Summary of Key Points
- •
Spine constructs should be patient and pathology specific.
- •
Most constructs require supplementation with adequate bone grafting to provide long-term stability.
- •
Cervical spine constructs may be applied in situations of clinical instability, maintenance or correction of alignment, or treatment of refractory pain.
- •
Most cervical spine constructs are applied in the neutral mode.
- •
Cervical constructs usually conform to one or more of five basic fixation and load-bearing types: distraction, tension-band, three-point bending, fixed moment arm cantilever beam and nonfixed moment arm cantilever beam.
Fundamental Concepts
The successful application of cervical spine instrumentation depends on several factors, including the nature and extent of the disease process, bone quality, and the technical expertise of the surgeon. One of the most crucial, but often overlooked, elements in this process is determined well before the operative procedure is undertaken. This is construct design.
The term construct is a neologism that has become entrenched in the spinal literature. For the purpose of this discussion, a construct denotes the aggregate of biologic or nonbiologic materials that are implanted for the purpose of providing stability to an unstable region of the spine. Construct design, then, is the process of contriving such an implant. For the most part, this chapter addresses the design of constructs composed of bone and instrumentation for application in the subaxial cervical spine.
Without a sound construct design strategy, cervical fixation systems are doomed to failure. The meticulous technical application of a poorly conceived construct is a futile exercise, as prone to failure as the correct system improperly applied. Despite its importance, relatively little emphasis has been placed on this element of the procedure. This chapter presents a strategy to aid in the selection of certain instrumentation systems designed for specific clinical problems of cervical spine instability. The specific advantages and shortcomings of each type of construct are also discussed.
Benzel described an excellent method for preoperative mapping of thoracic and lumbar instrumentation procedures, using a “construct blueprint.” This approach is practical in this region of the spine, because the choice of implant components that may be applied here is vast. The design of thoracolumbar constructs entails selection of the longitudinal member, cross-fixation mechanism, and implant-bone junction fixators. Each element may be different at various levels of a long construct, adding to the complexity of the system.
Additionally, the modes of construct application that may be used in the thoracolumbar spine are extensive. This refers to the desired forces that are applied by the surgeon at the implant-bone junction. Constructs may be placed in compression, distraction, neutral, translation, flexion, extension, and lateral bending modes. Several modes of application may be required in a single thoracolumbar construct, depending on the structural demands at any given level. A systematic approach to the formulation of an operative plan is essential when designing constructs with this degree of complexity. The construct blueprint is a concise format capable of communicating complicated surgical strategies to all members of the operative team.
The options concerning surgical approaches and types of fixation devices are more limited in the cervical region. The mode of application here is also less variable, because most cervical constructs are applied in the neutral mode. Although this simplifies the cervical construct design scheme, the need for cogent preoperative planning is just as essential. The format used to communicate the operative strategy is less important than the intellectual process of visualizing the biomechanical requirements of a given lesion and formulating an appropriate construct that satisfies these requirements.
The fundamental steps for appropriate construct design are to determine the need for instrumentation, select the construct best suited to solve the instability problem, and ascertain the need for postoperative orthotic stabilization to supplement the implant.
Indications for Cervical Construct Application
White and Panjabi outline four general indications for spinal stabilization: (1) to restore clinical stability to a spine in which the structural integrity has been compromised, (2) to maintain alignment after correction of a deformity, (3) to prevent progression of a deformity, and (4) to alleviate pain. Cervical spinal instrumentation may be applied in conjunction with a bone fusion in all of these scenarios. In rare instances, instrumentation may replace bone fusion as the principal means of cervical stabilization.
Optimally, internal fixation provides immediate postoperative stability to the region before the development of osseous fusion. Instrumentation thus protects the neural elements from trauma and the spine from deformity, until the bony fusion matures and can assume this role. Internal fixation also obviates, or at least significantly reduces, the requirement for postoperative external immobilization while the fusion mass heals. This technique improves patient comfort, which encourages accelerated mobilization after surgery. Additionally, this may enhance the probability of attaining successful bone fusion by ensuring compliance with postoperative immobilization.
Internal fixation may allow a reduction in the number of levels that require fusion by adding intrinsic strength and load-sharing properties to the construct. A shorter fusion facilitates the preservation of cervical motion and limits the resultant moment arm created by the fusion mass.
Clinical Instability
The most frequent indication for cervical instrumentation is instability. To paraphrase an oft-quoted general definition, instability requires the loss of spinal biomechanical integrity such that the spine is unable to prevent initial or additional neurologic deficit, major deformity, or incapacitating pain under physiologic loads. In practice it is essential to determine precisely the nature and extent of spinal instability. The nature of instability refers to the status of specific structures that normally confer stability on each motion segment in the cervical region. This concern addresses the competency of the ligamentous structures, bony elements, and annulus fibrosis of the intervertebral disc. Identification of the incompetent elements allows the severity of segmental spinal instability to be estimated. The extent of instability denotes the number of unstable motion segments, as well as whether the instability is predominantly ventral, dorsal, or both. Defining these concepts precisely is of fundamental importance, having an impact on the decision to instrument the spine and also dictating the selection of an appropriate construct.
The etiology of spinal instability is important. Symptomatic cervical instability may result from trauma, degenerative disease, neoplasia, or infection. Iatrogenic instability may also occur, particularly after cervical laminectomy for spondylotic disease. Construct design is influenced by the nature of the disease process that produced the instability, as the long-term structural demands placed on a construct are often determined by the progression or remittance of the underlying disease. Posttraumatic instability may demand the least of a construct: short-term immobilization is often all that is required to promote adequate healing. After the injury heals, the load-bearing and load-sharing properties of the construct are no longer required to maintain stability. Spondylotic and iatrogenic instability may require more from a construct, owing to the slowly progressive nature of the process. Instability arising from spinal neoplasia often mandates long-term participation by the instrumentation to maintain structural integrity. Bone fusion may not be attainable because of the rapid progression of disease or adjuvant use of radiation therapy: the instrumented construct must be designed to bear physiologic loads for the remainder of the patient’s life.
Maintenance of Alignment
Internal fixation may be indicated to prevent deformity from occurring or to preserve normal alignment after reduction. Unlike thoracolumbar instrumentation, cervical constructs are generally applied in the neutral mode, thus deformity reduction should occur before stabilization. Many constructs designed for use in the thoracolumbar spine can apply significant compressive, distractive, translatory, and rotatory forces to a region of spinal deformity, thus affecting reduction. As a rule, most cervical instrumentation systems cannot apply the magnitude of force required to reduce a deformity and are used predominantly to maintain reduction.
Prevention of spinal deformity may also be accomplished by the timely use of internal fixation. Progressive kyphosis or spondylolisthesis may result from spinal decompression procedures. If individuals at risk for this complication are identified preoperatively, cervical deformity may be preventable. Patients exhibiting a loss of the normal cervical lordotic configuration are prone to develop postlaminectomy kyphosis, which may be avoided by proper internal stabilization at the time of decompression. Similarly, operative resections that compromise principal load-bearing elements may render the spine incompetent to withstand physiologic loads and deformity may be prevented by spinal reconstruction, using bone graft and instrumentation to reconstitute the axial spine.
Pain Management
Spinal stabilization may be indicated to relieve incapacitating pain by reducing motion between spinal segments. This concept has been applied more extensively in the lumbar spine, particularly for treatment of mechanical low back pain arising from spondylolysis and subsequent degenerative spondylolisthesis. Fusion of the cervical spine purely for amelioration of axial pain may benefit certain patients greatly but selecting them is a significant clinical challenge. Such a procedure should be carefully considered and only performed after conservative treatment measures have failed.
Construct Selection
Cervical constructs should be designed to solve case-specific problems of spinal instability. This requires an understanding of the nature, extent, and causes of instability; load-sharing and load-bearing demands; bone integrity; and biomechanical attributes of various internal fixation systems. Implant cost and ease of application are also important concerns. Constructs may fail as a result of poor design, usually because biomechanical expectations of the implant were unreasonable. Two general rules help guide the selection of a cervical construct and limit unrealistic expectations: (1) the graft and implant must correct the specific preoperative instability, and (2) the long-term success of a cervical construct ultimately relies on the quality of the osseous fusion.
General Considerations
In most cases, cervical constructs are used to maintain clinical stability. This may be accomplished most efficiently by matching the implant with the major site of instability—that is, if the instability is primarily dorsal in location, a dorsal construct should be considered for stabilization. Similarly, ventral instability, created by incompetence of the anterior longitudinal ligament (ALL), vertebral body, or intervertebral disc complex, is most effectively treated by the application of a ventral construct. It is unreasonable to expect that a construct will function with optimal stability when implanted in a biomechanically disadvantageous position.
Internal fixation systems provide immediate postoperative stability to the instrumented region, but they do not provide long-term stability due to the “plastic” properties of bone at the implant-bone interface. As with most biologic materials, bone deforms and reforms when stress is applied. Eventually, even the most rigid construct allows a small degree of motion. Repetitive loading gradually increases the amount of movement and can ultimately lead to implant failure, unless bony fusion occurs. The long-term stability of all constructs is thus dependent on osseous fusion: no internal fixation system currently available can compensate for a poorly designed bone graft.
Cervical spinal implants may be considered as rigid, semirigid, or dynamic. Rigid implants attempt to achieve complete immobilization of the instrumented motion segments. Ventral plate systems, with locking screws and dorsal rod and hook/rod systems, provide rigid fixation. Luque rods and rectangles (Zimmer, Warsaw, IN), secured with segmental sublaminar or facet wires, and most lateral mass plate devices are examples of semirigid cervical implants. Rigid immobilization is potentially detrimental to bone fusion because of stress shielding and stress-reduction osteopenia. This concern has led to the development of dynamic instrumentation, such as nonfixed moment arm cantilever beam screw-plate implants and axially dynamic ventral fixators.
Modes of Application
The modes of application available for cervical constructs are more limited than those available for use in other spinal regions. Thoracolumbar implants may be placed in distraction, compression, neutral, translation, flexion, extension, and lateral-bending modes. In contrast, cervical spine constructs are generally applied in the neutral mode. This is not universally true, because certain cervical plate systems and wire constructs may provide a modest degree of compression. Theoretically, cervical rod/screw (or hook) devices can be placed in the compression or distraction modes as well. However, the majority of cervical constructs in clinical use are applied in the neutral mode at the time of surgery. Biomechanical conditions change as the spine is loaded after surgery. Most “neutral” implants must resist axial compression when the upright posture is assumed. These constructs then function in a distraction mode.
Cervical construct designs are also more limited in their mechanism of load bearing than their thoracolumbar counterparts. Generally, cervical constructs conform to one of five fundamental load-bearing types: (1) distraction fixation, (2) tension-band fixation, (3) three-point bending, (4) fixed moment arm cantilever beam, and (5) nonfixed moment arm cantilever beam fixation. Applied moment arm cantilever beam fixation, a technique occasionally applied in the thoracolumbar spine, is not used in the cervical spine. Assigning an implant to one of these fundamental load-bearing types is somewhat artificial, because a given construct may exhibit features of several mechanical types. However, it permits classification of implants by their principal biomechanical attributes.
Simple Distraction
Simple distraction fixation occurs when a distraction force is applied by a cervical construct, usually from a ventral, interbody location. Interbody strut grafts are the most common examples of this type of fixation. These devices principally resist axial loads. Dorsally applied interfacet distraction is seldom used because it may cause kyphosis when improperly applied. However, this method has been shown to be a safe and useful adjunct to increase foraminal area.
Tension-Band Fixation
Tension-band fixation is accomplished by any device that reconstitutes the ventral or dorsal tension band, thereby preventing distraction, and also possibly angulation, in the opposite direction. This type of fixation may be applied dorsally with interspinous wires or cables, sublaminar wires or cables, facet wires or cables, interlaminar clamps, or lateral mass screws and rods. These dorsal devices resist flexion most efficiently, because the flexion moment is coupled with dorsal distraction. Ventral tension-band fixation is accomplished principally with ventral cervical plate systems. These implants reconstitute the ventral tension band, thereby resisting ventral distraction and providing sound biomechanical stabilization of extension injuries.
Three-Point Bending
Three-point bending fixation occurs when forces are applied to the spine at three or more sites along the length of the construct. Dorsally directed forces are applied at the rostral and caudal ends of the construct. An equal but opposite ventrally directed force is applied at the fulcrum, usually in the center of the construct. Three-point bending instrumentation is applied dorsally in the cervical spine so to create lordosis and must fixate multiple motion segments. This type of fixation has been historically accomplished with Luque rods/rectangles secured with sublaminar wires or cables, lateral mass rib-wire constructs and hook/rod implants but is today more usually accomplished with lateral mass screws and rods.
Cantilever Beam Fixation
A cantilever is formed by a projecting beam supported at one end only. When the cantilever is rigidly attached to the supporting longitudinal member, a fixed moment arm cantilever beam is created. This variety of load bearing is accomplished by ventral cervical plate systems secured with locking screws and rigid lateral mass screw/rod instrumentation. A fixed moment arm cantilever beam device contributes some axial load-sharing properties to the construct. Nonfixed moment arm cantilever beam fixation employs a dynamic attachment of the cantilever to the longitudinal member: axially dynamic ventral fixators are the most common example of this type of load bearing today.
The classification of spinal implants by mechanism of load bearing is somewhat artificial. In practice, a single implant may function by using several of the fundamental load-bearing mechanisms simultaneously. For example, the lateral mass screw/rod construct is capable of stabilization by three such mechanisms: dorsal tension band, three-point bending, and fixed moment arm cantilever beam fixation may all be accomplished.
Construct Materials
A variety of biologic and prosthetic materials have been used for cervical spine stabilization. Most constructs are composed of a bone graft, coupled with a metal prosthesis. Occasionally, bone or metal components may be supplemented or replaced by methyl methacrylate or plastic.
Bone Grafts
Autograft and allograft bone have both been used extensively in spinal stabilization. High fusion rates are reported using either autograft or allograft but may be marginally higher with autograft. The use of autograft bone eliminates the very small concern of infectious disease transmission that may be associated with allograft bone, including human immunodeficiency virus (HIV) and hepatitis virus transmission, but carries the risk of donor-site morbidity.
The iliac crest provides a versatile and abundant source of bone graft material for incorporation into cervical spine constructs. Favorable attributes of this type of graft include ease of procurement in the supine and prone positions, strength, and relative expendability of the donor site. The tricortical structure of the iliac crest is responsible for much of the strength inherent in this graft, thereby providing excellent axial load-bearing capability. The abundant cancellous bone provides ample substrate for osseous remodeling. Although all commonly used configurations of iliac crest grafts can sustain high compressive loads, the Smith-Robinson type graft is probably superior to other styles of grafts in this respect. The principal disadvantage associated with iliac crest harvest is donor site morbidity, which may be substantial. Complications include pain, wound hematoma, infection, meralgia paresthetica, hip dislocation, and fracture of the anterior superior iliac spine.
Fibula is another commonly used graft material. It is particularly well suited for multilevel ventral reconstruction procedures, because the thick cortical bone in this graft resists high axial compressive loads. The relatively small amount of cancellous bone present in the fibula graft may delay bone remodeling, however. This may be partially overcome by packing additional cancellous bone in the center of the graft, as well as surrounding the outer cortical surface with the cancellous bone. Donor site morbidity arising from graft harvest may be significant, because one sixth of body weight is borne by the fibula so it is basically employed today from cadaver donors, particularly for reconstruction after anterior corpectomy due to its shape.
Rib grafts have also been used, particularly with dorsal cervical constructs. The native configuration of rib is advantageous because it conforms well to the cervical lordotic curve. There is minimal morbidity in harvesting rib as compared to iliac crest, rendering it an excellent option for posterior reconstructions.
Implants
Currently most spinal implants are fashioned from metal. Stainless steel was once used extensively for the manufacture of wires, cables, plates, screws, hooks, and rods used in spinal constructs, but its use has been largely discontinued due to the advent of newer titanium alloys. These alloys possess a relatively high tensile strength while retaining a reasonable degree of malleability, often required to tailor a component to anatomic specification, and are biocompatible. These alloys further facilitate postoperative imaging because they do not generate the significant artifact stainless steel does on magnetic resonance imaging (MRI) and computed tomographic (CT) imaging. Other synthetic materials have substituted titanium alloys in cervical constructs, particularly interbody cages. The most widely used are polyetheretherketone (PEEK) and carbon fiber cages. Their most striking advantage is relative radiolucency, therefore enabling good postoperative imaging and, particularly, assessment of fusion. Better fusion rates reported in the literature with PEEK interbody spacers may in fact correspond to better visualization in this patient population.
Regardless of the material used, compatibility of the implanted components is essential. All metal implants should be made of the same material. This eliminates the theoretic possibility of internal current generation that may cause corrosion. The size of implanted components should also be compatible. Fixators at the implant-bone junction should be of appropriate diameter, length, and configuration to match the longitudinal member.
The integrity of the patient’s native bone is an important factor. Bone quality can have an impact on construct selection, the biomechanical stability of a construct, and the need for postoperative external immobilization. Osteoporosis is detrimental to all forms of spinal fixation. It influences systems that rely on screw fixation most substantially. Hooks and sublaminar wires are less prone to pullout than screws, and thus they may be more suited for use in the osteoporotic patient. Poor bone quality may necessitate incorporation of additional levels into a construct to promote load sharing and enhance stability but may be difficult to assess. A general impression of bone mineralization may be gleaned from plain cervical radiographs. Dual-energy x-ray absorptiometry and quantitative CT provide an objective determination of bone mineral density. The clinical use of this technology is limited by the lack of cervical spine standards available for comparison. Also, the influence of bone mineral density on screw fixation biomechanics is poorly understood. Currently it is not possible to predict the holding strength of fixators at the implant-bone junction from preoperative studies.
Construct Application
Cervical spine integrity may be restored by either ventral or dorsal stabilization techniques. The application of both may be indicated in cases of severe instability creating a “360 degree” construct. The rationale for selecting one approach over another is case dependent and relies on the degree and extent of instability. If the site of major instability is ventral, a ventral construct should be created to restore structural integrity to the ventral spine. Dorsal instability is treated most effectively through dorsal stabilization. This general rule is valid for all causes of cervical instability. The underlying disease process does influence the selection of specific construct components and the method by which they are applied.
Neural compression often accompanies cervical instability and must be alleviated before stabilization. Neurologic deficit may result from direct neural compression by the disease process itself or by attendant spinal instability. The requirements of neural element decompression in the cervical spine influence the approach that is selected for stabilization; as a general rule, the approach should match the site of worst compression (anterior or posterior), but several exceptions exist. For example, extensive ossification of the posterior longitudinal ligament (OPLL) may result in excessive morbidity when approached anteriorly and thus is frequently treated through an indirect, posterior approach. Posterior decompression, however, is ineffective in the setting of cervical kyphosis and its application may necessitate correction of the deformity either in the same setting or through a separate anterior approach.
The surgeon must be wary and avoid exacerbation of neural compromise by the process of spinal stabilization. For example, dorsal tension-band fixation may increase ventral neural compression resulting from traumatic intervertebral disc herniation or neoplastic disease. This may produce additional neurologic deficit. Constructs must be designed with consideration for the structural alterations that they may induce and the effect that this may have on the neural elements. If this is not appreciated, disastrous consequences may follow.
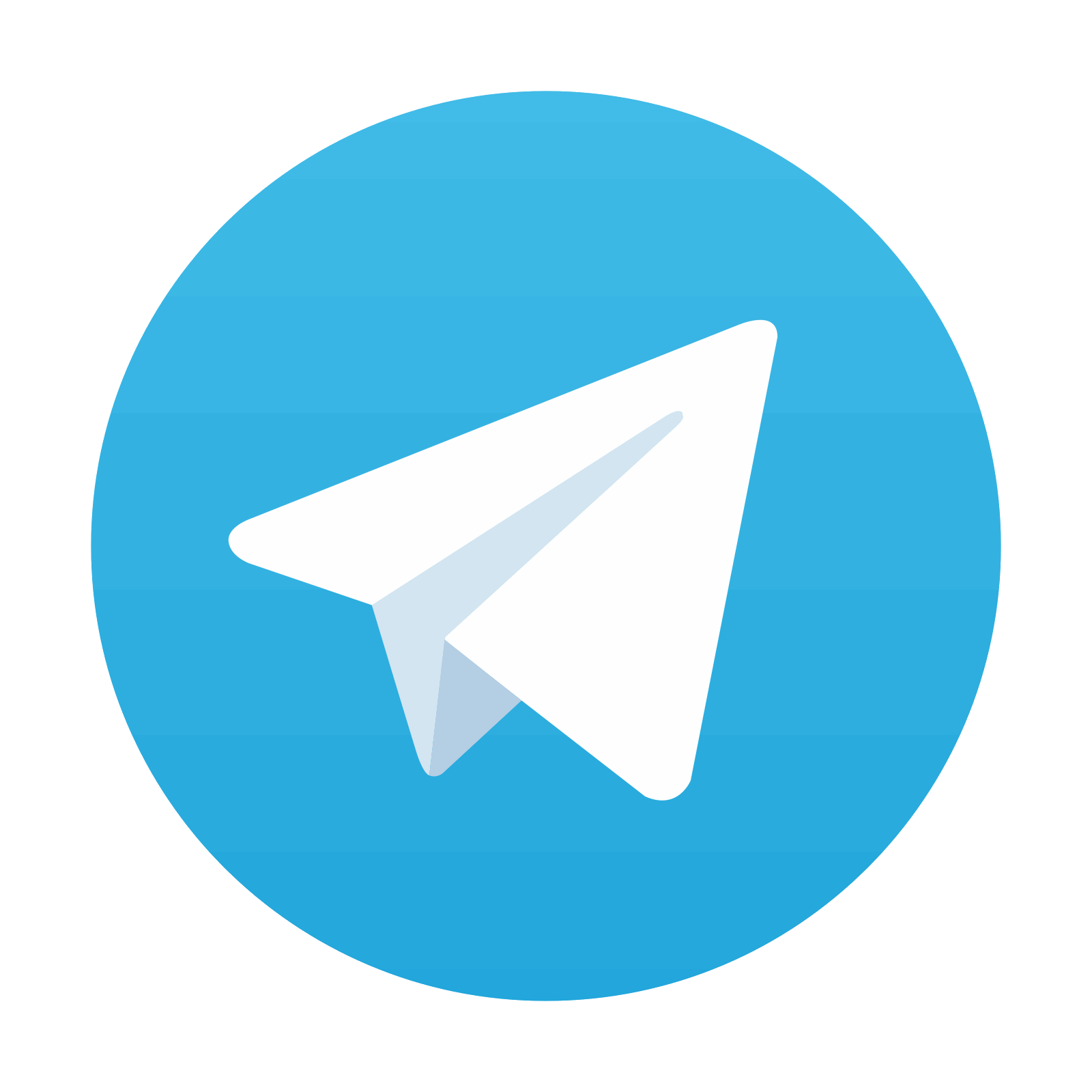
Stay updated, free articles. Join our Telegram channel

Full access? Get Clinical Tree
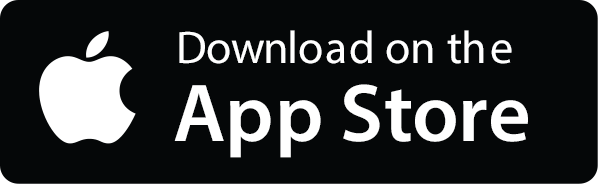
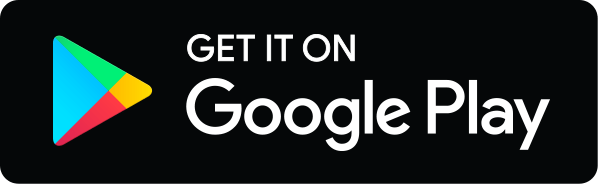