Abstract
Neuroimaging with positron emission tomography is increasingly developing radioligands that emulate targets traditionally within the domain of postmortem studies. Consequently, markers indicative of neuroinflammatory processes are advancing and enabling rapid assessment of the neuroinflammatory theory of major depressive disorder (MDD). One marker, translocator protein (TSPO), has now had a significant number of investigations in MDD and it is anticipated that investigations of other neuroinflammatory markers will be extended into mood disorders. Of these measures, neuroimaging of monoamine oxidase B demonstrates promising differences between MDD and health.
10.1 Introduction
Neuroimaging with positron emission tomography is increasingly developing radioligands that emulate targets traditionally within the domain of postmortem studies. Consequently, markers indicative of neuroinflammatory processes are advancing and enabling rapid assessment of the neuroinflammatory theory of major depressive disorder (MDD). One marker, translocator protein (TSPO), has now had a significant number of investigations in MDD and it is anticipated that investigations of other neuroinflammatory markers will be extended into mood disorders. Of these measures, neuroimaging of monoamine oxidase B demonstrates promising differences between MDD and health. These investigations offer novel potential to stratify MDD by abnormality of neuroinflammatory markers, investigate neuroprogression and assess effects of therapeutics on neuroinflammation in clinical settings.
10.2 Rationale for Neuroimaging Inflammation in Mood Disorders
A strong argument for neuroinflammation to be present in mood disorders is that greater peripheral inflammation is often associated with symptoms found in mood disorders. For example, by the early 2010s, the majority of investigations of peripheral inflammatory markers reported greater plasma levels of the cytokines IL-6 and TNF-α, and to some extent C-reactive protein during MDD, indicating that excessive peripheral inflammation occurs in at least a subset of MDD (1, 2). Another example is that increasing peripheral inflammation may induce depressive symptoms: Twenty to fifty percent of people receiving chronic interferon (IFN-α) treatment for infections or cancer have major depressive episode (MDE), anxiety, and anorexia (3, 4). Similiarly IFN-α treatment is also associated with depressive behaviors in rodents. Also more broadly, inducing peripheral inflammation (e.g., via vaccinations or lipopolysaccharide administration) elicits depression, anxiety, and decreased food intake in humans (5, 6) and rodents (7, 8). While these examples are compelling, several limitations should be mentioned, including that investigations of peripheral inflammation in MDE during 2000–2010 often did not control for body mass index (1, 2), with some investigations comparing MDE with high BMI to healthy with normative BMI; and that it is not established in humans that peripheral inflammation is fully predictive of brain inflammation.
Even so, it is notable that diseases associated with bodily and brain inflammation are associated with particularly high prevalence of mood disorders. Inflammatory bowel disease, a disease with well-established robust peripheral inflammation, is associated with three to five times the usual prevalence of mood disorders. Traumatic brain injury (TBI), which induces brain inflammation (9–12), is frequently associated with depressed mood (13) with 30–50% prevalence of MDE in the first year after TBI (14, 15). Lifetime rates of MDE in systemic lupus erythematosis and multiple sclerosis, illnesses with brain inflammation, are about 50%, which is, again, much higher than the general population (16–21).
The alternative to neuroimaging inflammation is to investigate with postmortem studies and while each offer complementary information, it is more difficult to recruit mood disorder subjects for postmortem studies than for imaging studies. Consistent with this, the majority of studies of inflammatory markers in the brain of mood disorders are either underpowered for moderate effect sizes in mood disorders or they focused on suicide instead. In a sample of twenty-four teenaged suicide victims (seven with MDE) and twenty-four controls, Pandey et al. (22) reported greater levels of IL-6, TNF-α, and IL-1β in the prefrontal cortex. In a postmortem microarray study of the prefrontal cortex in fourteen medication-free MDE subjects and fourteen healthy, Shelton et al. found increased transcription of cytokines that influence inflammation (23). The study of Shelton et al. is suggestive rather than definitive since the transcripts detected reflected both stimulatory and inhibitory cytokines. Even so, this was interpreted as reflecting increased inflammatory stress in the prefrontal cortex (23). Another postmortem study applied immunohistochemical staining with HLA-DR, a marker of microglial activation in a sample of nine subjects with a MDE secondary to MDD, five subjects with a MDE secondary to bipolar disorder, sixteen subjects with schizophrenia, and ten healthy. While this study was underpowered to detect an effect of MDE diagnosis, there was increased HLA-DR staining in the prefrontal cortex in those who died of suicide (24). More recently in 2018, in a comparison of twenty-three MDD subjects and twenty-three controls, within the hippocampus, the region sampled, Mahajan et al. reported differential expression of several genes involved in inflammation including upregulation of CCL2/MCP-1 and downregulation of ISG15, IFI44L, IFI6, NR4A1/Nur-77 and GABBR1, further supporting evidence of neuroinflammatory changes (25).
10.3 Translocator Protein Imaging
Translocator protein imaging is mainly associated with microglia in inflammation. Five to ten percent of brain cells are microglia that are important for early immune responses in the central nervous system where they detect stimuli such as damage-associated molecular patterns, pathogen-associated molecular patterns, and cytokines (26). In response to these stimuli, microglia become activated, changing their morphology and function. Morphologically, their dendrites alter from being longer and more slender to being thickened, shorter, and their cell body volume becomes larger, and sometimes they become ameboid (27, 28). When activated, microglial function shifts from a detection state into a response state (26, 29). This response state may vary depending on local conditions and includes roles also implicated in fostering chronic pathological changes such as increased secretion of IL1β, TNFα, IL-6, prostaglandin E2; diverting tryptophan metabolism toward kyurenine production and away from serotonin synthesis through overexpression of 2,3 indoleamine dioxygenase; and greater production of hydrogen peroxide (30). The response state may also include roles attributed to neuroprotective functions like secretion of IL-4, IL-13, IL-10, and TGFα, and phagocytosis of debris (31).
When microglia become activated, they overexpress TSPO, hence TSPO binding represents an important marker of neuroinflammation (32). There has been some debate regarding whether in pathological conditions, elevated TSPO level represent activated microglia versus astroglia. However, empirically, after exposure to stroke, toxins, and lipopolysaccharide administration, the timing of TSPO elevation closely matches the timing of elevations in markers associated with microglial activation but not such changes in markers associated with astroglial activation (33–35). In pathological conditions, it is believed that TSPO in microglia are the main contributors to the overall TSPO binding, although in healthy conditions, selective knockout of TSPO suggests that binding of TSPO to endothelial cells creates a low baseline signal (36). The question of whether TSPO labels human (versus rodent) microglia has also been raised but it has been demonstrated in postmortem human brain with human immunodeficiency virus that activated human microglia are labeled with TSPO (37).
In the mid 1990s the only high-affinity PET radioligand for the translocator protein was [11C](R)PK11195; however, formal modeling quantification in humans took place later in 2006 (38), which demonstrated, despite a number of previous studies applying reference tissue approaches, that the optimal modeling method was a two-tissue compartment and arterial sampling. In general, reference tissue approaches have been questioned for TSPO imaging, since no brain region with free and non-displaceable binding characteristics representative of gray matter have been shown to be substantially devoid of TSPO receptors. In the mid to late 2000s, a new generation of TSPO binding radiotracers emerged which show greatly improved specific binding to free and non-displaceable binding. There are a number of such radiotracers and a representative sample includes [11C]PBR28, [18F]FEPPA, [18F]PBR111, [18F]DPA714, and [11C]ER176 (see Table 10.1).
Table 10.1 Comparison of PET radiotracers for TSPO
[11C](R) -PK11195 | [11C] PBR28 | [18F]FEPPA | [18F]PBR111 | [18F] DPA714 | [11C]ER176 | |
---|---|---|---|---|---|---|
Selectivity | High (43) | High (39) | Excellent (40) | High (44) | Yes (45) | Yes (46) |
Reversibility | Very good (38) | Very good (47) | Good in gray matter (48) | Excellent (49) | Excellent | Yes (41) |
Brain uptake | good (50) | Very good (47) | Excellent (48) | Very good (49) | Excellent (51) | Very good (41) |
Modeling | Two-tissue compartment (38) | Two-tissue compartment (47) | Two-tissue compartment (48) | Two-tissue compartment (49) | Two-tissue compartment (41) | |
Specific binding to free and non-specific binding ratio |
| Very good (47) | High (48) | Very good (49) | Very good (51) | Very good (41) |
Reliability | Good for whole brain; poor for regions (50) | Yes in gray matter (52) | Good (personal communication) | Not yet published | Not yet published | Not yet published |
Brain-penetrant radioactive metabolites | Unlikely (53) | Negligible to low (39, 40) | Negligible (40) | Negligible (44) | Low (54) | Not yet published |
Measureable in diverse regions | Reliability best for whole-brain regions (50) | Yes | Yes | Yes | Yes | Yes (41) |
While Table 10.1 identifies key issues, there are additional advantages and disadvantages for each. [11C]PBR28 is probably the most widely applied technique in neuropsychiatry but has slightly less stable VT values over the PET scanning period in humans and there is some, but not completely consistent, report of radioactive, brain-penetrant metabolites in rodent brain (39, 40). The design of [18F]PBR111 trades off some of its ratio of specific binding to free and non-displaceable binding for excellent reversibility of its time activity curve, a particular advantage for imaging white matter; however, it has a lesser number of quantifiable regions due to binding of radioactive metabolite to bone. [11C]ER176 is at an earlier stage of development being newer. It is interesting insofar as its distribution volume measure is much less affected by the homozygous state of single nucleotide polymorphism rs6971(41), found in 1% to 10% of subjects, depending on their ethnicity (for other second-generation radioligands, subjects who are homozygous for this genotype are typically excluded from applied neuroimaging studies). This polymorphism causes a single amino acid substitution that reduces the binding of TSPO to all second-generation radioligands. While reliability of TSPO VT for most ligands has not been formally reported, it is common for such data to remain unpublished during the first several years of radiotracer application. There are some reports that for a subset of these radiotracers such as [11C]PBR28 and [18F]DPA714, there is an additional irreversible compartment that improves fitting (42).
TSPO PET imaging has been applied to discover that microglial activation occurs during MDE. In a [18F]FEPPA PET study of twenty unmedicated MDE with no active comorbid psychiatric illnesses and twenty controls, the translocator protein specific distribution volume (TSPO VT), an index of TSPO density, was significantly elevated in the primary regions of the prefrontal cortex, anterior cingulate cortex, and insular cortex by a substantial magnitude of 30%(56) (see Figure 10.1).
Figure 10.1 Elevated translocator protein density (TSPO VT) during a major depressive episode (MDE) secondary to major depressive disorder (MDD). TSPO VT was significantly greater in MDE of MDD (depressed, N = 20, 15 HAB, 5 MAB) compared to controls (healthy, N = 20, 14 HAB, 6 MAB). All second-generation TSPO radioligands, such as [18F]FEPPA, show differential binding according to the SNP rs6971 of the TSPO gene resulting in high-affinity binders (HAB) and mixed-affinity binders (MAB). Red bars indicate means in each group.
Elevated TSPO VT may occur during several neuroinflammatory changes including microglial activation, astroglial activation, and activation of peripheral macrophages. However, in brain tissue greater TSPO level is best interpreted as reflecting greater levels of microglial activation because the increased TSPO expression in mammalian brain after diverse paradigms like stroke, neurotoxins, and lipopolysaccharide administration (27, 28) has a temporal course that closely matches the increased expression of other markers of microglial activation rather than astroglial activation, and peripheral macrophages are typically of low density in human brain. Neuroinflammation, and in particular microglial activation, is a well-established quantitative response to brain injury in neurodegenerative conditions. In addition, induction of microglial activation is implicated in the generation of depressive behaviors in humans and rodents through mechanisms such as the diversion of tryptophan metabolism to kynurenine, stimulation of the hypothalamic–pituitary–adrenal axis and glucocorticoid receptor resistance (29). Thus, given that microglial activation is a marker of advancing disease and is implicated in depressive symptoms it may be advantageous to clinically investigate and categorize chronologically advanced MDD differently.
This has since been demonstrated to be a highly replicable finding, arguably now one of the most replicated findings in mood disorders research (see Table 10.2). Typically across groups, there is 25% to 35% greater TSPO binding in gray matter regions across the brain including prefrontal cortex, anterior cingulate cortex, and insula. However, to date, there have not been studies of TSPO imaging in bipolar disorder or dysthymia.
Table 10.2 Comparisons of translocator protein binding between currently depressed and healthy subjects
Study | Diagnosis and number of subjects | Radiotracer | Region | Results |
---|---|---|---|---|
Setiawan et al. (56) | 20 Medication-free MDE, MDD, 20 healthy | [18F]FEPPA | PFC, ACC, insula, 14 gray matter regions | 30% elevated in MDE |
Li et al. (57) | 40 Medication-naive MDE, MDD, 20 healthy | [18F]FEPPA | Gray matter, white matter, Frontal Cortex, Temporal Cortex, Hippocampus | ~25 to 35% elevated in MDE |
Holmes et al. (58) | 14 Medication-free MDE, MDD, 13 healthy | [11C](R)PK11195 | PFC, ACC, insula |
|
Setiawan et al. (59) | 50 MDE (30 new treatment-resistant MDE + previous 20 MDE), 30 healthy | [18F]FEPPA | PFC, ACC, insula, 14 Gray matter regions | ~ 35% elevated in MDE with long history of being untreated |
Richards et al. (60) | 28 MDE (16 medicated; 12 unmedicated), 20 healthy | [11C]PBR28 | sgPFC, ACC | ~ 25% elevated in sgPFC~15% elevated in ACC |
Li et al. (61) |
| [18F]FEPPA | Gray matter, white matter, frontal cortex, temporal cortex, hippocampus | ~25% elevated in MDE |
Elevated TSPO binding also has major implications for neuroprogression, which may be defined as a pathological reorganization of the central nervous system (CNS) along the course of severe mental disorders (4). Chronic microglial activation may become neuroprogressive consequent to its role in responding to accumulating tissue damage and the inherent feed-forward mechanisms from this process (10, 14, 15, 59). Some of these feed-forward mechanisms stem from production of cytokines, complement proteins, reactive oxygen species, and proteinases. The production of cytokines may induce autocrine effects to produce and maintain more activated microglia whereas the latter three processes may lead to cascades of neuronal damage and additional inflammation (15). For example, neuronal damage as secondary to exposure to reactive oxygen species and proteinases may induce microglial activation through microglial detection of damage-associated molecular patterns with upregulation of toll-like receptors (TLR) such as TLR3 and TLR4 receptors, the latter was found in a sample of dorsolateral prefrontal cortex of MDD subjects (16).
In contrast to neuropsychiatric illnesses like Parkinson’s disease and Alzheimer’s disease in which neuroprogression is firmly established at a neuropathological level, neuroprogression is not well established in MDD, with few investigations demonstrating greater levels of pathology with longer duration of illness (59). While many pathologies have been proposed to be neuroprogressive in MDD, including loss of astroglia and resultant reduced glutamate uptake (5), loss of somatostatin-positive interneurons (6), decreased neurogenesis (7) persistence of elevated MAO-A level (8), hippocampal volume loss (9), and chronic microglial activation (10–12), only hippocampal volume loss has been empirically shown to have greater magnitude of effect with greater duration of MDD, mainly through cross sectional study (9, 13), with mean reductions of 4% overall (9, 13).
Setiawan et al. discovered greater TSPO VT in the gray matter regions sampled were associated with greater duration of untreated MDD. MDE subjects with history of no antidepressant treatment for ten years or more had 31% to 39% greater TSPO VT across gray matter regions as compared to healthy subjects, and approximately 30% greater TSPO VT across these same regions as compared to MDE subjects with short durations of untreated illness (see Figure 10.2 and 10.3). A compelling issue is that there is great potential for pathologically staging MDD, given the increase in TSPO VT of 14% to 18% per decade. This suggests that duration of untreated MDD may reflect a more precise measure of neuroprogression given its relationship with elevated TSPO VT, as compared to the current clinical definition of differentiating between single- and multiple-episode MDD.
Figure 10.2 Relationship between regional translocator protein distribution volume and duration of untreated major depressive disorder
Analyses of covariance (ANCOVAs) with regional translocator protein distribution volume (TSPO VT) values as the dependent variable found that the combination of duration of untreated illness and rs6971 genotype, these predictor variables accounted for approximately 50% of the variance in the three prioritized regions. All second-generation TSPO radioligands, such as [18F]FEPPA, show differential binding according to the single-nucleotide polymorphism rs6971 of the TSPO gene resulting in high-affinity binders (HAB) and mixed-affinity binders (MAB).
Figure 10.3 Translocator protein density greater with more years of untreated MDD compared to short duration of untreated MDD and healthy controls.
Group (duration untreated ≥10 years (N = 25), duration untreated <10 years (N = 25), and healthy (N = 30)) and genotype were significant predictor variables in a multivariate analysis of variance with TSPO VT. Additional comparisons based on the least significant difference test showed significant differences between a long duration of untreated MDD as compared to healthy controls and significant differences between long duration of untreated MDD and short duration of untreated MDD in all of these regions. Second-generation TSPO radioligands, such as [18F]FEPPA, show differential binding according to the single-nucleotide polymorphism rs6971 of the TSPO gene resulting in high-affinity binders (HAB) and mixed-affinity binders (MAB). Red bars indicate means in each group.
The second main finding was that total duration of illness predicted greater TSPO VT and duration of antidepressant exposure was a similar magnitude negative predictor of TSPO VT throughout the gray matter regions sampled. The yearly increase of TSPO VT is no longer present when antidepressant treatment occurs since duration of antidepressant exposure is a negative predictor in the model when total duration of MDD is included, and these predictors are of similar magnitude but in the opposite direction. Since most of the antidepressant exposure was selective serotonin reuptake inhibitors or selective serotonin and norepinephrine reuptake inhibitors, this provides a context for interpreting literature reports that serotonin reuptake inhibitors reduce induction of microglial activation in cell culture in regards to the brain during clinical treatment in humans (20, 21). The in vitro findings could have been interpreted to indicate that short durations of serotonin reuptake inhibitor treatment strongly reduce microglial activation, but the data of this cross sectional study argue that the yearly accumulation of greater TSPO VT merely stops. Since inducing microglial activation is associated with concurrent onset of depressed mood and behaviors (29, 30), it may be therapeutically useful to develop superior strategies to modulate and/or reduce microglial activation such as via minocycline administration. Some positive preliminary results of add-on minocycline were shown in a randomized double-blind placebo-controlled trial of MDD (31).
There are several other studies that probed the relationship of TSPO binding to clinical features of MDE. Richards et al. (60) found that medication-free subjects who also had long histories of MDD have higher levels of TSPO binding. Holmes et al. (58) reported that the presence of current suicidal ideation was associated with greater TSPO binding in the ACC and Li et al. (61) reported that poorer attention performance was associated with greater prefrontal cortex TSPO VT.
10.4 Novel PET Imaging Probes of Neuroinflammation
In the postmortem field it is common to apply multiple markers to characterize microglial and astroglial activation, whereas this is impractical with PET when arterial sampling is required. However, there will be increasingly greater opportunity to investigate other markers of neuroinflammation in MDE (see Table 10.3). Challenges with these markers are that they are often not specific to individual cell types, and that many of the radiotracers listed are in development and not yet established for use in humans.
Table 10.3 PET imaging of neuroinflammation: molecular targets and potential radiotracers
Molecular target | Cellular expression | Expression in neuroinflammation | Radiotracers investigated |
---|---|---|---|
TSPO | Microglia and astrocytes | Upregulated | [11 C]PK11195, [18 F]FEPPA, [18 F]PBR06, [18 F]FEDAA1106, [11 C]PBR28, [11 C]ER176, [18 F]DPA-714 |
GSK-3 | Microglia and astrocytes | Upregulated | [11 C]PF-367, [11 C]SB-216763, |
MAO-B | Astrocytes and 5-HT releasing neurons | Upregulated | [11 C]SL25.1188, [11 C]-L-deprenyl, [11 C]-L-deprenyl-D2, [18 F]fluorodeprenyl-D2, [18 F]fluororasagiline-D2, |
ROS | Microglia | Upregulated | [11 C]hydromethidine, [11 C]1, [18 F]ROStrace, [11 C]ascorbic acid |
I2BS | Astrocytes | Upregulated | [11 C]BU99008 |
COX-1 | Microglia | Upregulated | [11 C]Ketoprofen-methyl ester, [11 C]PS13, [18 F]PS2 |
COX-2 | Microglia | Upregulated | [11 C]MC1 |
Arachidonic acid | Microglia and astrocytes | Upregulated | [11 C]Arachidonic acid |
S1P1 | Microglia ≫ astrocytes | Upregulated | [18 F](R)-1-[[3-(6-fluorohexyl)-phenyl]amino-4-oxobutyl]phosphonic acid, [11 C]TZ3321, [18 F]TZ35110, [18 F]TZ43113, [18 F]TZ35104, [18 F]TZ4877, [18 F]TZ4881 |
CB2 | Microglia and astrocytes | Downregulated | [11 C]NE40, [11 C]MA2, [18 F]MA3 |
Purinergic receptor: P2X7 | Microglia ≫ astrocytes | Upregulated | [11 C]A-740003, [11 C]SMW139, [11 C]JNJ-54173717, [11 C]GSK1482160 |
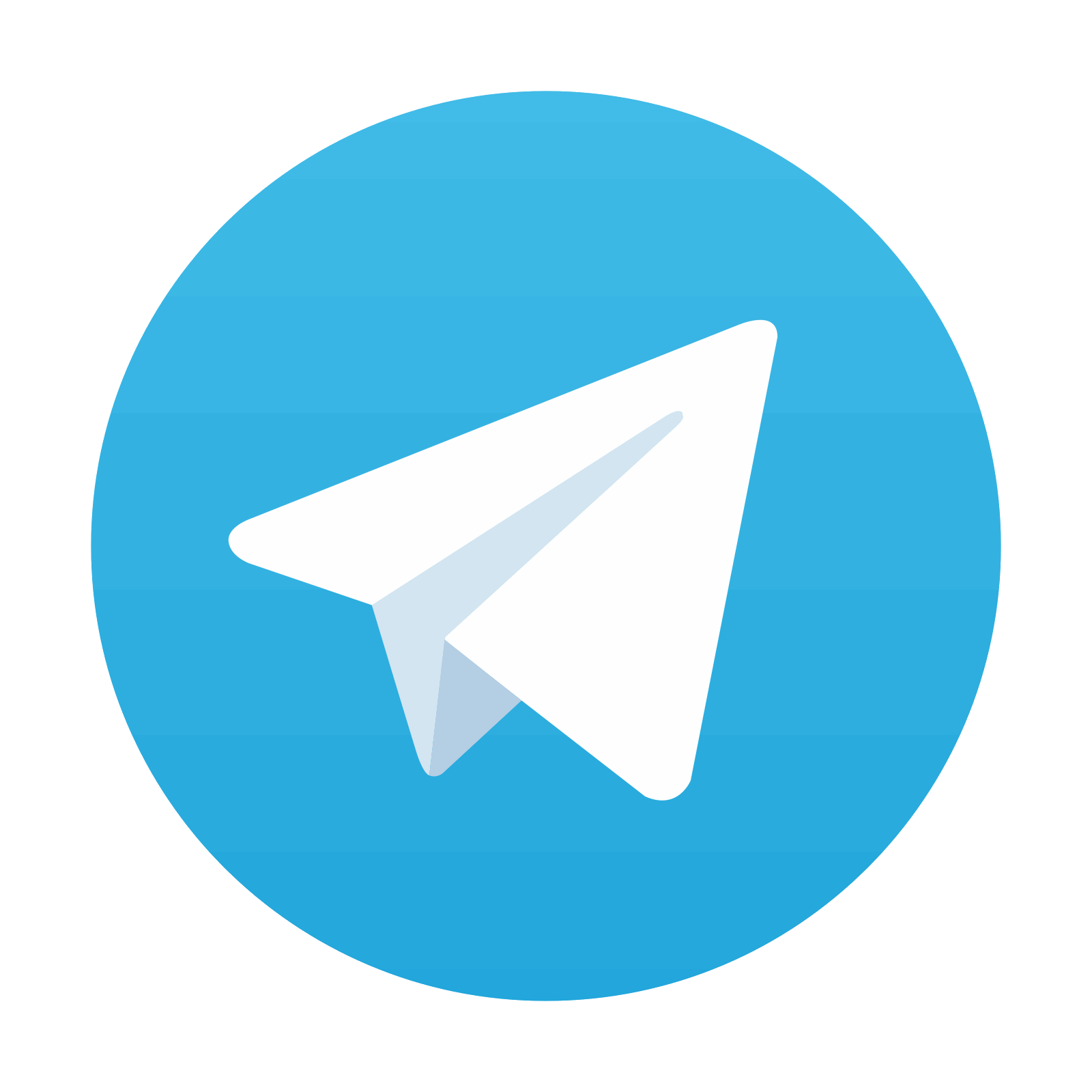
Stay updated, free articles. Join our Telegram channel

Full access? Get Clinical Tree
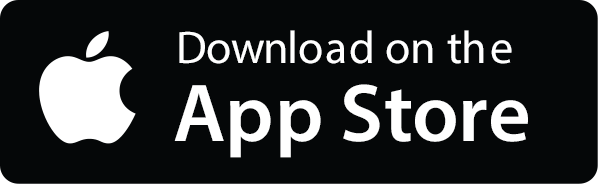
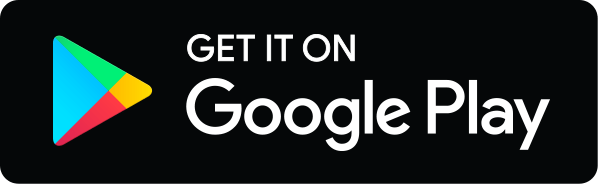
