Fig. 5.1 a, b
a Lumbar puncture needles. Top: Quincke needle (yellow, 20 G × 312). Middle and bottom: Sprotte needle (green, 21 G × 312) and guiding trocar.
b Comparison of needle tips. Left: Sprotte needle with conical tip and lateral opening. Right: Quincke needle with sharply ground tip.
Relative contraindications. Coagulopathy is a relative contraindication. Thrombocyte counts lower than 50 000/μL increase the risk of hemorrhage. Thrombocyte counts below 20 000/μL require platelet substitution therapy prior to puncture. In a patient undergoing anticoagulant therapy with heparin, the heparin treatment must be stopped for 2 hours and the partial thromboplastin time (PTT) must have returned to normal. Heparin treatment may be resumed about 2 hours after puncture. If a patient has had effective oral anticoagulation, the prothrombin time (PT, Quick test) should have returned to at least 50% or the international normalized ratio (INR) should be at least 1.5. Acute situations require administration of prothrombin complex (PPSB).
Materials and Methods
Materials
Spinal puncture needles. Two different types of cannula are available in various lengths and diameters for cerebrospinal puncture (Fig. 5.1):
• Quincke needle: The Quincke spinal needle has a slant, sharply ground tip. This facilitates percutaneous passage; the needle can be easily advanced and, thanks to its elastic firmness, is easy to guide accurately. This cannula type is usually to be preferred in patients in whom puncture is difficult for anatomical reasons. Its disadvantage is that the relatively large diameter of the tip damages the dura, and a considerable amount of cerebrospinal fluid may leak from the puncture site once the cannula is removed (see “Postlumbar Puncture Syndrome” below).
• Sprotte needle: A widely used alternative is the flexible Sprotte needle, which, with its conical tip with a lateral opening, causes little trauma to the dura. The drawback is that penetration of the skin requires a sharply ground stylet, which serves as a guide for the puncture needle but does make it more difficult to correct the position of the needle.
Pitfalls in the Use of Quincke Needles
The puncture trauma caused by the Quincke needle can be reduced by turning the needle such that the sharply ground opening faces laterally during introduction. This way, the longitudinally running dural fibers are more pushed apart than cut through, and can rapidly close up again when the needle is removed.
CSF collection tubes. The tubes should be sterile, clean, and noncoated, and also crystal-clear so as not to obstruct the macroscopic assessment. A conical bottom of the tube is best for centrifugation because the cell pellet will collect there and the supernatant can be gently poured or pipetted off. Since the tubes are frequently sent away for analysis, tight closure is essential. Glass tubes are unsuitable, not only because of the risk of breakage but also because cells have an increased tendency to adhere to glass. Polystyrene is inert in this respect; it is also the most suitable material for DNA and RNA analysis and for measuring neurotransmitters and cytokines.
Performing the Lumbar Puncture
Patient education. The patient needs to be thoroughly informed about puncture of the subarachnoid space. For formal legal reasons, this must be done at least 24 hours prior to the procedure, except in cases of emergency. As with every invasive procedure, the patient should give consent in writing, either by signing the informed consent form provided (e. g., Perimed form) or without a form.
Positioning. Lumbar puncture may be performed with the patient sitting or lying down. In any case, it is a good idea to have the position supported by an assistant. It is essential that it is a relaxed position for the patient, with as much ventral flexion and stretching of the lumbar spine (arching of the back) as possible and with the legs flexed.
• In the sitting position, it is advisable for the patient to have both legs resting on a raised support (e. g., a chair), pulling the knees in toward the chest and bending the neck forward while grasping the knees with the arms. A pillow may be pushed beneath the thighs for support.
• In lateral decubitus, the patient is helped by another person to keep torso and legs flexed. When performing lumbar puncture on a patient in this position, the bed should be firm in order to avoid “artificial” scoliosis.
Puncture site. Lumbar puncture is performed between the spinal processes of the fourth and fifth lumbar vertebrae (L 4/L 5), or the third and fourth lumbar vertebrae (L 3/L 4). The L 4/L 5 interspace is easy to locate by palpating the right and left iliac crests and imagining a line between them that cuts the spinal process of vertebra L 4. The puncture site lies below this imaginary line, exactly on the midline.
Puncture between vertebrae L 2 and L 3 must be avoided, because the terminal cone of the spinal cord usually reaches down to vertebra L 2 and the risk of injuring the spinal cord is therefore high.
How to Perform a Lumbar Puncture
Mark the puncture site and thoroughly clean the area with a surgical disinfectant. From now on, it is mandatory that you wear sterile gloves and follow aseptic procedures. Anxious and tense patients may receive local anesthesia: after intradermal injection, infiltrate the deeper tissues with 1–2 mL of 1% or 2% lidocaine solution. After 2–3 minutes, stretch the skin around the puncture site using the thumb and index finger (or index and middle fingers) of the left hand and insert the puncture needle at the marked puncture site in slightly cranial direction. To help you guide the puncture needle securely, it makes sense to rest the arm lightly on an armrest. The depth of penetration varies: it is normally about 3.5–4.5 cm (considerably more in obese or muscular patients, less in children). When the needle penetrates the flaval ligament or the dura, you will feel a springy resistance. Then withdraw the stylet, keep it sterile, and let the CSF drain into the collection tubes. If there is no CSF dripping, reinsert the stylet and advance the needle very slowly. If the CSF still does not flow, pull back the needle toward the skin and then correct its direction. As a last resort, you can repeat the lumbar puncture one vertebra higher (at the L 3/L 4 interspace).
If the CSF drips very slowly, you may carefully turn the needle slightly. To check the needle position, have someone apply abdominal pressure; the drip rate should increase noticeably. If the needle position is correct, use the Queckenstedt test to check for free CSF passage. To do this, carefully press the jugular veins—again, the drip rate should increase noticeably.
If the needle is advanced too far it may damage the ventral venous plexus and there will be blood in the CSF. If the needle touches a nerve root, the patient will feel a sharp pain radiating into the leg (“like an electric shock”). If this happens, the needle must be pulled back immediately and its direction corrected medially.
Once the CSF has been collected, reinsert the stylet (still sterile!) into the puncture needle. Remove the needle, compress the puncture site with a sterile swab, and cover it with a sterile swab.
Postpuncture management. After lumbar puncture, it is sensible to have the patient lie prone for 1–2 hours to compress the puncture site and minimize CSF leakage from it. Although controlled studies have not confirmed that postpuncture bed rest after lumbar puncture has a favorable effect in terms of reducing postpuncture headache, in practice it has proven sensible to have several hours of bed rest and to avoid physical strain for at least 24 hours (Dieterich and Brandt, 1985; Cook et al., 1989).
Pitfalls in Performing a Lumbar Puncture
When collecting the CSF, it is important to ensure that neither the rim nor the lid of the collection tube comes into contact with the gloves, as powder particles from the gloves produce artifacts that would interfere with the interpretation of the cytological preparations.
Bloody CSF. If the CSF has the color of flesh or appears bloody, let it drip into at least three tubes (three-tube method) and compare the intensities of the blood admixed. Decreasing color intensities indicate artificial admixture of blood; the bloody CSF should be centrifuged immediately (200–300 g). A clear supernatant also indicates that the puncture fluid was artificially bloody.
Collecting CSF from the Ventricular Catheter
Today, ventricular CSF is collected almost exclusively by closed drainage systems. During the collection, which is usually by means of a three-way stopcock, it is essential that sterile precautions are carefully observed. The volume of CSF between the tip of the catheter in the ventricle and the collection site outside the ventricle is about 2 mL. Hence, the first 2 mL of CSF drawn should be discarded to ensure accurate analysis of the ventricular CSF. Even after the catheter has been clamped, because of the tendency of cell debris to form sediment within the system it is recommended that 5 mL CSF be drawn and discarded.
Complications of Lumbar Puncture
Puncture may cause the transient manifestation of low cerebrospinal pressure syndromes such as radicular irritation and, more commonly, postlumbar puncture headache.
Postlumbar Puncture Syndrome
Symptoms. Postlumbar puncture syndrome is characterized by headache, often accompanied by further vegetative symptoms such as nausea, hypotension, tachycardia, sweating, and orthostatic dysregulation. The symptoms appear with a latency period of several hours, in 80% of cases within 48 hours. Only in rare cases do symptoms last for more than 5 days. The headache mostly improves or disappears completely when the patient lies down. The headache is most prominent in the back of the neck (Schwarz et al., 1999).
Frequency. The frequency of postlumbar puncture headache reported in the literature varies widely; it is between 5% and 60% for diagnostic lumbar puncture and myelography, and between 1% and 40% for spinal anesthesia.
Etiology. Numerous factors favoring the occurrence of postlumbar puncture syndrome have been discussed in the literature; they include age, sex, body mass index (BMI), needle size (Flaatten et al., 1987; Müller et al., 1994; Hafer et al., 1997), needle diameter, direction of puncture, and bed rest after the puncture.
• Age, sex, and BMI: Patients aged below 60 years tend to suffer more often from postpuncture symptoms, and women are more often affected than men. A low BMI seems to favor the symptoms (Kuntz et al., 1992).
• Puncture hole: The decisive factor is the size of the puncture hole, which is determined by the size of the needle (needles > 22G must only be used for special indications) and by the form of the sharp tip (Fig. 5.1 b). With the Sprotte needle, postpuncture headaches develop in only 6.7% of cases; with the Quincke needle, the figure is 18.9% (Carson and Serpell, 1996; 22 G needles in both cases; n = 7580).
• Bed rest: Bed rest after lumbar puncture does not seem to play an important role. However, this view is based mostly on the comparison of at least 4 hours of bed rest versus resting for 24 hours, so that it seems advisable to recommend several hours spent mostly in bed and to avoid heavy physical activity for 1–2 days.
Pathophysiology. Low cerebrospinal pressure develops when more CSF is lost through the puncture site than can be compensated by production of new CSF. This leakage has been demonstrated by scintigraphy and magnetic resonance imaging (MRI). Low cerebrospinal pressure causes irritation of pain-sensitive intracranial structures (blood vessels, meninges, tentorium, and falx) when in the upright standing position. Cerebral vascular dilatation and reduced venous drainage also seem to play a role (Iqbal et al., 1995; Wang and Schmidt, 1997).
Rare Complications
Severe complications after lumbar puncture are extremely rare so long as contraindications are heeded.
Potentially, cerebral and spinal herniation, intracranial and spinal hemorrhage, cranial nerve paresis, and inoculation meningitis may occur.
Herniation. The use of imaging procedures to exclude elevated intracranial pressure has minimized the risk of herniation. Relatively, the risk of herniation is highest in acute bacterial meningitis:
• In 19 out of 445 children (4.3%), cerebral herniation was observed (Rennik et al., 1993). In 5 of these 19 children (26%), CCT was normal. In 12 children, the complication occurred within 12 hours after lumbar puncture.
• The autopsies of 27 out of 40 adults who died of bacterial meningitis (Durand et al., 1993) revealed temporal herniation, with 4 of them also showing cerebellar herniation. In 5 patients, herniation occurred within minutes or a few hours after lumbar puncture. The cerebrospinal pressure was documented in 4 patients and was greater than 50 cmH2O.
Spinal cord compression by a space-occupying mass. If there is an extramedullary space-occupying mass compressing the spinal cord, the release of CSF pressure during lumbar puncture may lead to increased pressure on the spinal cord distal to the compression, or it may damage the cauda equina.
Hemorrhage. In very rare cases, lumbar puncture may lead to subdural hematoma and hygroma even in the absence of clotting disturbances, when dural veins rupture due to low cerebrospinal pressure. A similar mechanism is postulated when there is postpuncture subarachnoid hemorrhage from an incidental basal aneurysm. After traumatic vascular puncture, spinal subdural and epidural hematomas and symptoms of meningeal irritation may develop due to hemorrhages from—usually—lateral venous and arterial vessels.
Inoculation meningitis. Bacterial meningitis due to inoculation during lumbar puncture is extremely rare (0.2%) if meticulous attention is paid to maintaining sterility, thorough disinfection, and avoidance of droplet infection.
Cranial nerve palsies. A similar frequency is reported for cranial nerve palsies, the most common one being sixth (abducens) nerve palsy, but the third, fourth, fifth, seventh, and eighth cranial nerves can also be affected. These effects are caused by low CSF pressure and appear after a latency period of 4–14 days; they usually disappear within 4–6 weeks.
Sensory irritation. If the puncture needle touches a nerve root, the sensory irritation eases off after a short time. However, patients often complain about nonspecific back pain for several days after the lumbar puncture.
References
Carson D, Serpell M. Choosing the best needle for diagnostic lumbar puncture. Neurology 1996;47:33–37
Cook PT, Davies MJ, Beavis RE. Bed rest and postlumbar puncture headache. The effectiveness of 24 hours’ recumbency in reducing the incidence of postlumbar puncture headache. Anaesthesia 1989;44:389–391
Dieterich M, Brandt T. Is obligatory bed rest after lumbar puncture obsolete? Eur Arch Psychiatry Neurol Sci 1985;235:71–75
Durand ML, Calderwood SB, Weber DJ, et al. Acute bacterial meningitis in adults. A review of 493 episodes. N Engl J Med 1993;328:21–28
Flaatten H, Rodt SA, Vamnes J, et al. Postdural puncture headache. A comparison between 26- and 29-gauge needles in young patients. Anaesthesia 1989;44:147–149
Gower DJ, Baker AL, Bell WO, Ball MR. Contraindications to lumbar puncture as defined by computed cranial tomography. J Neurol Neurosurg Psychiatry 1987;50:1071–1074
Hafer J, Rupp D, Wollbruck M, et al. The effect of needle type and immobilization on postspinal headache. Anaesthesist 1997;46(10):860–866
Iqbal J, Davis LE, Orrison WW, Jr. An MRI study of lumbar puncture headaches. Headache 1995;35:420–422
Kuntz KM, Kokmen E, Stevens JC, et al. Post-lumbar puncture headaches: experience in 501 consecutive procedures. Neurology 1992;42(10):1884–1887
Muller B, Adelt K, Reichmann H, Toyka K. Atraumatic needle reduces the incidence of post-lumbar puncture syndrome. J Neurol 1994;241:376–380
Rennick G, Shann F, de Campo J. Cerebral herniation during bacterial meningitis in children. BMJ 1993;306:953–955
Schwarz U, Schwan C, Strumpf M, et al. Postdural puncture headache: diagnosis, prevention and therapy. Schmerz 1999;13:332–340
Wang LP, Schmidt JF. Central nervous side effects after lumbar puncture. A review of the possible pathogenesis of the syndrome of postdural puncture headache and associated symptoms. Dan Med Bull 1997;44:79–81
Measurement of Cerebrospinal Pressure
The cerebrospinal or CSF pressure can be measured spinally (after lumbar puncture of the CSF space) or intracranially (e. g., using a ventricular catheter after neurosurgical intervention).
Indications
Lumbar Measurement
It would make sense to measure the opening pressure of the CSF with every lumbar puncture, but for pragmatic reasons this is usually not routinely done. The following “special” indications remain:
• If elevated intracranial pressure is suspected but imaging is normal (e. g., pseudotumor cerebri) → confirmation of diagnosis
• If imaging reveals hydrocephalus but no evidence of aqueductal stenosis → confirmation of normal-pressure hydrocephalus
Intracranial Measurement
Continuous measurement of intracranial pressure is indicated for the following purposes:
• Prophylactic diagnosis of critical situations.
• Decision–making for surgery (drainage, shunt, removal of hematoma, decompression).
• Evaluation of treatment. Hence, it is often carried out in patients with subarachnoid hemorrhage, craniocerebral trauma, space-occupying cerebral infarction, intracerebral hemorrhage, and severe meningitis.
Methods
Technique for Lumbar Measurement of Cerebrospinal Pressure
After puncturing the CSF space, remove the stylet from the puncture needle and immediately connect a sterile rising pipe (or air-filled infusion tube) with its three-way stopcock closed. Fix the infusion tube to a central venous pressure monitor (or a vertical ruler). Zero the monitor on the middle of the vertebra at the level of the puncture site. Now open the passage and wait until the CSF column has reached its maximum.
By applying pressure on both jugular veins for about 10 seconds (Queckenstedt test), the CSF pressure is elevated by 3–10 mmHg. When the compression is released, the pressure rapidly falls back to the original level. The clinical purpose of this maneuver is to check that the spinal CSF has free passage: if the passage is blocked, the rise in pressure will fail to occur. In such cases, the baseline value is often also very low.
After taking the measurement, close the system by means of the three-way stopcock, remove the needle, and transfer the CSF to a tube for analysis.
Results
Lumbar Measurement of Cerebrospinal Pressure
The pressure values obtained by lumbar measurement in a patient lying exactly horizontal and with free passage of spinal CSF correspond to the intracranial pressure (ICP).
Measured values vary slightly depending on heartbeat and respiration. Normal values in adults are between 60 and 200 mmH2O, corresponding to 5–15 mmHg (1 mmHg = 13.62 mmH2O). Values below 5 mmHg suggest a low cerebrospinal pressure (postlumbar puncture syndrome). Values above 15 mmHg indicate an elevated intracranial pressure. These values refer to measurements taken when the patient is positioned absolutely horizontally. Normal values for measurements taken in a seated patient cannot be established, since they differ individually because the water column between the cisterna magna and the puncture site varies depending on body size (Oschmann et al., 2003).
Pitfalls in Lumbar Measurement of Cerebrospinal Pressure
Falsely high values are measured in very tense patients. For this reason, if measured values are greater than 15 mmHg, the first thing is to check whether the patient is relaxed, and perhaps wait for a short time to see whether the CSF pressure falls when the patient relaxes. The pressure may also be slightly elevated in very obese patients (up to 20 mmHg). Shouting and coughing raise the CSF pressure considerably (≥ 20 mmHg and 30–110 mmHg, respectively). In patients ventilated with positive end-expiratory pressure (PEEP), the pressure is expected to increase by 1–2 mmHg per 50 mmH2O of PEEP.
Falsely low values are obtained if the needle tip is partially displaced. If pathologically low values are obtained, therefore, it is therefore advisable carefully turn the needle and check its position by applying abdominal pressure. Low CSF pressure can also occur with forced hyperventilation via reduced partial CO2 pressure.
Measurement of CSF Pressure via a Ventricular Catheter
Determination of intracranial pressure via a ventricular catheter—or via probes directly placed into cerebral tissue, or epidural probes—requires neurosurgical intervention and also calls for considerable experience in assessing the values obtained. The measurement should always be related to a defined reference system; in most cases, the reference system is the foramen of Monro.
Pitfalls in Measuring Cerebrospinal Pressure Intracranially
Since the hydrostatic pressure in a resting fluid depends on the height of the fluid column, the intracranial pressure within the central nervous system varies significantly. The anterior–posterior difference in the brain is 13 mmHg in supine and 10 mmHg in lateral decubitus. Accurate alignment of the measuring point and allowance for the position of the patient are therefore essential. In addition, there are numerous other sources of error that can lead to measurement inaccuracies: errors of the pressure transducer, problems with connecting the pressure transducer, inaccurate measuring because tubes are too long or too short, additive loss of CSF, or motor restlessness of the patient (Aschoff and Steiner, 1999).
Complications with Ventricular Catheters
Common complications with ventricular catheters include infections (6–7%), surgically induced hemorrhage (1%), and malpositioning of the catheter in the basal ganglia or mesencephalon. Particularly when the ventricular catheter remains in place for several days, it makes sense to examine the CSF regularly in order to identify infections early and exchange the catheter system.
References
Aschoff A, Steiner T. Messung von Hirndruck und Perfusionsdruck. In: Schwab S, Krieger D, Mallges W, eds. Neurologische Intensivmedizin. Berlin, Heidelberg: Springer; 1999
Oschmann P, Kunesch E, Zettl UK. Liquorpunktion-Indikationen, Techniken und Komplikationen. In: Zettl UK, Lehmitz R, Mix E, eds. Klinische Liquordiagnostik. 2nd ed. Berlin: de Gruyter; 2005.
Whiteley W, Al-Shahi R, Warlow CP, Zeidler M, Lueck CJ. CSF opening pressure: reference interval and the effect of body mass index. Neurology 2006;67:1690–1691
Zhong J, Dujovny M, Park HK, Perez E, Perlin AR, Diaz FG. Advances in ICP monitoring techniques. Neurol Res 2003;25:339–350
Cell count, Cytology
Indications
Conventional cytology. Qualitative differences between the cells in the CSF make it possible to demonstrate morphological equivalents of various diseases. The main indications are:
• Acute, subacute, and chronic inflammation of the CNS.
• Tumors of the CNS and meninges.
• Detection of absorptive reactions in case of hemorrhage and irritation.
To fully exploit the possibilities of CSF diagnosis, differential cell counts should be performed with every cerebrospinal puncture.
The frequent practice of performing differential cell counts only when the total cell count is above 20 cells/μL may lead to an incorrect diagnosis:
• In the presence of meningeal tumor infiltration, the total cell count may be normal.
• An old, not too extensive subarachnoid hemorrhage will indeed always cause a phagocytic reaction, but the total cell count is often not increased.
Immunocytology. Immunocytochemical methods are a useful supplement to conventional cytological CSF diagnosis. Their main diagnostic indication lies in the detection of malignant cells, especially when:
• The cells cannot be adequately characterized morphologically.
• It is difficult to distinguish in the differential diagnosis between inflammatory transformation and malignant transformation of lymphocytes.
• The tumor cell fraction is very small.
The detection of intracellular immunoglobulins is also a sensitive inflammation marker and the object of diagnostic and scientific investigations.
Preanalytical Requirements
Conventional cytology. The CSF should be collected in a tightly closing sterile, transparent, colorless, and uncoated polystyrene tube. Conical tubes are best for subsequent processing.
Cell analysis must be carried out within 1–2 hours, since even at 4°C cell loss becomes unpredictable after 2 hours.
For protein studies, CSF without cells may be stored for 1–3 weeks at 4°C. The time and site of collection should be given on the laboratory requisition form.
Immunocytology. Immunocytological CSF diagnosis requires especially rapid and careful processing of the cell preparation. The emphasis should be on gentle sedimentation, high cell yield, and celerity. A low rotational speed of 200–300 g during precentrifugation and 750 rotations per minute (rpm) during cytocentrifugation usually ensure that cells are well preserved and surface structures intact. Coated multi-field adhesive slides are preferred, since they increase cell adhesion and make it possible to apply the cells obtained by gentle centrifugation directly onto the microscopic slide. The sediment must be prepared within 1–2 hours and air-dried, and staining is best done immediately thereafter. Unfortunately, this is often not possible for logistic reasons, but it has been shown that either freezing the sediment or storing the cells in preservative can damage surface structures and thus prevent optimal staining.
Procedures
Cell Counts
The cell count is obtained using untouched CSF. For this purpose, the CSF should be fresh and gently mixed. The cell counting is mainly done under the microscope, usually in a Fuchs-Rosenthal counting chamber, less often in a Neubauer counting chamber. Increasingly, automated hematological analyzers are being adapted for counting cells in CSF and are used routinely in diagnostics.
Counting in a Chamber
Structure of the chamber. The Fuchs-Rosenthal chamber differs from the usual chambers for counting blood cells in having a larger surface area (16 mm2) and larger chamber depth (0.2 mm), thus yielding a larger volume of 3.2 μL (the volume of the Neubauer chamber is 1 μL). The surface area is subdivided into 4 × 4 large squares, with 16 small squares in each; i. e., it consists of a total of 256 small squares.
How to Use the Counting Chamber
Gently mix the fresh CSF.
Mix the CSF with a staining solution (e. g., 5.0 mL glacial acetic acid + 0.2 mL methyl violet + 1.0 mL absolute ethanol + distilled water to make 100 mL) to stain the nuclei of leukocytes and lyse the erythrocytes.
Using a leukocyte pipette or a small tube, dilute the CSF with the staining solution at a ratio of 10:1 (corresponding to a CSF dilution of 9 in 10).
Thoroughly mix the diluted CSF.
Place one drop into the counting chamber.
Wait a few minutes to let the cells sediment.
Count the cells under a microscope.
Determination of cell count. If the number of cells is low, all the squares are counted. If there are more than 20 cells in one large square, it is enough just to count the cells in four large squares and then obtain the total cell count (CC) by multiplication. With the usual CSF dilution of 9/10 and a chamber volume of 3.2 μL, the number of cells per microliter of CSF is calculated as follows:
Assessment of cell count:
Normal: ≤ 4 cells/μL.
Pathological: ≥ 5 cells/μL.
If the CSF is contaminated with blood, the total cell count must be corrected; for this purpose, deduct 1 cell per 1000 erythrocytes.
Automated Cell Counting
For automated cell counting, different cytometric systems are available: Advia 120 (Beyer), CellDyn 4000 (Abott), and UF-100 Urine Flow Cytometer (Sysmex).
Examination. The amount of CSF required for examination is 100–300 μL; it is mixed with a stain-fixative solution according to the instructions. Measuring can be done after 4 minutes; the stained cells are stable for a period of 4 hours. To ensure that no cells transferred from previously processed samples or from blood samples are included, three blank measurements must be made before the CSF sample is measured.
Results. Comparative studies have shown varying degrees of agreement with the manual cell count (Strik et al., 2005) depending on the number of cells and any possible contamination with blood. The UF-100 and CellDyn 4000 systems are able to differentiate bacteria or fungi from cells, thus preventing falsely high cell counts (Ziebig et al., 2000; Hoffmann and Janssen, 2002).
Samples containing blood or a lot of cell debris pose a problem; they require dilution at higher than 1:10 to allow less than 1500 erythrocytes/μL to be obtained.
The Advia 120 system also allows distinction between different cells. Comparative studies with differential cell counts under the microscope showed good agreement in respect of neutrophilic granulocytes (van Acker et al., 2001; Aune et al., 2004; Mahieu et al., 2004). The agreement in respect of monocytes and eosinophilic granulocytes was less good. With regard to lymphocytes, reports regarding the reliability of automated cell counting are completely contradictory (Strik et al., 2005). No studies are available regarding differentiation between transformed lymphocytes, plasma cells, macrophages, and tumor cells.
For the time being, current methods of automated differential cell counts by no means replace conventional CSF cytology under the microscope.
Concentration of Cells
Since CSF contains few cells, differential cell counts can not be performed on a smear as they are for blood—the cells have to be concentrated first.
Sedimentation Chamber Method and Cytocentrifugation
Requirements. A procedure for cell concentration should neither change the cell composition nor denature the cells during processing, nor should it change their original morphology. The procedure must be simple, quick, and easy to perform in a reproducible manner. The amount of CSF required must be small.
Methods. Two methods are generally used:
• Sedimentation chamber procedure: The Sayk sedimentation chamber, which has undergone various modifications, uses forced natural sedimentation of the cells. CSF (1–5 mL, depending on the cell count) is placed in a cylinder standing on a microscopic slide. The cylinder is held down by a spring, and liquid is sucked up by filter paper surrounding the cylinder. The cells thus sediment within 20–30 minutes and are then available for further processing.
• Cytocentrifugation: Using a cytocentrifuge, cell preparations can be produced within 10 minutes. At least 200 μL of CSF is placed in a cylinder. The CSF is sucked up by filter paper, as in the Sayk chamber, and is therefore unavailable for further diagnostic tests. The centrifuge should be set at 800 rpm.
Each method has its advantages and disadvantages (Table 5.1). Other procedures, such as the Kistler and Bischoff membrane filter method, and the use of prestained microscopic slides (test simplets) have not achieved general use, because they change the cell morphology or the preparations are unstable.
Microscopic slides. The slides for cell sedimentation are stored in 3% HCl-ethanol. Before use, they are thoroughly rinsed under tap water and dried.
Precentrifugation A widely used method for improving the yield of cells while maintaining cell morphology is precentrifugation.
Principle. CSF is centrifuged in a conical polystyrene tube for 8–10 minutes at 2200 rpm. The supernatant is pipetted off and stored for further diagnostic tests that can use cellfree CSF. The pellet is resuspended in at least 200 μL of a protein-containing medium (e. g., 9 mL medium 199 + 1 mL heat-inactivated fetal calf serum), mixed well, and pipetted into the cytocentrifugation chamber.
Advantages and disadvantages. The advantages of this method include an excellent cell yield, the possibility of preparing several sediments, the availability of the total supernatant for further protein diagnostics, and the cytoprotective effect of the medium. The method is disadvantageous when the CSF is mildly or moderately contaminated with blood and the cell image is obscured by overlying erythrocytes, making cell evaluation difficult. In addition, maintaining sterility is essential, since bacterial contamination of the medium leads to rapid growth of bacteria.
The medium needs to be stored in a refrigerator and should be prepared freshly every day.
Staining Methods
All staining methods used in hematology can be applied to CSF sediments. The cells become sufficiently fixed by airdrying; additional fixation is unnecessary and can change the cell morphology. Only Gram’s stain requires the sediment (without any medium) to be heat-fixed by flaming.
May–Grünwald–Giemsa stain. Standard staining uses the May–Grünwald–Giemsa method. The nuclei appear in different hues of purple and the cytoplasm in different hues of blue, thus making it possible to differentiate individual structures (Kölmel, 1978).
Fig. 5.2 Immunocytochemical staining of B lymphoblasts with a CD 19 antibody and alkaline phosphatase anti-alkaline phosphatase (APAAP) detection reaction.
May–Grünwald–Giemsa Staining Procedure
Air-dry the cell preparation.
Cover the slide for 3 minutes with May–Grünwald solution (eosin + methylene blue).
Drain staining solution and rinse with tap water.
Cover slide for 15–20 minutes with freshly prepared, filtered Giemsa solution (standard solution diluted 1:10 with tap water).
Drain staining solution and rinse with tap water.
Air-dry the preparation.
Special stains. The Berlin blue reaction detects iron-containing material. Sudan black or Sudan red stains fat particles. Periodic-acid–Schiff stain (PAS reaction) demonstrates mucopolysaccharides and glycogen. PAS-positive reactions are found in lymphoblasts and carcinoma cells, and also in macrophages associated with Whipple’s disease.
Immunocytochemistry
Principle. Antigenic structures on the cell surface or in the cytoplasm are labeled with specific antibodies. The antibody-labeled cells are visualized by coupling to a fluorescent dye, or they are stained by coupling to alkaline phosphatase (Fig. 5.2) or peroxidase. This makes accurate cell typing possible. Since usually only a few cells are available, microscopic methods are predominantly used. (Flow cytometric cell typing requires at least 10 000 cells and is available only in large centers.)
Procedure. To prevent nonspecific binding, CSF proteins have to be removed by washing with phosphate buffer; the preparation is then fixed with glutaraldehyde or methanol and then incubated with a target-specific monoclonal antibody. The optimal antibody concentrations vary; they have to be titrated for each individual antibody.
Selection of antibodies. The selection of antibodies is guided by the cell morphology, any known previous findings (such as a known primary tumor), and the clinical problem at hand. According to all of this, an antibody panel is assembled (Table 5.2). Double-staining with fluorescentlabeled antibodies makes it possible to assign antigens to certain cellular structures. However, fluorescent-stained preparations are perishable, so they have to be assessed immediately, and re-evaluation at a later time is not possible. Indirect staining of antibodies with alkaline phosphatase or immunoperoxidase allows long-term preservation of the preparations, thus ensuring correct assignment of antigenic structures to individual cell types.
Findings
Visual Assessment
Normal CSF is colorless and as clear as water.
Changes in color. If erythrocytes are present (> 1000/μL), the CSF is cloudy and pink; contamination with leukocytes (~ 1000/μL) results in an opaque-turbid to whitish-yellow color. In purulent meningitis, the CSF can be purulent-turbid due to an increased cell count, bacteria, and elevated protein content. The CSF can also be whitish-turbid when just the protein content is increased.
Fig. 5.3 Normal lymphocytes in CSF.
Fibrin contamination. The sediment may contain delicate or, in purulent meningitis, coarse fibrin clots and sometimes also cells. Tuberculous meningitis may lead to a severe increase in fibrin, with coarse fibrin clots (“spiderweb clots”), often containing mycobacteria.
Xanthochromia. Xanthochromia, a diffuse yellow to yellowbrown discoloration of the CSF, is due to contamination with hemoglobin derivatives originating from a hemorrhage that is several days old. Xanthochromia is caused by the presence of bilirubin; it is also found in severe hyperbilirubinemia.
How to Tell Hemorrhage from Artificial Contamination with Blood
Bloody CSF should be gently centrifuged (at 200–300g) as soon as possible, preferably immediately after collection. If the CSF has been artificially contaminated with blood, the supernatant is colorless and clear; if an old hemorrhage is the cause, the supernatant is xanthochromic. If the CSF stands for more than 60–90 minutes after collection, or is centrifuged at higher speed, the erythrocytes lyse and the hemoglobin derivatives mimic an old hemorrhage.
CSF may be xanthochromic during the first weeks of life if there is marked hyperbilirubinemia; also when the protein content is very high.
Normal CSF
Lymphocytes and Monocytes
Normal lumbar CSF contains two cell types, lymphocytes and monocytes, in a ratio of 7:3. If more than 4–5 mL CSF is collected and the cells are allowed to differentiate further in stored aliquots, the proportion of monocytes becomes higher. The CSF occasionally contains solitary granulocytes and, more frequently, a few fresh erythrocytes artificially introduced during lumbar puncture.
Fig. 5.4 Lymphocyte and monocyte in CSF.
Lymphocytes. Lymphocytes circulating in the CSF originate partly from the blood, into which they can migrate back, and partly from the arachnoid tissue. They are predominantly T lymphocytes; B cells amount to only 1–2%. Lymphocytes occurring in normal CSF are small and isomorphic; they have a compact round or slightly oval nucleus and a narrow rim of pale cytoplasm (Fig. 5.3). Mild lymphocytic transformation may occur even in normal CSF, with enlargement of the nucleus and cytoplasmic rim and increased basophilia of the cytoplasm.
Monocytes. Monocytes have either migrated into the CSF from the blood or stem from the leptomeninges or microglia. As mononuclear phagocytic leukocytes, they are slightly larger than lymphocytes (Fig. 5.4). They have an eccentric kidney- or horseshoe-shaped nucleus and a wider, more intensely stained cytoplasm which often contains vacuoles. Monocytes are unstable in vitro and degenerate rapidly.
In normal newborns, cell counts are often elevated until the 4th to 6th week of life, up to 10 cells/μL—in very premature newborns even up to 40 cells/μL. Cell counts above 40/μL after the 6th week are pathologic. The granulocyte fraction may be up to 50%. The monocyte fraction is often increased as well, with some of the monocytes showing considerable signs of activation. The erythrocyte count can also be markedly elevated as a result of birth-related manipulation or difficulties in the lumbar puncture. Erythrocyte counts of up to 1000/μL in mature newborns, and up to 20 000/μL in very premature newborns, are still considered normal (Hobusch, 2003).
Other Cells
Occasionally, cartilage cells get into the CSF as a result of lumbar puncture. They have a strongly acidophilic, cloudy cytoplasm, and a darkly stained nucleus (Fig. 5.5). Normal CSF may contain epithelial cells, and these are observed even more frequently after repeated punctures. They stem from the choroid plexus or the ependyma and are usually present in clusters. These cells are large with slightly basophilic or acidophilic cytoplasm, and the nuclei are usually marginal, round, pyknotic, and isomorphic.
Fig. 5.5 Cartilage cells in CSF.
Fig. 5.6 Phagocytes in a patient undergoing intrathecal chemotherapy.
Fig. 5.7 Signet-ring cell in a patient with breast cancer.
Fig. 5.8 Hemosiderophage with erythrocytes in a patient with subarachnoid hemorrhage.
Occasionally the bone marrow is injured during lumbar puncture, particularly in elderly patients and in infants. The cytological preparation then contains all stages of myelopoesis and erythropoesis (solitary or in cluster arrangements) and also plasma cells:
• Pronormoblasts (proerythroblasts) have a strongly basophilic cytoplasm and a large, round nucleus with nucleoli.
• Normoblasts (erythroblasts) show a small, coarse, and dark nucleus.
• Myeloblasts and promyelocytes, which are the largest cells; they have several prominent nucleoli which cease to be detectable during later maturation.
Phagocytes develop from the mononuclear phagocytic system; they remove foreign particles (cells, bacteria, viruses, fungi, pigments, lipids, and intrathecally administered substances). The phagocytic capability of these cells is extremely high; the phagocytosed material is deposited in the cytoplasm (Fig. 5.6) and often appears as an optically empty vacuole (signet ring cell, Fig. 5.7) or as numerous fine droplets, or vacuoles, as in lipophages.
Bloody CSF
Hemorrhage into the subarachnoid space triggers a violent leptomeningeal reaction leading to extensive pleocytosis of all cells of the hematopoietic system with transient predominance of granulocytes. After 4–6 hours, the mononuclear –phagocytic system is activated and the erythrocytes are phagocytosed (erythrophages). After 3–4 days, the hemoglobin derivatives are further degraded and dark-staining hemosiderin forms in the cells (hemosiderophages, Fig. 5.8). After 3–4 weeks, bright yellow hematoidin crystals develop that may be intra- or extracellular (Fig. 5.9).
Fig. 5.9 Hematoidin crystals in a patient with subarachnoid hemorrhage.
Pitfalls with Erythrophages in the Differential Cell Count
Even ex vivo, erythrocytes introduced by lumbar puncture and undergoing lysis after about 2 hours may still activate monocytes and initiate erythrocyte phagocytosis. Hence, the detection of one or two erythrophages in the differential cell count is not conclusive evidence of disease-related hemorrhage into the subarachnoid space.
Inflammation
Granulocytes
Granulocytes do not occur in normal CSF. They appear when the permeability of vascular walls in the subarachnoid space increases and toxins exert a chemotactic effect. The migration rate of granulocytes is then very high, and the CSF may be swamped with granulocytes within a few hours. The metabolism of granulocytes is based on anaerobic glycolysis; hence, granulocyte invasion is followed by elevated lactate levels.
Neutrophilic granulocytes. The nuclei of neutrophilic granulocytes are usually polymorphic, with several lobes strung together (Fig. 5.10); young granulocytes with rod-shaped nuclei are quite rare in CSF. The half-life of granulocytes is only a few hours in vivo and in vitro; it is therefore essential to determine the cell count immediately after CSF collection and to prepare a cell sediment. Neutrophilic granulocytes can invade the cerebrospinal space after any acute irritation, e. g., epileptic seizure, ischemic stroke, hemorrhage, and inflammation. They are therefore primarily a nonspecific sign of inflammation. The quantity and duration of granulocytes detected in the CSF permit conclusions on their genesis:
• Neutrophilic granulocytes are the real cells of acute defense against bacteria, and in bacterial infections can reach levels of several 10 000 cells/μL within a few hours. Even after successful treatment of bacterial inflammation they can still be detected for several more days (Fig. 5.11), but fall below 5% by about the 20th day of illness.
• In viral meningitis, granulocytes predominate during the first 3 days of illness, though rarely amount to more than 700/μL; after the 10th day of illness they are completely replaced by mononuclear cells (Fig. 5.12).
• In tuberculous meningitis and Listeria meningitis, granulocytes are still found after 30–40 days of treatment.
Fig. 5.10 Neutrophilic granulocytes.
Eosinophilic granulocytes. Eosinophilic granulocytes in CSF do not differ morphologically from those in the blood. Their nuclei have often the characteristic “spectacles” shape, but may have three segments. Typical are the eosinophilic granules in the cytoplasm, which are very resistant: even after cell lysis, isolated granules can still be detected in the CSF sediment. Small numbers of eosinophilic granulocytes appear during the healing phase of every inflammatory reaction (Fig. 5.13); they play an important role in allergic-hyperergic reactions and are frequently observed as a foreign body reaction after shunt operations. When eosinophilic granulocytes in the CSF persist over weeks and months, this is frequently due to zoonosis of the CNS (cysticercosis, hydatid disease, or other parasitic worm infestations). An eosinophilic tissue reaction detectable in the CSF is also observed with malignant tumors, particularly malignant lymphoma. The pathogenetic role of this eosinophilia is unknown and its prognostic relevance is the subject of much controversy (von Wasielewski et al., 2000; Axdorph et al., 2001; Dorta et al., 2002).
Basophilic granulocytes. Basophilic granulocytes are similar in size to neutrophilic and eosinophilic granulocytes, but their cytoplasm is packed with basophilic granules. Relatively little is known about the function of these cells; they appear during inflammation and interact with other inflammatory cells.
Fig. 5.11 Bacterial meningitis in the subsiding exudation phase: individual mononuclear cells.
Fig. 5.12 Viral meningitis: mononuclear pleocytosis, lymphocytic transformation, and a few granulocytes.
Fig. 5.13 Cryptococcal meningitis: neutrophilic, eosinophilic, and basophilic granulocytes.
Fig. 5.14 Transformed lymphocytes in neuroborreliosis.
Fig. 5.15 Plasma cells in the proliferative phase of bacterial meningitis.
Transformed Lymphocytes and Plasma Cells
Stimulated lymphocytes and plasma cells are characterized by an increase in the size of the cells, including the nuclei, and initially pale cytoplasm that becomes basophilic as the cells mature (Fig. 5.14). The nuclei may be slightly polymorphic and lie eccentrically, often surrounded by a bright perinuclear area. Plasma cells found in CSF have probably developed from small lymphocytes of the B cell system that have migrated from the blood into the CSF. The mature plasma cell has a round, eccentric nucleus with coarse chromatin, occasionally exhibiting a typical spoke structure and a bright perinuclear area (Fig. 5.15). Plasma cells are often binuclear (Fig. 5.16).
Even normal CSF may contain a small number of transformed lymphocytes and solitary plasma cells. Apart from this, their presence can also be the result of a monomorphic cellular reaction to a variety of different stimuli. They can appear in the course of any acute, subacute, or chronic inflammation, whether caused by pathogens or of autoimmune origin; they can be a nonspecific reaction to hemorrhage, intrathecal treatment, or surgery of the CNS; or they may be an accompanying reaction to tumor cell infiltration.
Fig. 5.16 Binuclear plasma cell.
Fig. 5.17 Typical mitotic figure (arrow) and transformed lymphocytes in neuroborreliosis.
Fig. 5.18 a, b Mitotic figures in meningitis and malignant melanoma.
a Typical metaphase (arrow) in a patient with meningitis.
b Dividing cell in anaphase (arrow) in a patient with meningeal metastases of malignant melanoma.
Following the acute exudative phase of bacterial meningitis, mononuclear cells with prominent lymphocytic transformations usually predominate during the proliferative and reparative phases; in viral meningitis, this is already seen during the first days of the illness. In neuroborreliosis (Fig. 5.17), the presence of marked lymphocytic transformation and plasma cells is striking, as is also the case in Epstein-Barr virus infection, which induces pronounced B cell activity.
Tumor Cells
For tumor cell diagnosis in the CSF, the generally established criteria of malignancy apply. There are often signs of increased cell division (Fig. 5.18 a, b). However, the absence of mitotic cells does not exclude the diagnosis of tumor cells. Malignant cells occur solitarily or in clusters, and the cell size may vary considerably. The nucleocytoplasmic ratio has shifted toward the nucleus. The nuclei are often highly polymorphic with more prominent and more numerous nucleoli and irregular, loose chromatin structure (Fig. 5.19). Nucleus and cytoplasm stain intensively. The cytoplasmic rim is irregular and often pseudopodia-like. It is not usually possible to assign tumor cells to a particular organ on the basis of lumbar puncture cytology. Cytoplasmic inclusions are more common with tumors of epithelial origin, whereas cells of mesodermal origin have a more homogeneously stained cytoplasm. But these criteria are too vague to serve as the sole basis for diagnosis.
Fig. 5.19 Leptomeningeal metastases of breast cancer: polymorphic tumor cells (arrows) in CSF.
Special Features of Ventricular CSF
Ventricular CSF and lumbar CSF differ more in their protein compositions than in their cell populations.
Catheter Irritation. In patients with indwelling ventricular catheters, the CSF often contains eosinophilic granulocytes due to irritation and phagocytic reactions or to intrathecal treatment. Tissue fragments and giant cells are often seen, particularly when the ventricular catheter is not completely patent or adheres to the ventricular wall. Irritation-induced granulocytic pleocytosis after surgical intervention, or due to material intolerance, is often much more pronounced in ventricular CSF than in lumbar CSF and can attain 300–400 cells/μL (Kluge and Kalff, 2003).
Meningitis. In pathogen-induced meningitis, the pleocytosis is usually markedly less pronounced in the ventricular CSF, since the turnover of ventricular CSF is much higher. However, the granulocytic phase usually persists longer in the ventricular CSF.
Meningeal carcinoma. In meningeal blastoma or carcinoma, the ventricular CSF is not representative of the total CSF findings. There are markedly more tumor cells in the lumbar than in the ventricular CSF, so treatment cannot be monitored on the basis of the ventricular reservoir alone.
References
Aune MW, Becker JL, Brugnara C, et al. Automated flow cytometric analysis of blood cells in cerebrospinal fluid: analytic performance. Am J Clin Pathol 2004;121:690–700
Axdorph U, Porwit-MacDonald A, Grimfors G, Björkholm M. Tissue eosinophilia in relation to immunopathological and clinical characteristics in Hodgkin’s disease. Leuk Lymphoma 2001;42:1055–1065
Chamberlain MC, Kormanik PA, Glantz MJ. A comparison between ventricular and lumbar cerebrospinal fluid cytology in adult patients with leptomeningeal metastases. Neuro Oncol 2001;3:42–45
Dorta RG, Landman G, Kowalski LP, et al. Tumour-associated tissue eosinophilia as a prognostic factor in oral squamous cell carcinomas. Histopathology 2002;41:152–157
Ellis MJ, Kazina CJ, Del Bigio MR, McDonald PJ. Treatment of recurrent ventriculoperitoneal shunt failure associated with persistent cerebrospinal fluid eosinophilia and latex allergy by use of an “extracted” shunt. J Neurosurg Pediatr 2008;1:237–239
Fulkerson DH, Boaz JC. Cerebrospinal fluid eosinophilia in children with ventricular shunts. J Neurosurg Pediatr 2008;1:88–295
Hobusch D. Besonderheiten der Liquordiagnostik im Kindesalter. In: Zettl UK, Lehmitz R, Mix E, eds. Klinische Liquordiagnostik. Berlin: de Gruyter; 2003
Hoffmann JJ, Janssen WC. Automated counting of cells in cerebrospinal fluid using the CellDyn-4000 haematology analyser. Clin Chem Lab Med 2002;40:1168–1173
Kluge H, Kalff R. Besonderheiten des Ventrikelliquors. In: Zettl UK, Lehmitz R, Mix E, eds. Klinische Liquordiagnostik. 2nd ed. Berlin: de Gruyter; 2005
Kölmel HW. Liquorzytologie. Berlin, Heidelberg: Springer; 1978
Mahieu S, Vertessen F, Van der Planken M. Evaluation of ADVIA 120 CSF assay (Bayer) vs. chamber counting of cerebrospinal fluid specimens. Clin Lab Haematol 2004;26:195–199
Strik H, Luthe H, Nagel I, et al. Automated cerebrospinal fluid cytology: limitations and reasonable applications. Anal Quant Cytol Histol 2005;27:167–173
Van Acker JT, Delanghe JR, Langlois MR, et al. Automated flow cytometric analysis of cerebrospinal fluid. Clin Chem 2001;47:556–560
von Wasielewski R, Seth S, Franklin J, et al. Tissue eosinophilia correlates strongly with poor prognosis in nodular sclerosing Hodgkin’s disease, allowing for known prognostic factors. Blood 2000;95:1207–1213
Ziebig R, Lun A, Sinha P. Leukocyte counts in cerebrospinal fluid with the automated hematology analyzer CellDyn 3500 and the urine flow cytometer UF-100. Clin Chem 2000;46:242–247
Further Reading
Hohlfeld R, Schwendemann G, Schwartz A, et al. Typisierung von Liquorzellpopulationen mit monoklonalen Antikörpern. In: Kölmel HW, ed. Zytologie des Liquor cerebrospinalis. Weinheim: VCH; 1986
Kluge H, Wieczorek V, Linke E, Zimmermann K, Isenmann S, Witte OW (eds.) Atlas of CSF Cytology. Stuttgart, New York: Thieme; 2006
Kölmel HW. Liquorzytologie. In: Zettl UK, Lehmitz R, Mix E, eds. Klinische Liquordiagnostik. 2nd ed. Berlin: de Gruyter; 2005
Kranz BR, Thiel E, Thierfelder S. ZNS-Befall bei lymphohämopoetischen Neoplasien: Inzidenz und immunzytologischer Nachweis im Liquor. In: Kölmel HW, ed. Zytologie des Liquor cerebrospinalis. Weinheim: VCH; 1986
Wick M. Immunzytologie. In: Zettl UK, Lehmitz R, Mix E, eds. Klinische Liquordiagnostik. 2nd ed. Berlin: de Gruyter; 2005
Proteins
Total Protein
Importance
Quantification of the total protein in CSF has two main purposes:
• Rapid emergency information about the barrier function.
• Guidance for subsequent analysis.
In Germany and most other European countries, the total protein value is not used for differential diagnosis (except for emergency analysis) because the CSF/serum albumin quotient is a much better indicator of blood–CSF barrier dysfunction; it is more sensitive and more specific.
Detection Methods
Possible procedures. While there is a reference method for total protein analysis in serum (biuret reaction), the same is not true for CSF: most of the procedures used for protein detection in serum are not sensitive enough for CSF analysis. Turbidimetric and nephelometric methods are currently used in addition to dye-binding reactions. Pandy’s test is a semiquantitative method that has been used in the past in the context of emergency analysis. In Europe, it has been increasingly replaced by quantitative total protein analysis. In developing countries, however, this simple, fast, and inexpensive phenol reaction is still in use.
Analytical tests. The best method of total protein analysis in CSF and other fluids is kinetic detection of the maximum intensity of scattered light in 40% trichloroacetic acid (TCA) (Reiber, 1980 a; Reiber, 1983; Chap. 4, this volume, “Immunochemistry”). This method may be recommended as a reference method in CSF analysis because it is mass-related, i. e., independent of the molecular form or amino acid composition of proteins, and is not influenced by any contaminations in the CSF.
Nephelometric two-point measurement in 20% TCA is far more prone to inaccuracy than kinetic measurement (Reiber, 1983). Turbidimetric assays with low TCA concentration or sulfosalicylic acid together with an endpoint detection method are biased by instability of the agglomerated proteins, which have a tendency to flocculate. The method using benzethonium chloride in alkaline solution yields a more stable and homogenous turbidity (Luxton et al., 1989). Dye-binding reactions with Coomassie blue and pyrogallol red–molybdate show different accuracies for proteins with different numbers of aromatic amino acids.
Thus, the dye-binding reactions depend on the fraction of aromatic amino acids, whereas precipitation reactions (e. g., turbidimetry) depend on the molecular form (globulin vs. albumin). Unbiased accuracy is achieved with kinetic nephelometry in 40% TCA.
Reference Range
The CSF reference values for proteins in Table 21.2 are provided only for orientation in laboratory analysis, not as a basis for clinical evaluation of CSF data. The relationship between total protein and QAlb in CSF is shown in Table 21.3.
Pitfalls of Total Protein in CSF
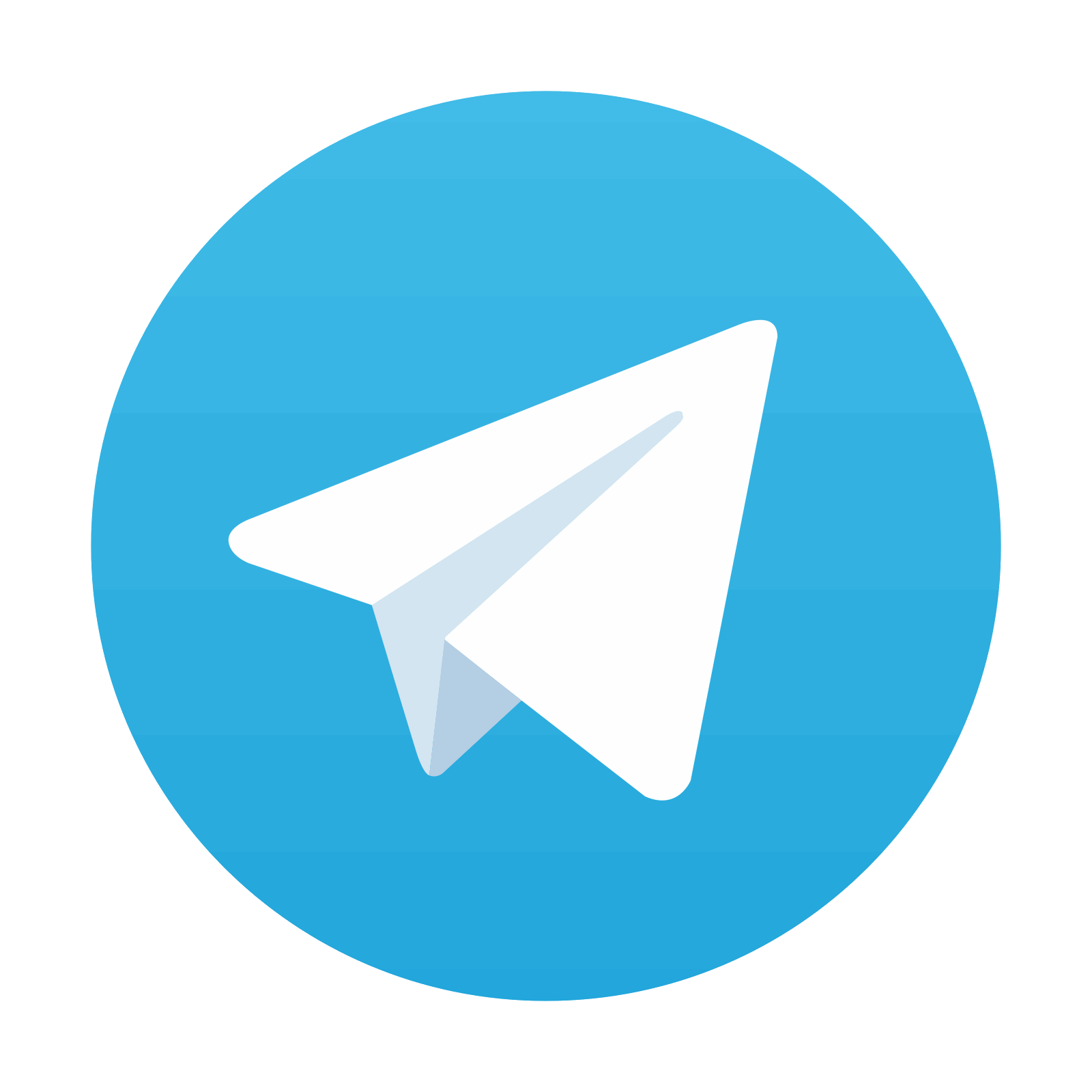
Stay updated, free articles. Join our Telegram channel

Full access? Get Clinical Tree
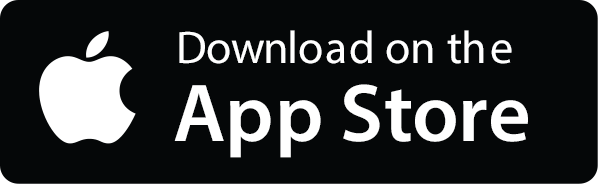
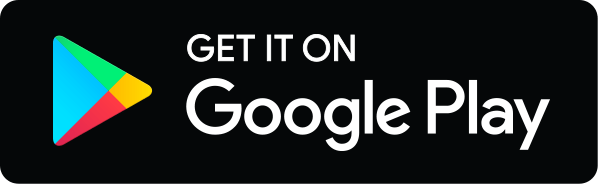