Cerebral Vasospasm
Key Points
Cerebral vasospasm following subarachnoid hemorrhage is a poorly understood phenomenon with an unclear correlation with the delayed ischemic neurologic deficits (DINDs) seen in a subgroup of patients with this disorder.
Mechanisms other than simple regional hypoperfusion and ischemic injury may be at play in declining patients exhibiting angiographic evidence of cerebral vasospasm.
Introduction
Patients who are severely ill with the sequelae of aneurysmal subarachnoid hemorrhage encounter significant setbacks even after their aneurysm has been secured by open clipping or endovascular occlusion. Chief amongst these is the onset of delayed ischemic neurologic deficits (DINDs) related to stroke or stroke-like lesions throughout the brain, usually seen within the first 2 weeks after the bleed. This overlaps with the long recognized period of risk of vasospasm following subarachnoid hemorrhage, and for many years conventional wisdom has held that the two phenomena are directly and causally related (1,2), that is, that ischemia of the brain during this period of critical illness is related to regional cerebral perfusion deficits induced by arterial spasm. Recent work has drawn this direct 1:1 relationship into question (3,4). It is likely that angiographic evidence of vasospasm or altered indices on transcranial Doppler imaging are just one facet of a complex physiologic storm at play in these severely ill patients (5). Still, vasospasm of the major branches of the circle of Willis and of the distal arterioles likely makes a substantial contribution to the onset of ischemia in some patients and endovascular relief of severe vasospasm is likely to continue to play a role in their management.
Vasospasm and Cerebral Ischemia
Delayed vasospasm requiring endovascular intervention is usually seen 3 to 14 days following a severe subarachnoid hemorrhage, but can also be seen in postpartum patients, trauma, arteriovenous malformations, and tumors (6). The biochemical mechanisms of vasospasm are not completely understood, with various hypotheses explaining its pathogenesis (7):
A role for increased production of protein kinase C with increased production of vasoconstricting prostaglandins and inhibition of production of prostacyclin (a vasodilator) (8,9).
Increased protein kinase C leads to excessive intracellular activity of free calcium in smooth muscle, causing phosphorylation of contractile proteins (10).
Local depletion of nitric oxide (NO), which is an important tonic dilator of cerebral arteries by virtue of its activation of cyclic guanosine monophosphate (cGMP). Inactivation of NO by oxyhemoglobin or superoxide radicals may initiate this process (11,12).
Oxidative stress may lead to activation of calcium channels and of vasoactive proteins such as arachidonic acid to produce vasoactive lipids or, alternatively, bilirubin oxidation products causing vessel wall contraction (3).
Increased availability and potency of endothelin-1 (ET-1), a potent vasoconstrictor (13). ET-1 levels rise in response to shear stress, hypoxia, catecholamines, insulin, and angiotensin II and are counteracted by NO through the intermediary roles of endothelin-3, prostaglandin E2, and prostacyclin (14).
Angiographic evidence of vasospasm can be seen in 30% to 87% of patients following subarachnoid hemorrhage, but only about half of these will require specific management, and only the most severely affected of the latter group will require endovascular intervention (15,16,17). However, with recent use of diffusion imaging on MRI it is evident that the proportion of patients who develop infarctions may be much higher than previously believed, possibly as many as 50% to 80% even with optimal ICU management (17). Even with optimal Triple-H therapy (hemodilution, hypertension, hypervolemia) with nimodipine, as many as 13.5% of patients with subarachnoid hemorrhage die or become disabled from vasospasm, and among those disabled following a subarachnoid hemorrhage, DIND can account for almost 40% of the disabilities (18). Previously, these complications were ascribed primarily to the effects of vasospasm, although this is now in question. Nevertheless, a strong correlation exists between the severity of vasospasm and the likelihood of a bad clinical outcome due to ischemic deficits (19). Particular risk factors predicting a risk of severe vasospasm include a heavy burden of clot in the CSF, as categorized by the Fisher scale (20), and in female patients who smoke (21,22,23).
Microstructural changes in the vessel wall have been described in animal experiments and anecdotally in human patients, suggesting that the onset of vasospasm and
decreased wall compliance correlates with the development of smooth muscle vacuolation, myonecrosis, periadventitial inflammation, and medial and subendothelial fibrosis (24,25,26,27). The timeline of these events is clinically significant because it is a well-recognized phenomenon that spastic cerebral arteries become less compliant and responsive to intra-arterial drug instillation as the course of severe spasm evolves. Correlation between histologic mural changes and decreasing responsiveness to papaverine have been confirmed in monkeys (28,29); experiments also confirmed the transience of the response to papaverine with the effect lasting less than 24 hours and possibly as short as 10 minutes (30,31). These observations in animals dovetail with clinical anecdotal experience that the most intractable cases of persistent spasm become more difficult and hazardous to treat as the days elapse.
decreased wall compliance correlates with the development of smooth muscle vacuolation, myonecrosis, periadventitial inflammation, and medial and subendothelial fibrosis (24,25,26,27). The timeline of these events is clinically significant because it is a well-recognized phenomenon that spastic cerebral arteries become less compliant and responsive to intra-arterial drug instillation as the course of severe spasm evolves. Correlation between histologic mural changes and decreasing responsiveness to papaverine have been confirmed in monkeys (28,29); experiments also confirmed the transience of the response to papaverine with the effect lasting less than 24 hours and possibly as short as 10 minutes (30,31). These observations in animals dovetail with clinical anecdotal experience that the most intractable cases of persistent spasm become more difficult and hazardous to treat as the days elapse.
Cortical Spreading Depression and Other Causes of Delayed Cerebral Ischemia
Despite the emphasis for many decades on treating vasospasm as a means of reducing the likelihood of delayed ischemic deficits in patients with subarachnoid hemorrhage, several anomalous observations indicate that the cause-and-effect relationship between vasospasm and ischemia is not direct. With the exception of nimodipine, many drug trials with tightly argued hypotheses have succeeded in reversing the severity and extent of vasospasm but have failed to demonstrate an improvement in clinical outcome (32,33). For instance, clazosentan, an endothelin receptor antagonist, can reduce the severity of vasospasm by half in a dose-dependent manner compared with placebo, but does not improve clinical outcome at 3 months (34,35). At a more fundamental level, the correlation between the distribution and timing of vasospasm and the onset and location of cerebral infarctions is not consistent (36,37,38), and even the effects of Triple-H therapy on cerebral blood flow are unclear and not correlated with an impact on clinical outcome at 1 year (39,40). Quantitative studies of vasospasm using CT perfusion and PET suggest that even in territories with angiographically severe vasospasm, the ischemic threshold of cerebral blood flow (CBF) of 25 mL/100 g/min may not be reached in many severely ill patients, raising the question of why ischemic infarctions occur in this patient population (41,42).
The failure to detect a clear therapeutic effect on clinical outcome from systemic or intra-arterial drugs known to reverse cerebral vasospasm as well as other inconsistencies in the patterns of ischemic deficits following severe subarachnoid hemorrhage has shifted attention to other pathophysiologic mechanisms. These include microvessel spasm, distal microthrombosis (36,43), platelet activation (44), and the phenomenon of cortical spreading depression (45) (“killer waves of depolarization”) (46). Histologic postmortem evidence of thromboembolic mediation of cerebral ischemia in patients who have succumbed after subarachnoid hemorrhage is well-recognized, and transcranial Doppler evidence of microcirculatory emboli following open surgery or endovascular coiling appears to support the hypothesis that distal ischemia may be related to spontaneous platelet activation (37,43,44).
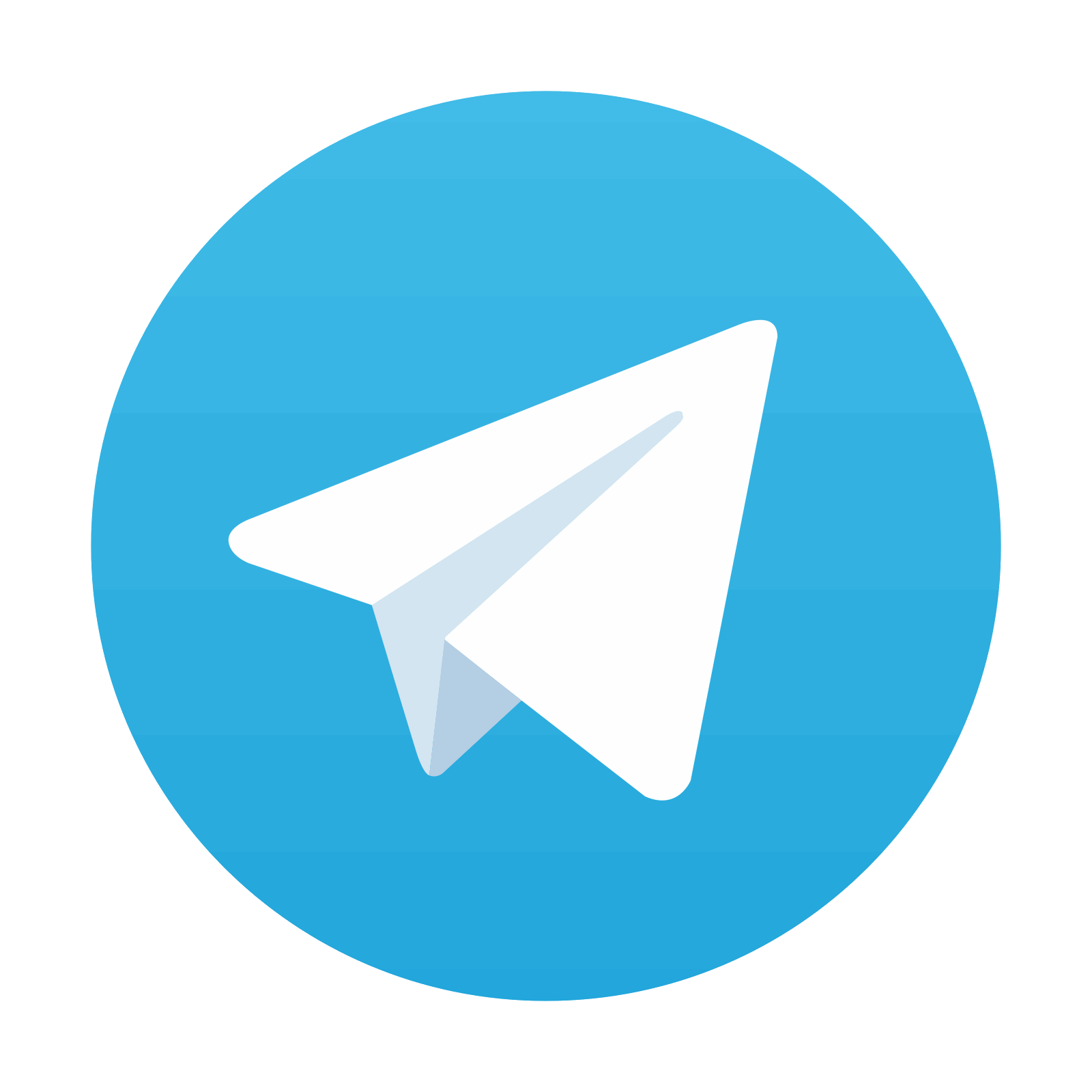
Stay updated, free articles. Join our Telegram channel

Full access? Get Clinical Tree
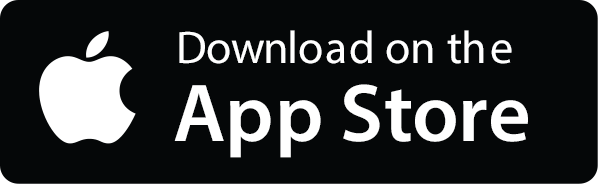
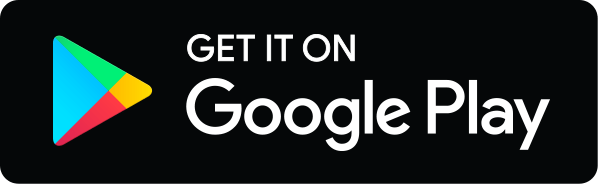