html xmlns=”http://www.w3.org/1999/xhtml” xmlns:mml=”http://www.w3.org/1998/Math/MathML” xmlns:epub=”http://www.idpf.org/2007/ops”>
Accurate ante-mortem diagnosis in frontotemporal lobar degeneration (FTLD) is essential for establishing a clinical prognosis and tailoring patient management. Moreover, in the light of potential future therapies, biomarkers discerning the underlying FTLD subtype as well as monitoring treatment response are indispensable. Till now, there is no specific biochemical marker for diagnosis of FTLD or one of its subtypes, nor for predicting the course of this disease. However, there is light at the end of the tunnel. Cerebrospinal fluid (CSF) tau, P-tau, and amyloid-β42 (Aβ42) are well-established CSF biomarkers that can be helpful in the diagnosis of FTLD by excluding Alzheimer’s disease. In addition, new approaches have been set in the last few years to discover new biomarkers, not only to certify FTLD, but also to differentiate between the different neuropathologic forms of FTLD. In this chapter we will give an outline of the CSF biomarkers that have been studied in FTLD till now.
Introduction
Cerebrospinal fluid (CSF) biomarkers may be of great use in establishing an accurate diagnosis of frontotemporal lobar degeneration (FTLD) or one of its subtypes. CSF protein composition is considered to reflect the pathologic processes taking place in the brain, and is therefore an ideal testing platform. Additionally, CSF is easily accessible, making it very useful for diagnostic purposes.
FTLD is a spectrum of disorders with similar clinical presentations, based on different underlying neuropathologic processes. As described in detail in Chapter 13, three main neuropathologic subgroups can be discerned [1–3]. The most common neuropathologic abnormality is FTLD-TDP in which accumulation of ubiquinated and hyperphosphorylated transactive DNA-binding protein of approximately 43 kDa (TDP-43) is found in brain tissue. FTLD-tau is another subtype characterized by accumulation of the microtubule-associated tau protein. The last and rarest form is FTLD-FUS, which is related histopathologically to fused in sarcoma (FUS) immune-reactive inclusions [4].
The ideal CSF biomarker in FTLD has to be highly sensitive and specific and apart from having a significant additional value over the clinical diagnosis, it should reflect neuropathologic processes and help to distinguish between the different pathologic forms of FTLD [5].
In Alzheimer’s disease (AD), CSF tau, P-tau (phosphorylated tau), and amyloid-β42 (Aβ42) have been well validated as CSF biomarkers. Additionally, a substantial number of papers have been published using these biomarkers in clinical dementia differential diagnosis. Motivated in part by that success, we have seen substantial research performed on CSF biomarkers in FTLD [6–15].
This chapter will outline the CSF biomarkers that have been investigated in FTLD so far. Hypothesis-driven approaches have been applied in studies investigating CSF levels of proteins involved in pathology or genetics of FTLD. Non-hypothesis-driven approaches have been used in technically sophisticated quests for differences between FTLD CSF protein patterns and other dementias and controls.
Hypothesis-driven CSF research in FTLD
Progranulin and TDP-43 in CSF of FTLD patients
The most common pathologic abnormality in FTLD-TDP is accumulation of ubiquinated and hyperphosphorylated TDP-43 in brain tissue. This pathologic hallmark occurs in genetically sporadic cases and has also been associated with progranulin (GRN) and chromosome 9 open reading frame 72 (C9orf72) genetic mutations (see Chapter 14 for details on the genetics of FTLD). Pathogenic mutations in the GRN gene are of various types and result in substantially reduced levels of progranulin (PGRN) in body fluids [16]. As a consequence, the most logical approach is to measure PGRN and TDP-43 in CSF. Nevertheless, the number of publications addressing this subject is still limited.
PGRN is a 593-amino acid secreted glycoprotein composed of a signal peptide followed by 7.5 tandem repeats of a 12-cysteinyl granulin motif that can be proteolytically cleaved to form a family of 6-kDa granulin peptides (GRN). Both PGRN and GRN peptides are believed to play a key role in cell cycle progression/development, cell motility, neuritic outgrowth, wound repair, and inflammation [17].
Current knowledge suggests that PGRN deficiency increases factors leading to cellular stress, and in combination with other disease modulators, these alterations could cause TDP-43 to mislocalize and become insoluble [16].
In one study, CSF PGRN was measured in three FTLD patients carrying a Ser82fs mutation in the GRN gene and this was compared with 24 controls; a threefold reduction of CSF PGRN levels was found in FTLD patients [18]. Two other studies showed also lower PGRN levels in CSF of patients carrying a GRN mutation [19, 20]. In these studies no diagnostic value was calculated owing to the small number of patients (n = 5 and n = 3, respectively).
Measuring serum PGRN levels has been shown to be a highly sensitive and specific screening method for identifying GRN loss-of-function mutations [21]. Investigating plasma levels of PGRN in patients with GRN gene mutations, significant lower levels of PGRN [19, 22] were found. Finch et al. reported a diagnostic value of nearly 100% (8 mutation carriers) [22], and Ghidoni et al. a specificity of 92.5% and a sensitivity of 100% [19]. However, in FTLD patients without GRN mutations, PGRN levels (in CSF and plasma) were comparable to those in control subjects [18–20, 22], and therefore PGRN is not a suitable biomarker for genetically sporadic FTLD.
The papers mentioned above report the specificity of monoclonal antibodies used and the workup/preparation of the enzyme-linked immunosorbent assay (ELISA). However, a complete validation of the ELISA is lacking; usually this includes the determination of a detection limit, recovery, stability, precision, and parallelism [23]. This is something to take into consideration for further studies.
TDP-43 is a 414-amino acid protein that contains two RNA recognition motifs (RRM1 and RRM2) and a glycine-rich C-terminal domain. TDP-43 can act both as a transcriptional repressor and as a splicing regulator. Although physiologic TDP-43 resides mainly in the nucleus, pathology-relevant TDP-43 redistributes from the nucleus to the cytoplasm where it is cleaved and forms phosphorylated and ubiquitinated inclusions [24].
One study found elevated plasma TDP-43 levels in about half of patients clinically diagnosed with FTLD and a quarter of patients with AD [25]. However, there was significant overlap in TDP-43 levels between the two groups. Additionally, some concentrations measured with the ELISA were extremely low, making these measurements less precise [25].
In CSF, there are no well-designed studies measuring TDP-43. An indication of increased CSF TDP-43 levels in FTLD and amyotrophic lateral sclerosis (ALS) was found in one study using a Western blot technique, which is less suitable to quantify TDP-43. Partly overlapping values with the control group were found [26]. Another study also showed increased levels of CSF TDP-43 in ALS patients, especially in the earlier stages [27]. The ELISA probably measured the full-length TDP-43. As a result, sensitivity and usefulness of TDP-43 as a biomarker in clinical practice could be improved by developing ELISA systems that are more specific for pathologic forms of TDP-43, including phosphorylated TDP-43 and its N- or C-terminal fragments. Additionally, CSF TDP-43 measurements in post-mortem-verified FTLD patients would possibly lead to an increased diagnostic accuracy.
CSF tau and P-tau in FTLD patients
FTLD-tau is characterized by pathologic accumulation of intracerebral tau protein, which has been associated with mutations in the tau gene, located on chromosome 17. Intracerebral tau pathology may also be seen in sporadic FTLD cases.
Tau proteins belong to the family of microtubule-associated proteins and are found in axons of neurons. Tau binds microtubules and is essential for growth, stabilization, and transport in neuronal axons.
Six tau isoforms are present in the human brain and they differ by the presence of one or two amino-terminal inserts and by the number of carboxy-terminal repeats (3 or 4 repeats). Different amounts of tau isoforms have been found in CSF of different tauopathies [28–30].
Abnormal phosphorylation of tau is one of the important events in the process leading to aggregation of tau. There are 79 potential serine and threonine phosphorylation sites on the longest tau isoform. Phosphorylation has been reported on approximately 30 of these sites in normal tau proteins. A correlation between the amount of pathologic tau in CSF and the severity of dementia has been demonstrated [31], indicating that this is a reliable marker for neurodegenerative processes. In addition, levels of CSF tau predict the rate of cognitive decline in AD [32–34] and this altered state reflects the elevated levels of P-tau in CSF. The most commonly studied P-tau epitopes are serine181 (P-tau 181) and threonine 231 (P-tau 231) [28, 29, 31].
In FTLD, several pathologic variants have been described in association with abundant intracellular and extracellular hyperphosphorylated filamentous tau inclusions. However, the mere presence of intra-cerebral tau pathology does not necessarily result in increased CSF tau levels. Normal CSF tau levels have been found in FTLD patients with tau mutations [35, 36].
CSF tau levels have been measured in several autopsy-confirmed studies, and the focus was mainly to differentiate FTLD from AD and controls [6, 35, 37–44]. These studies revealed that AD can be distinguished from FTLD and control subjects based on increased CSF tau levels. In FTLD, however, CSF tau levels were comparable to those of controls [6, 35, 37–44]. Looking for the diagnostic value to discriminate FTLD from AD, these studies found a sensitivity and specificity which reaches approximately 80–90%. Different biomarkers and combinations (tau/Aβ42 ratio, P-tau/Aβ42 ratio, and P-tau) have been used to calculate the sensitivity/specificity.
Within the different pathologic FTLD forms, one study investigated CSF tau in GRN-mutated patients, showing levels within normal limits [45]. Bian et al. have identified patients with pronounced low levels of CSF tau [35]. These include both FTLD-tau and FTLD-TDP patients; however, a lower trend (not significant) in CSF tau was seen for FTLD-tau patients [36]. Recently, one study showed a lower P-tau/tau ratio in FTLD-TDP compared with FTLD-tau, reaching a sensitivity of 82%. These findings have not yet been replicated [46].
In some of these studies mentioned, two different immunohistochemical platforms to measure CSF tau were merged [35, 36, 38, 44, 47]. The lack of convergence between these two methods needs further study.
Apart from studies in pathologically or genetically proven FTLD, including relatively low patient numbers, the majority of CSF studies in FTLD has been performed in clinically diagnosed cases (see Table 11.1). Although it is believed that clinical diagnosis results in approximately 10–20% underestimation of biomarker accuracy due to misdiagnosis [44], these studies may provide interesting information.
Schematic overview of CSF studies in FTLD patients (used in this chapter)
NFL | P-NFH | TAU | P-TAU | Aβ42 | Aβ40 | Aβ38 | Progranulin | TDP-43 | Path. confirmed | ||
---|---|---|---|---|---|---|---|---|---|---|---|
1 | Arai [82] | C < F | |||||||||
2 | Bian [35] | C, F < A | A < F,C | x | |||||||
3 | Bibl [56] | C < F < A | A < F < C | ||||||||
4 | Bibl [69] | F < C | F = A | F < C | |||||||
5 | Bibl [57] | A < F < C | F < A, C | F < A, C | |||||||
6 | Bibl [58] | F, C < A | F < C< A | A < F < C | F < C, A | F < C, A | |||||
7 | Blasko [13] | F < A | F < A | ||||||||
8 | Borroni [83] | C < F< A | C < F< A | ||||||||
9 | Borroni [84] | C < F< A | C < F< A | ||||||||
10 | Brunnstrom [41] | F < A | x | ||||||||
11 | Buerger [8] | C, F < A | C, F < A | ||||||||
12 | Carecchio [45] | F = C | PGRN mutation | ||||||||
13 | Clark [37] | C < F< A | A < F, C | x | |||||||
14 | de Jong [74] | C < F | C < F | F < A | F < A | A < F | |||||
15 | Engelborghs [59] | C, F < A | C, F < A | A < F < C | |||||||
16 | Fabre [85] | C < F | |||||||||
17 | Gabelle [60] | C < F< A | C, F < A | A < F < C | F < A, C | F < A < C | |||||
18 | Ghidoni [19] | F < C | PGRN mutation | ||||||||
19 | Gloeckner [9] | C < F, A | F, A < C | A < F, C | |||||||
20 | Green [14] | C < F< A | |||||||||
21 | Grossman [38] | C,F < A | C,F < A | A < F, C | x | ||||||
22 | Irwin [47] | F < A | x | ||||||||
23 | Kapaki [10] | C < F < A | C, F < A | A < F < C | |||||||
24 | Kasai [27] | C < ALS | |||||||||
25 | Koopman [40] | F < A | F < A | A < F | x | ||||||
26 | Matsuda [86] | F < A | |||||||||
27 | Mecocci [87] | C, F < A | |||||||||
28 | Molina [11] | F < A | |||||||||
29 | Paraskevas [88] | C < F < A | |||||||||
30 | Parnetti [12] | F < A | A < F | ||||||||
31 | Petzold [89] | C < F < A | |||||||||
32 | Philips [20] | F < A, C | PGRN mutation | ||||||||
33 | Pijnenburg [64] | C < F < A | A < C, F | ||||||||
34 | Pijnenburg [63] | C = F | C = F | C, F < A | C, F < A | A < C, F | |||||
35 | Pijnenburg [62] | A < C, F | F < C | ||||||||
36 | Riemenschneider [7] | C < F < A | A < F < C | ||||||||
37 | Rosso [65] | C < F < A | C, F < A | A < C, F | |||||||
38 | Schoonenboom [66] | C < F < A | C, F < A | A < C, F | |||||||
39 | Schoonenboom [6] | C < F < A | C, F < A | A < F < C | x | ||||||
40 | Sjögren [61] | C, F < A | A < F < C | ||||||||
41 | Sjögren [73] | C < F | C, F < A | ||||||||
42 | Sjögren [15] | C, F < A | F < C < A | ||||||||
43 | Steinacker [26] | C < F | |||||||||
44 | Toledo [44] | C, F < A | C, F < A | A < F < C | x | ||||||
45 | Van Damme [18] | F < C | |||||||||
46 | Verwey [67] | C < F < A | F, C < A | A < C, F | F < C | ||||||
47 | Landqvist Waldӧ [75] | C < A < F | F < A | F < A | A < F | x |
A = Alzheimer’s disease, F = FTLD, C = controls.
In clinically diagnosed FTLD patients, CSF tau values tend to be intermediate between AD and controls and thus most often were slightly increased. Since FTLD usually occurs before the age of 65 years, comparison with AD with early onset (EOAD) is relevant. When only patients with EOAD where compared with FTLD, the difference was even larger, showing much higher levels of tau in AD patients [48].
Considering P-tau levels, several studies found higher levels in FTLD compared with controls, whereas others show similar CSF P-tau levels. When comparing CSF P-tau levels between FTLD patients and AD patients, levels were higher in AD [48].
The diagnostic value of CSF tau and P-tau for identifying FTLD or one of its subgroups seems to be limited, since the differences between groups are small and sometimes inconsistent. Probably, both clinical misdiagnosis and heterogeneity of underlying FTLD pathology contribute to the variation of results. The discrepancy between the findings of a normal to decreased CSF tau in pathologically verified FTLD versus normal to increased CSF tau levels in clinically diagnosed cases, however, warrants further and larger studies in definite FTLD.
CSF amyloid in FTLD patients
Amyloid (Aβ) is formed after cleavage of the amyloid precursor protein (APP), which is a transmembrane glucoprotein. Proteolytic enzymes, α-, β-, and γ-secretase, cleave APP and Aβ protein is generated. The γ-secretase, which produces the C-terminal end of the Aβ peptide, cleaves within the transmembrane region of APP, and can generate a number of isoforms of 36–43 amino acid residues in length. The most common isoforms are Aβ40 and Aβ42. The Aβ40 form is the more common form of the two, but Aβ42 is more fibrillogenic [49].
It has been widely demonstrated that CSF tau, P-tau, and Aβ42 are reliable biomarkers to identify AD, even in its preclinical stages [50, 51]. The exact relationship between AD neuropathologic change (tau for neurofibrillary pathology and Aβ42 for extracellular plaques) and the observed measurement of these peptides in CSF is not known. CSF tau is thought to reflect underlying axonal degeneration. Lower CSF Aβ42 in AD may be the result of Aβ42 aggregation into extracellular plaques.
In FTLD no cerebral Aβ42 amyloidosis is seen and from this perspective, measurement of CSF Aβ42 does not seem useful to identify FTLD but may be only helpful in excluding AD neuropathology. Recently, however, several clinical AD cases have been described with AD pathology (confirmed by CSF, or PET, or autopsy), harboring GRN mutations [52–55]. A possible link between the GRN gene and the type of amyloidosis found in AD is therefore not excluded.
In pathologically confirmed cases, comparable levels of CSF Aβ42 were found in FTLD patients and controls (see also Table 11.1). Compared with AD patients, FTLD patients had higher CSF Aβ42. In a subset of pathologically confirmed cases, Aβ42 seemed to be lower in tau-positive patients than tau-negative patients; however, this was not significant [35, 38].
In clinically defined cohorts, higher levels of CSF Aβ42 were found in FTLD compared with AD [6–15]; compared with controls, different results were seen (see Table 11.1). Several publications show lower levels of Aβ42 in FTLD [6, 7, 10, 44, 56–61] whereas others show comparable results between FTLD and controls [35, 38, 62–67].
Besides Aβ42, also truncated forms of Aβ have been measured in CSF of FTLD patients, using various methods. CSF Aβ38 and Aβ40 seem to be lower in FTLD than controls [56, 62, 67, 68] but when FTLD was compared with AD, discrepant results were found. Several studies showed lower Aβ38 and Aβ40 in FTLD [57, 62]. Others found comparable results for Aβ40 and Aβ38 between AD and FTLD [67, 69]. One study applied Aβ40 in a model to discriminate FTLD from controls, showing a sensitivity of 87% and specificity of 90% [56]. However, this study used Western blot measurements, and cannot be compared with ELISA studies. Gabelle et al. found also lower CSF Aβ38 and Aβ40 levels in FTLD compared with controls and AD, measured with multiplex kits (Meso-Scale Discovery®) [60]. In this study, when combining P-tau and Aβ38, FTLD patients were correctly classified with a sensitivity of 85% and a specificity of 82%.
The suggestion that amyloid biomarkers are probably modified in FTLD raises many questions on the relationship between tau and Aβ biology. It has been hypothesized that common upstream drivers cause both intracerebral elevation of Aβ and tau hyperphosphorylation through independent but parallel mechanisms [70]. For example, one of the link hypotheses concerns functioning of the GSK3 (glycogen synthase kinase 3) enzyme. GSK3 phosphorylates the tau peptide to form P-tau which eventually will cluster in filaments. Additionally, GSK3 interacts with presenilin, which in turn is needed for γ-secretase cleavage of Aβ protein precursor to create Aβ [71, 72].
In summary, among patients with an FTLD-related clinical syndrome, a normal CSF AD biomarker profile is suggestive of underlying FTLD by ruling out AD pathology. The role of truncated Aβ forms in the diagnosis of FTLD needs to be further established.
Neurofilaments in FTLD patients
Neurofilament (NF) proteins are major constituents of the neuronal cytoskeleton. Localized in large neurons and myelinated axons, they play an important role in neuronal structure. NFs consist of three polypeptides: the light (NFL, 60 kDa), medium (NFM, 150 kDa), and heavy (NFH, 190–210 kDa) subunits. NFs are likely to be released into the extracellular fluid following neuronal death and axonal degeneration.
Increased levels of NFs in CSF may reflect neuronal degeneration in neurologic disease. Analyses of NFs in CSF of dementia patients have shown conflicting results. CSF NFL levels were increased in FTLD patients compared with controls [73–76]. However, others found comparable results between controls and FTLD patients [63]. These potential CSF biomarkers need further study; to date, to our knowledge, no studies have reported CSF NF measurements in pathologically confirmed FTLD cases.
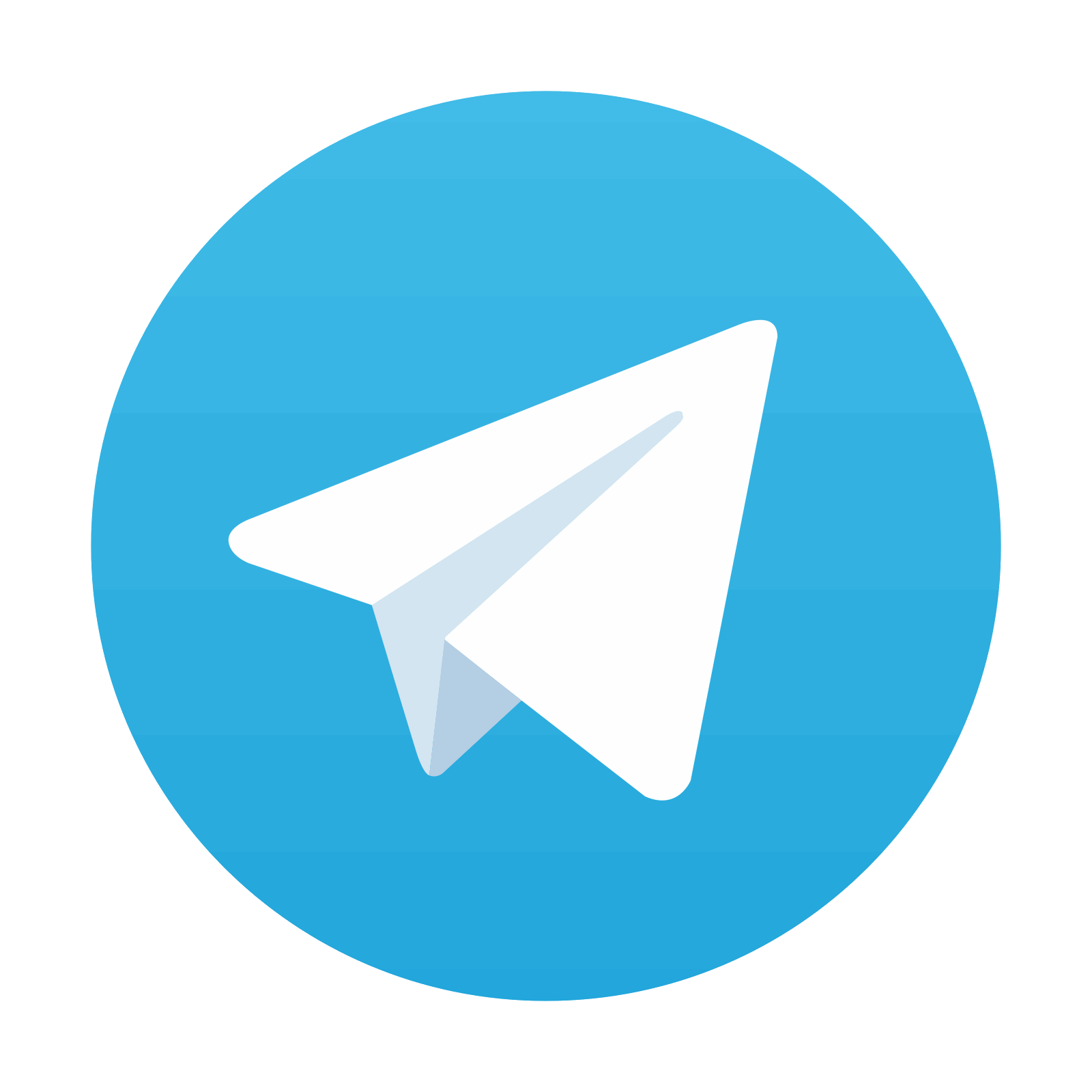
Stay updated, free articles. Join our Telegram channel

Full access? Get Clinical Tree
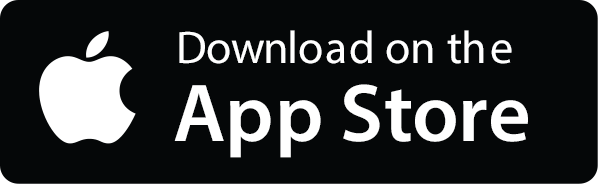
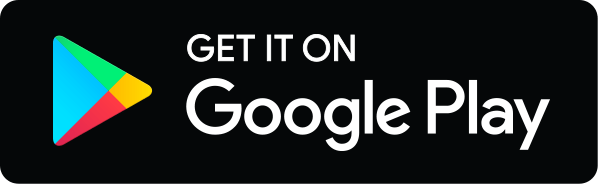