the nodule of the cerebellum ( Figure 25.2 ). The fastigial nuclei project to the gaze centres of the brainstem and vestibular nuclei to control saccadic eye movements ( Chapter 24 ).


A paramedian strip, the spinocerebellum , includes the vermis and paravermal (next to the vermis) cerebellar cortex and receives input from the spinocerebellar tracts (and through the pons cortical input, as well as input from the vestibular nuclei and reticular formation). The vermis projects to the fastigial nuclei and, through projections to the reticular formation and vestibular nuclei via the reticulospinal and vestibulospinal tracts , influences postural reflexes of the head and trunk. The paravermal area receives cortical input via the pons and spinal cord via the spinocerebellar tracts and projects to the globose and emboliform nuclei ( Figure 25.2 ). The two nuclei together are called the interposed nucleus . The interposed nucleus projects to the red nucleus and thalamus that, through their connections to the spinal cord ( rubrospinal tract ) and cortex, monitor and correct motor activity of the limbs.
The remaining lateral strip is the largest and projects through the dentate nucleus ( Figure 25.2 ). This area is called the pontocerebellum because of its numerous inputs from the contralateral nuclei pontis. It is also called the neocerebellum because the pontine nuclei convey information from large areas of the cerebral neocortex (phylogenetically the most recent). It is uniquely large in the human brain and plays a significant role in the planning, initiation, control, and correction of voluntary movements.
Microscopic anatomy
The structure of the cerebellar cortex appears uniform throughout. It is made up of three principal layers: the innermost granular layer (directly adjacent to the white matter), the Purkinje cell layer composed primarily of Purkinje cells, and the outermost molecular layer ( Figure 25.3 ).

The granular layer contains billions of granule cells , whose somas are only 6 to 8 μm in diameter. Their short dendrites receive so-called mossy fibres from all sources except the inferior olivary nucleus. Before reaching the cerebellar cortex, the mossy fibres, which are excitatory in nature, give off collateral branches to the deep cerebellar nuclei.
The axons of the granule cells extend into the molecular layer where they divide in a T-shaped manner to form parallel fibres (they run parallel to the transverse fissures of the cerebellum), parallel to other parallel fibres, but perpendicular to the axes of the Purkinje cell dendritic tree. They make excitatory (glutamatergic) contacts with the distal dendrites of Purkinje cells. The granular layer also contains Golgi cells (see later) whose dendrites are stimulated by the parallel fibres of the granule cells.
The Purkinje cell layer consists of very large Purkinje cells . The fan-shaped dendritic trees of the Purkinje cells are the largest dendritic trees in the entire nervous system. The ‘fans’ are disposed at right angles to the parallel fibres.
The dendritic tree of Purkinje cells receives synapses from a huge number of parallel fibre axons (as many as 100,000, but only a small number are active at any one time) of granule cells, each one making successive synapses (one or two per Purkinje cell) on the dendritic spines of about 400 (possibly many more) Purkinje cells. Not surprisingly, stimulation of only a small number of granule cells by mossy fibres has a small facilitatory effect upon many Purkinje cells. Many thousands of parallel fibres must act simultaneously to bring the membrane potential of the Purkinje cell to threshold.
Each Purkinje neuron also receives a single climbing fibre from the contralateral inferior olivary nucleus. In stark contrast to the one-per-cell synapses of parallel fibres, the olivocerebellar fibre divides at the Purkinje dendritic branch points and makes numerous synaptic contacts (thousands) with those dendritic spines ( Figure 25.4 ). A single action potential applied to one climbing fibre is sufficient to elicit a short burst of action potentials from its Purkinje cell, known as a complex spike (see later). The complex spike is so powerful that, for some time after it ceases firing, the synaptic effectiveness of these parallel fibres undergoes a long-term depression (LTD) . In this sense, the Purkinje cells remember that they have been excited by olivocerebellar fibres.

The axons of the Purkinje cells are the only axons to emerge from the cerebellar cortex. Remarkably, they are entirely inhibitory (the neurotransmitter is GABA) in their effects. Their principal targets are their corresponding deep cerebellar nuclei. They also give off collateral branches, mainly to Golgi cells .
The molecular layer is almost entirely taken up with Purkinje dendrites, parallel fibres, supporting neuroglial cells, and blood vessels. However, two sets of inhibitory interneurons are also found there, lying in the same plane as the Purkinje cell dendritic trees, but perpendicular to the granule cell axons (parallel fibres). Near the cortical surface are small stellate cells ; close to the Purkinje cell layer are larger basket cells . Both sets are contacted by parallel fibres, and they both inhibit Purkinje cells laterally. The stellate cells synapse upon dendritic shafts, whereas the basket cells form a ‘basket’ of synaptic contacts around the soma, as well as forming axo-axonic synapses upon the initial segment of the axon. A single basket cell synapses upon some 250 Purkinje cells. Because one group, or row, of Purkinje cells is active, the rows on either side will be inhibited by these interneurons.
The final cell type in the cortex is the Golgi cell , whose dendrites are contacted by parallel fibres and whose axons divide extensively before synapsing upon the short dendrites of granule cells. The synaptic ensemble that includes a mossy fibre terminal, granule cell dendrites, and Golgi cell boutons is known as a glomerulus ( Figures 25.5 ). Golgi cells function to limit the output of the mossy fibre inputs onto the granule cells.

Spatial effects of mossy fibre activity ( Figure 25.6 )
As already noted, cerebellar afferents (except for olivocerebellar ones) form mossy fibre terminals after giving off excitatory collaterals to one of the deep cerebellar nuclei. The afferents excite groups of granule cells, which in turn facilitate many hundreds of Purkinje cells arranged in rows beneath the parallel fibres of the granule cells. Along the row of excitation, known as a microzone (smallest efferent unit of the cerebellar cortex), the Purkinje cells begin to fire and to inhibit neurons in one of the deep nuclei. At the same time, weakly facilitated Purkinje cells along the edges of the microzone are inhibited by stellate and basket cells. As a result, a particular row of Purkinje cells is sharply focused, while those rows on either side will be inhibited. The excitation is terminated by Golgi cell inhibition via the granule cells that stimulated them (self-limiting). Powerful excitation will last longer because highly active Purkinje cells inhibit underlying Golgi cells, thereby allowing the granule cells to continue to fire.

- 1.
Mossy fibre stimulating a granule cell (Gr).
- 2.
Parallel fibre activity follows simultaneous activation of many granule cells.
- 3.
Activation of distal Purkinje cells (P1) results in selective inhibition of neurons within the appropriate central cerebellar nucleus.
- 4.
Activation of stellate (S) and basket cells (B) inhibits adjacent Purkinje cells (P2).
- 5.
Golgi cells (Go) terminate granule cell activity.
- 6.
Intense online activity can be sustained by inhibition of Golgi cells by Purkinje cells.
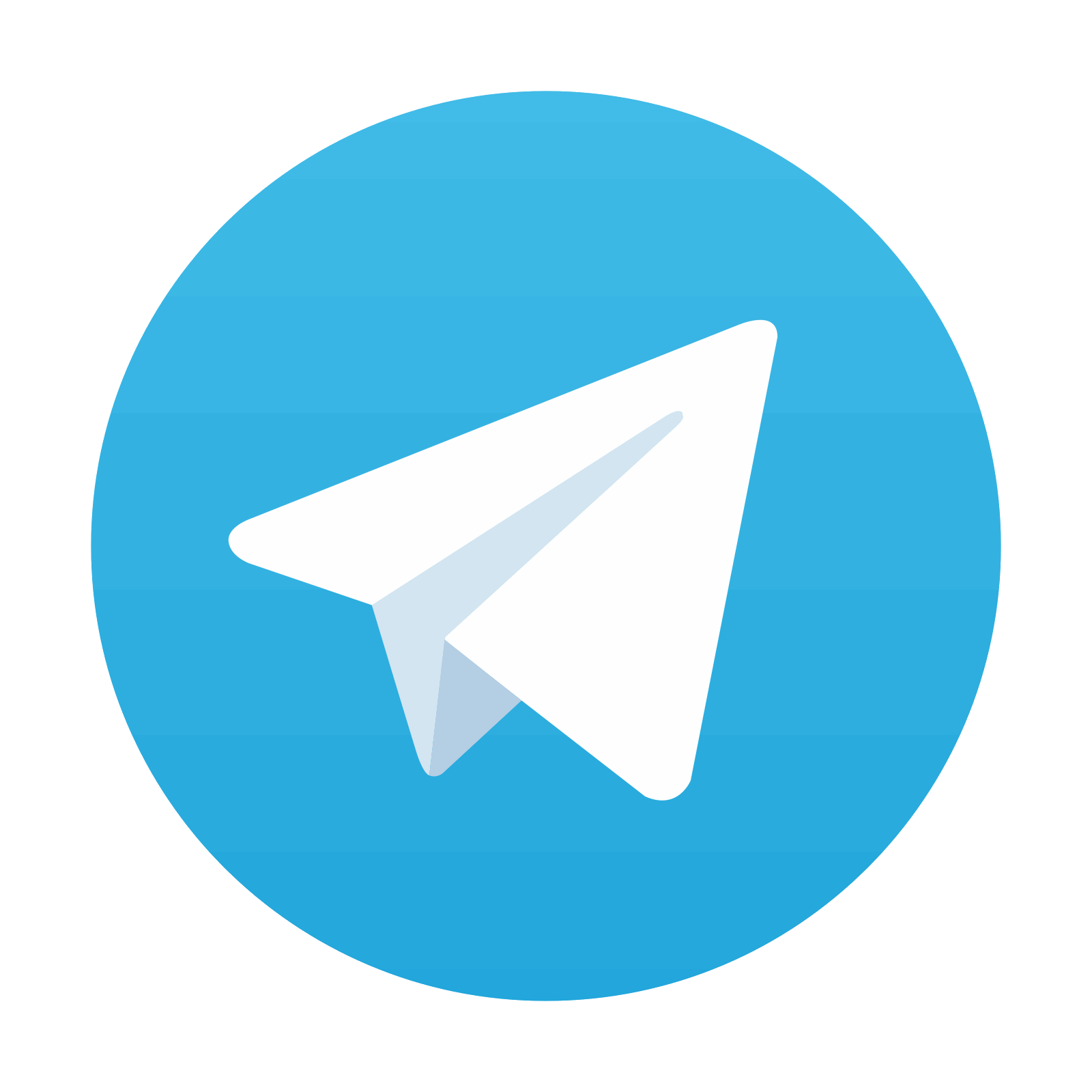
Stay updated, free articles. Join our Telegram channel

Full access? Get Clinical Tree
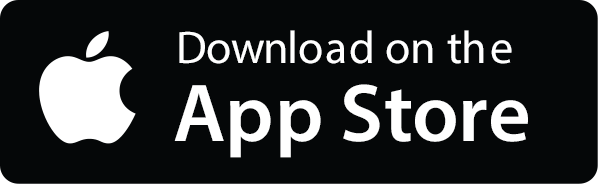
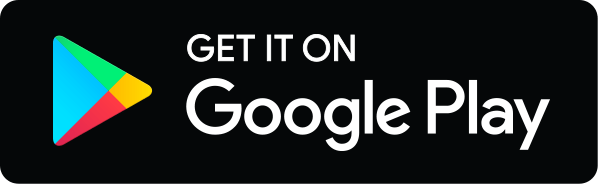
