Abstract
Genetics explain 60–85% and 31–50% of the risk to develop, respectively, bipolar disorder (BD) (1, 2) and major depressive disorder (MDD) (3, 4). Thus, hereditability has emerged as a crucial factor in the pathophysiology of mood disorders. Early genetics studies focused their attention on specific single-nucleotide polymorphism (SNP), preselected on prior evidence for their functional role in coding products that may influence relevant features of the disorders, also known as candidate gene approach. In these studies, we define risk allele, genetic variants associated with the disorder or worse clinical features such as reduced response to therapeutics, early onset, and higher recurrence.
Genetics explain 60–85% and 31–50% of the risk to develop, respectively, bipolar disorder (BD) (1, 2) and major depressive disorder (MDD) (3, 4). Thus, hereditability has emerged as a crucial factor in the pathophysiology of mood disorders. Early genetics studies focused their attention on specific single-nucleotide polymorphism (SNP), preselected on prior evidence for their functional role in coding products that may influence relevant features of the disorders, also known as candidate gene approach. In these studies, we define risk allele, genetic variants associated with the disorder or worse clinical features such as reduced response to therapeutics, early onset, and higher recurrence. In recent years thanks to the substantial advance in genetic technology, the new cost-effective microarray procedures implemented in genome-wide association studies (GWAS), the list of risk genes associated with mood disorders has rapidly increased (5). However, genetic data explain only a small portion of phenotypical variance of mood disorders (6). In order to fill the gap between genetic asset and phenotype, the focus moved to endophenotypes integrating genetic and neuroimaging data in an imaging genetic perspective (7). This conceptual framework identifies brain function and structure as “intermediate phenotypes” between the genetic vulnerability, such as risk allele, and the disorder, closer to biological pathways than phenotype itself, and thus widely affected by genetic risk variations (8). Insights from meta-analyses and research studies confirm also the hereditability of neuroimaging abnormalities in BD and MDD, as detected in high genetic risk subjects and first-degree relatives of affected patients (9–13).
Parallel to the quest for intermediate phenotypes, a new approach has emerged, which views mood disorders as the result of the modulation of gene expression by environmental stimuli (14) through epigenetic mechanisms. Consequently, epigenetic has also been studied within the imaging genetic approach.
This chapter will address the issue of the identification of imaging genetic and epigenetic biomarkers in MDD and BD, focusing mainly on studies investigating white matter (WM), gray matter (GM), and functional magnetic resonance imaging (fMRI).
11.1 Genome-Wide Association Studies
Recent genetics studies have begun to adopt GWA methods identifying several independent single-nucleotide polymorphisms (SNPs) significantly associated with diagnosis of MDD: OLFM4, TMEM161B-MEF2C, MEIS2-TMCO5A, NEGR1, RSRC1-MLF1, L3MBTL2, VRK2, FHIT, RBFOX1, SORCS3, HACE1-LIN28B, KIAA0020-RFX3, PAX5, RERE, BICC1, PCLO, NCAN, and NETRIN1 (15–17); and BD: TRANK1, ANK3, and ODZ4 genes (18). Only one SNP in the calcium voltage‐gated channel subunit alpha1C (CACNA1C) gene associated with both MDD and BD. Neuroimaging studies explored the effect of the emerged loci from GWAS on both functional and structural brain data in order to elucidate their role in MDD and BD pathophysiology.
Studies in MDD showed that the piccolo presynaptic cytomatrix protein (PCLO), which is involved in establishing active synaptic zones, in synaptic vesicle trafficking, and in monoaminergic neurotransmission, is altered in MDD (19) and is associated with antidepressant treatment response (20). The rs2522833 was associated with a decreased GM in the left temporal pole in drug-naive, first-episode MDD patients carrying C allele (21), whereas A/A, linked to an increased risk of depression, associated with higher GM hippocampal volume and reduced WM lesion volume in patients with late-life MDD (22). fMRI studies showed increased activation in the left amygdala in response to emotional negative stimuli, but no altered prefrontal recruitment on executive function task (23). PCLO*C carriers compared to non-risk ‘A’ allele carriers showed reduced insula and a trend-wise of anterior cingulate cortex (ACC) and inferior frontal gyrus activation during emotional memory processing (24), and worse memory performance and lower encoding-related hippocampal activation (25). Larger hippocampal volumes (26), only in the absence of early-life adversities (27), and increased activation in brain regions involved in emotion processing have been reported in individuals with the T allele of Bicaudal C homologue 1 (BICC1) gene (27), which has an important role in neuroplasticity. A similar role is played by the NCAN gene, involved in cell adhesion and migration and neurite growth, that impact on subcortical brain structure in healthy and depressed subjects (28). Finally, attention has to be given to (a) the arginine–glutamic acid dipeptide (RE) repeats (RERE) gene, important for normal brain development (29) that was associated with WM alterations in first-episode and drug-naive MDD patients (30); (b) Tescalin gene, involved in neuronal proliferation and differentiation, associated with both WM and GM changes in MDD (31); (c) LHPP gene that encodes an enzyme known as phospholysine phosphohistidine inorganic pyrophosphate phosphatase and influences brain activity in MDD (32).
Studies in BD showed a role of ANK3 (loci rs10761482, rs10994336, and rs9804190), a gene coding for Ankyrin G, a protein involved in voltage gating in neurotransmission, in neurogenesis and in myelination. Evidences in BD suggest an effect of ANK 3 genes on WM integrity: BD carriers of the risk allele of rs10761482 showed a lower fractional anisotropy (FA), a measure of WM microstructure, in the forceps (33), while rs9804190 risk allele with reduced FA in the uncinate fasciculus and the cingulate gyrus (34). When performing neuropsychological test (N-back test), the rs10994336 SNP risk was associated with hyperactivation of the anterior and posterior cingulate cortex (35). No effect on brain volume and cortical thickness was observed (36). Another SNP involved in neurotransmission is Neuregulin 1 (NRG1) rs35753505, which affects myelin, neural, and glial cellular growth, differentiation, and death (37). The risk C allele was associated with greater WM volume in the cingulum/parahippocampal gyrus and the callosal body (38) and increased functional response in orbitofrontal cortex (39).
Among the genes related to both MDD and BD, the CACNA1 C gene regulates the L-type voltage-dependent calcium channel 1C subunit, which is involved in mechanisms of neuronal plasticity also indirectly affecting genetic transcription, neuronal signaling, and excitability (40). The rs1006737*A risk allele, possibly linked to a decreased expression of CACNA1C, has been related to MDD and BD (18, 41) with altered functioning of brain regions that have been related to mood disorders (42, 43). In MDD, the A allele associated with an increased activation in the left inferior frontal gyrus and cerebellar areas (44), whereas in BD was associated with greater orbitofrontal thickness (45), reduced GM volume in left putamen, and increased volume in the right amygdala and right hypothalamus (46). Thickness in caudal portion of ACC was also negatively correlated with age only in BD carriers of the risk allele (45). During emotional processing of negative faces, the risk allele associated with higher activation of amygdalae, and lower of ventrolateral prefrontal cortex (47–49). Higher hippocampal functional response has also been detected during emotional tasks (50, 51). During a verbal fluency task, CACNA1C risk allele associated with increased activation in the bilateral prefrontal‐temporal and occipital cortex and thalamus (52). Other studies, however, failed in highlighting any significant effect of this gene on brain volume, thickness, WM integrity, and function (36, 53–56).
GWAS studies suggest a detrimental effect of SNPs involved in neurotransmission, neural growth, myelination, and plasticity on brain structure and function both in MDD and BD with a pleiotropic effect on corticolimbic circuitry involved in both affective and cognitive processes, confirming its relevance in mood disorder pathophysiology. It appears that these gene variants can influence brain structure and function, although the mechanism by which this occurs remains to be determined. More research is needed to understand both the function of these genes and their relevance in psychiatric disorders.
11.2 Polygenic Risk Score
Despite these promising results, studying single-risk variants may lead to explain only small effects. In order to evaluate the cumulative effect of multiple risk alleles, a polygenic risk score (PGRS) (also called genetic risk score or genome-wide score) has been developed. PGRS summarises in a single variable the genetic liability to a disorder prompted by GWAS results. For each subject, PGRS is calculated by summing the number of risk alleles, weighted by their effect size emerged from the GWAS study. The role of polygenic risk scoring in mood disorder has been widely investigated (57–59), however, when combining this approach to neuroimaging data in order to identify the influence of genetic factors on brain structure and function, limited and contrasting results emerged.
A higher polygenic risk for MDD and reduced WM integrity and cortical thickness in the medial prefrontal cortex, an area associated with negative affect and poor functioning in social domains, has been highlighted (60, 61). In the only fMRI study performed on MDD-PGRS during a working memory task, a higher PGRS was associated with increased brain activation in right middle frontal gyrus and the right supplementary motor area, whereas a lower PGRS was related to increased activation of the bilateral cerebellum, bilateral middle occipital gyri, bilateral middle frontal gyrus, right precentral gyrus, and left inferior parietal lobule (62).
However, other studies found no evidence for an association between PGRS and either subcortical brain volumes or WM integrity (63, 64) or functional connectivity in MDD (65).
Higher PGRS for BD has been associated with a lower functional response in visual cortex during a face recognition task, whereas signal in ventromedial prefrontal cortex and ACC was higher during working memory, face matching, and verbal fluency tasks (66–68). The volume of amygdala, as well as of globus pallidus, has been previously related to PGRS of BD (69). A resting-state study pointed out significant association between PGRS and functional connectivity between the insula and the bilateral cuneus, precuneus, posterior cingulate, and midbrain (70). These data suggest a significant effect of the genetic liability for BD of brain functional activity and connectivity in both cognitive and affective processing. On the other side, studies focusing on structural imaging mainly failed in highlighting significant associations between PGRS and WM integrity, subcortical and cortical volumes (71–73). However, a GWAS study investigating average FA, as quantitative phenotype in unaffected relatives of patients with BD and a matched healthy control showed significant associations with SNPs involved in cell adhesion, WM development, and neuronal plasticity (e.g., EPS15L1, ADAM7, LPP, HEPACAM, ROBO4), supporting the role of WM microstructure as endophenotype of BD (74).
11.3 Candidate Gene Studies
Other results for imaging genetics come from the candidate gene approach where SNPs are preselected based on prior evidence for their functional role in coding products that influence relevant neural systems or relevant features previously related to the disorders. Accordingly, SNPs have been selected among genes involved in the activity of neurotransmitters such as serotonin (5-HT), dopamine, and glutamate, growth factors, and response to stress.
The most studied neurotransmitter in depression pathophysiology is 5-HT. Changes in 5-HT synthesis, turnover, and receptor density and structure affect the clinical outcome and pathophysiology of MDD and BD (75, 76), and SNPs involved in serotoninergic transmission have been explored in imaging genetic studies. The s variant of an SNP in the promoter region of the serotonin transporter (5-HTTLPR, SLC6A4 gene) is associated with reduced transcriptional activity (77), leading to lower serotonin transporter (5-HTT) expression and 5-HT reuptake. From a clinical perspective, the 5-HTTLPR*s allele is a risk factor for the development of MDD and in BD was associated with an earlier onset (78) and a worse response to antidepressants (79), whereas l/l showed a worse recurrence of mood episodes (78) and increased hopelessness and suicidal ideation (80, 81). In healthy subjects, 5-HTTLPR*s carriers showed increased amygdala reactivity (82, 83); lower amygdala–ACC functional coupling in response to emotional stimuli, with GM volume reductions in both these regions (84); and concurrent variation in emotional processing performances (85). The long allele of the 5-HTTLPR has been associated with decreased hippocampal GM and WM volumes in patients with MDD (86, 87). These results were confirmed when late-onset patients were considered, whereas a significant association between the 5-HTTLPR*s allele and smaller hippocampal volumes was observed in early-onset (88) and drug naive patients (89).
5-HTTLPR*s allele was also associated with smaller caudate nucleus (90), whereas LA/LA homozygotes (5-HTTLPR tri-allelic) associated with larger left thalamus and putamen volumes (91). However, other studies did not find significant genotype interaction in hippocampal volume (92, 93) and/or amygdala volumes (94), or the orbitofrontal cortex (95). fMRI studies reported (a) no association between 5-HTTLPR gene and amygdala activity or connectivity (96); (b) increased amygdala activation in 5-HTT risk allele carriers to masked emotional faces (97); (c) a lower activation of the medial prefrontal cortex and connectivity with amygdala in MDD (98), confirming an alteration in prefrontal-limbic regulation.
In BD, the 5-HTTLPR*s allele correlated to increased amygdala and reduced dorsolateral prefrontal volume, and WM integrity in several tracts (increased radial diffusivity and mean diffusivity in several brain tracts, including corpus callosum, cingulum bundle, uncinate fasciculus, corona radiata, thalamic radiation, inferior and superior longitudinal fasciculus, and inferior fronto-occipital fasciculus), suggesting demyelination or loss of bundle coherence (99). When considering childhood stress, in a GxE model, researchers found that patients carrying the 5-HTTLPR*s allele showed smaller hippocampal volumes when they had a history of emotional neglect (100). An association between 5-HTTLPR*s allele and alterations in microstructural fronto-limbic WM have been recently reported in depressed elderly patients, who also showed a lower remission rate (101). Related to serotoninergic transmission, an imaging genetic study explored effect of 5-HT1A receptor promoter gene polymorphism (rs6295) on functional connectivity during processing of emotional negative stimuli. The risk allele (G/G) associated with worse antidepressant response (102), with a higher risk of committing suicide (103), and a higher coupling between amygdala and ventrolateral prefrontal cortex for emotional stimuli compared to C carriers, also positively associated with depressive symptoms (104).
Another SNP investigated in mood disorder is the rs4680 on the Catechol-O-methyltransferase (COMT) gene. COMT inactivates extraneuronal dopamine in the brain and a valine (Val) to methionine (Met) transition leads to a decrease of enzymatic activity, resulting in an increased dopamine level (105). This genetic variant affects clinical and prognostic features such as response to antidepressant treatment (106, 107), recurrence of manic or psychotic episodes (108, 109), and rapid-cycling variant of BD (110). Structural studies reported controversial results. Val/Val patients with MDD showed a reduction in FA in several fiber tracts compared to Met carriers (111), suggesting a cortico-limbic network dysfunction in MDD. Conversely, a decreased FA in several fiber tracts (112) and smaller bilateral caudate (113) was reported in Met-carrier MDD patients compared to healthy control but not in Val/Val individuals.
An fMRI emotional processing study showed that activation in the inferior frontal gyrus (IFG), correlated with the number of met-alleles in healthy controls but such correlation was not seen in MDD patients. Moreover, during a working memory task, met-allele was associated with lower activation in the middle frontal gyrus (MFG) in both healthy controls and MDD patients (114). In BD, Val allele compared to Met allele, was associated with enhanced reactivity of the amygdala and reduced activity in ventromedial and lateral prefrontal cortex. Val allele was also associated with higher significant positive functional connectivity between amygdala, dorsolateral prefrontal cortex, and supramarginal gyrus, whereas Met carriers presented a significant negative coupling (115) during processing of negative stimuli. During working memory task, Val/Val displayed decreased activity in the dorsolateral prefrontal cortex (116). Data suggest that COMT affects pleiotropically the reactivity to stimuli in the prefrontal cortex and in amygdala in both healthy controls and BD patients.
Another Val/Met transition has been observed in an SNP (rs6265) in the brain-derived neurotrophic factor (BDNF) gene. The BDNF protein is a neurotrophin affecting cerebral plasticity, neural maturation, survival, and differentiation, and the Met allele may result in reduced transport of BDNF mRNA to dendrites and decreased packaging and secretion of BDNF in neuronal cells (117, 118). Several genetic-neuroimaging studies showed a significant genotype–diagnosis interaction with reduced volume in prefrontal cortex (119), anterior cingulate (120), and hippocampus (121) among MDD Met carriers. When accounting for a history of childhood trauma, Met carriers showed significantly smaller hippocampal volume, outlining the importance of a gene–stress interaction (122).
Met allele and lower BDNF serum levels were reported in ACC and caudal brainstem (pons) in depressed subjects. Additionally, lower BDNF levels in ACC were reported in subjects who had been exposed to early-life adversity and/or committed suicide, shedding light on its possible role in the neurobiology of suicide (123).
BDNF also affects WM microstructure, including the corona radiata, uncinate fasciculus, inferior longitudinal fasciculus, cingulum, and corpus callosum (124, 125), and resulted as a moderator of the association between uncinate fasciculus connectivity and antidepressants treatment response (126) in MDD. Moreover, an interaction effect of both BDNF and 5HTTLPR on the left transverse frontopolar volume was also detected, suggesting their important roles in brain regions involved in emotion processing in MDD (125). fMRI studies found decreased bilateral hippocampal functional connectivity with the temporal cortex and dorsal nexus (127), poorer performance at n-back task associated with hippocampal activation (128) in MDD Met carriers. In contrast with previous results, some studies found no evidence or even reversed data (90, 129, 130), thus, implicating the need to further elucidate these associations.
In BD, the Met allele was associated with reduced volume in hippocampus, in anterior cingulate gyrus, in dorsolateral prefrontal cortex (131, 132), with loss of gyrification, and with GM volume in left hemisphere (133, 134). Effect of rs6265 was not confirmed in pediatric BD patients (135). The abnormalities detected in BD have been suggested as neurobiological underpinning of the cognitive impartments detected in the disorder and associated with Met allele (136) and BDNF peripheral serum level proposed as biomarker for the disorder (137).
Among the neurotransmitters involved in mood disorder, glutamate has a crucial role due to its involvement in both neuronal signaling and neurotoxicity. Some neuroimaging studies focused their attention on D-amino acid oxidase activator (DAOA) and SLC6A15 genes, implicated in the glutamate synthesis and dysfunction. The DAOA rs2391191 was associated with altered region of homogeneity in the cerebellum, right middle frontal gyrus, and left middle temporal gyrus in MDD (138). Moreover, MDD patients with a SLC6A15 risk A-allele showed lower FA than controls with the same genotype in the left parahippocampal cingulum, known to be important in emotional processing (139). An abnormal region of homogeneity of the corpus callosum, cingulum and the frontal, parietal, and temporal lobes was associated with SLC6A15 rs1545843 in MDD patients (140).
After its release, glutamate reuptake is performed by excitatory amino acid transporters (EAATs), involved in maintaining physiological levels of glutamate in the brain. Polymorphisms in genes coding for these transporters affected WM, GM, and functional connectivity, interacting with adverse childhood experience. For EAAT1, rs2731880*T allele leads to reduced EAAT1 expression and glutamate uptake and T/T bipolar patients had a higher significant negative connectivity between the amygdala and ACC and performed better in the face-matching task compared to rs2731880*C carriers (141). For EAAT2-181A > C (rs4354668), when exposed to high stress, the carriers of the C allele showed lower axial diffusivity compared to A/A, whereas when exposed to low stress they showed higher axial diffusivity and higher GM volume (142). Excess of free glutamate may then contribute to vulnerability to stress. Within the Homer family of postsynaptic scaffolding proteins, exerting a crucial role in glutamate-mediated synaptic plasticity affecting synaptic homeostasis, neuroplasticity, the AA genotype of the Homer rs7713917 polymorphism, previously related to mood disorders and suicide (143), associated with lower fractional anisotropy in frontal WM tracts, lower GM, and higher fMRI neural responses to emotional stimuli in medial prefrontal cortex (144).
Other genes involved in the circadian rhythm, myelination, and neuroplasticity have been studied as possible biomarkers of mood disorder. Genes involved in the control system of circadian rhythms, related to core features of BD, such as patterns of sleep, rest, and activity, age at onset of illness, and response to antidepressant treatment (145) have been associated with WM microstructure. Period3 (PER3)4/4 homozygotes had increased radial diffusivity and reduced fractional anisotropy, whereas CLOCK 3111 T/C rs1801260*C carriers showed increased mean diffusivity.
The A/A genotype of the transcriptional factor polymorphism of the sterol regulatory element binding protein (SREBF-2, rs1052717), involved in lipid and cholesterol metabolism, associated with increased radial diffusivity and reduced FA in cingulum, corpus callosum, superior and inferior longitudinal fasciculi, and anterior thalamic radiation. These results suggest a role of SREBF-2 in affecting WM integrity possibly related to its effect on myelination processes (146).
Glycogen synthase kinase-3 beta (GSK3β) is involved in the control of gene expression, and affect neurodevelopment and regulation of neuronal polarity, neuronal plasticity, and cell survival (147). Few studies exist in imaging genetics that reported an association of GSK3β rs6438552 and rs12630592 polymorphisms with altered GM volume in the right hippocampus and temporal lobe (148, 149), altered functional brain activity in the thalamus, and parts of the occipital and parietal lobes (150), and an association of GSK3β substrate genes with medial prefrontal cortices (151) in MDD patients.
In BD, a widespread effect on WM integrity and GM volumes was shown in less active glycogen synthase kinase 3-β (GSK3-β) rs334558*C gene-promoter variant. The low-activity C allele was associated with less detrimental clinical features of mood disorders, such as delayed onset and a better clinical response to treatments (152) and higher brain integrity; higher volumes and axial diffusivity were observed in ventral prefrontal cortex and in corpus callosum, forceps major, cingulum bundle, superior and inferior longitudinal fasciculus, fronto-occipital fasciculus, thalamic radiation, corona radiata, and corticospinal tract (153).
Considering the important role that stress seems to play in mood disorder, the glucocorticoid receptor, a mediator of the stress response, has been the focus of several imaging-genetic studies. The gene for the glucocorticoid receptor regulator FK506 binding protein 5 (FKBP5) is a glucocorticoid inducible gene that was found to be associated with response to antidepressants (154) and the recurrence of depressive episodes (155). In fact alterations of the glucocorticoid system have consistently been reported in MDD (156). Results indicate that the additive effect of connectivity alterations of the right hippocampus and the FKBP5 genotype influence depression risk (157). Moreover, the interaction between the high-risk allele and childhood maltreatment associated with WM changes in the insula and inferior frontal gyrus (158). Other studies showed a volume reduction of portions of the frontal and parietal areas (159) and abnormal functional coupling of regions involved in perception, recognition, and attention allocation (160).
Finally, some study focused on late-onset depression and, as it often precedes the onset of dementia, on the APOE ε4 allele, which is known to be a genetic risk factor for Alzheimerʼs disease. Geriatric depressed patients with an ApoE ε4-allele showed alteration in hippocampal morphology (161, 162) that seems to be related to cognitive decline (163), smaller right medial frontal gyrus, left middle frontal gyrus and left inferior occipital gyrus (164), abnormal hippocampal functional connectivity (165), and default mode network connectivity (166). An increase in WM hyperintesities has been reported in patients with ApoE ε4-allele (167) who showed chronic course of MDD, a higher number of depressive episodes, and lower age at onset (168).
Although the mechanisms underlying this relationship remain unclear, geriatric depressive patients with the ApoE ε4-allele may be an early manifestation of the AD.
11.4 Epigenetics
Years of research on the genetic basis of several disorders has made clear that genetics is not enough to explain chronic diseases. Besides the DNA sequence inherited from parent to offspring and identical through life and in all the cells and tissues of our body, other information is present in our genome, which is cell and tissue specific and can be modified by the environment especially in critical periods during development but also later in life. This information is epigenetic and epigenetic modifications enable the regulation of gene expression without altering the sequence of the DNA. The most widely studied epigenetic modification is DNA methylation, that refers to the addition of a methyl group onto the 50 carbon of a cytosine ring by DNA methyltransferases (169). When methylation of the gene is done on the promoter region, it interferes with the binding of transcription factors to the promoter region, thereby inhibiting gene expression (170, 171). In this case, a higher degree of methylation is related to decreased gene expression.
As for genetic studies, the exploration of DNA methylation in mood disorders has focused on genes associated with the activity of neurotransmitters involved in the disorder such as serotonin, dopamine, and glutamate but also on glucocorticoid receptor for its role in the response to stress. Indeed, stress is widely considered one of the major factors responsible for the induction of epigenetic changes in mood disorder (172).
DNA methylation in the SLC6A4 promoter has been associated with history of lifetime depression (173), depression severity (174), antidepressant response (175), 5-HTT mRNA levels (176), childhood maltreatment (177), and acute stress (177), whereas hypermethylation was observed in a monozygotic twin with BD but not in controls (178).
Neuroimaging studies investigated SLC6A4 DNA methylation in association with different brain characteristics as WM microstructure, GM volume, and brain function. Medication-naive MDD patients showed elevated SLC6A4 DNA methylation, measured at five cytosine–guanine (CpG) sites of the promoter region, compared to healthy subject. Furthermore, a greater level of methylation was associated with lower fractional anisotropy and axial diffusivity (179), smaller hippocampal volume (CA1, gyrus dentate, and CA2/3) in patients with MDD, and with treatment with SSRI (180). The study of SLC6A4 methylation in an AluJb element in the promoter, a genomic element able to regulate nearby gene expression (181), showed that lower AluJb methylation was lower in MDD patients and associated with decreased amygdala reactivity during an emotional face-matching task. Furthermore, in subject carriers of the 5-HTTLPR/rs25531 risk allele, an increased bilateral amygdala activation was observed (182).
When considering the COMT gene, MDD subjects showed lower methylation at CpG sites 1–5 compared to healthy controls that were associated with lower fractional anisotropy and higher radial diffusivity in the superior longitudinal fasciculus, inferior longitudinal fasciculus, anterior thalamic radiation, and uncinate fasciculus in MDD, whereas the opposite was observed in healthy controls (183).
Two genes involved in the glucocorticoid response to stress have been investigated in epigenetic studies, the glucocorticoid receptor gene NR3CI and the FKBP5 gene. Decreased methylation of FKBP5 was observed in children and adult victims of childhood trauma (184). Also, in MDD, higher childhood trauma predicted lower FKBP5 intron methylation in the carriers of the FKBP5 rs1360780*T allele (185, 186). Lower DNA methylation of intron 7 associated with reduced cortical thickness of the right transverse frontopolar gyrus in the rs1360780*C allele homozygote group (186), reduced GM concentration, and increased hemodynamic response during an emotional recognition task in the inferior frontal orbital gyrus (185).
Methylation in the promoter region of the glucocorticoid receptor NR3C1 gene has been suggested to mediate glucocorticoid resistance by which HPA axis remains activated even after a stressor has ended. Lower methylation at two CpG sites of the NR3C1 promoter has been reported in MDD and associated with lower volume in the cornu ammonis (CA) 2–3 and CA4-dentate gyrus hippocampal subfields whereas, in healthy controls, lower methylations associated with lower volume in the subiculum and presubiculum (187). Greater methylation of exon1D of the NR3C1 gene, which includes a glucocorticoid response element required for transcription factors to bind and trigger autoregulation of the receptor after cortisol release, was associated with increased familial burden of anxious-depressive disorders and reduced resting-state hippocampal connectivity in a monozygotic twin sample (188).
Methylation at the BDNF promoter region has been associated with MDD (189), antidepressant treatment response (190), and suicide (191). Furthermore, in animal models, this methylation associates with BDNF gene expression in neuronal cells (192), suggesting that in regions with greater methylation this might lead to a decreased BDNF release. Indeed, greater BDNF DNA methylation at four CpG sites of the promoter region associates with lower fractional anisotropy in the right anterior corona radiata in MDD (193).
Involved in neuronal proliferation and differentiation, TESC rs7294919 methylation in the CpG pos 2 and pos 3 was greater in MDD, and greater DNA methylation of CpG 3 associated with lower FA and higher RD of the right parahippocampal cingulum (194).
In conclusion, factors affecting core psychopathological features of mood disorders, course and outcome of the illness, and the risk to develop the disorder, do influence brain structure and function, as studied with multimodal brain imaging. In turn, structure and function of the brain, as influenced by genetic variants, have been associated with core characteristics of the illness. This supports imaging genetics as a useful research perspective to investigate the biological underpinnings of mood disorders.
References
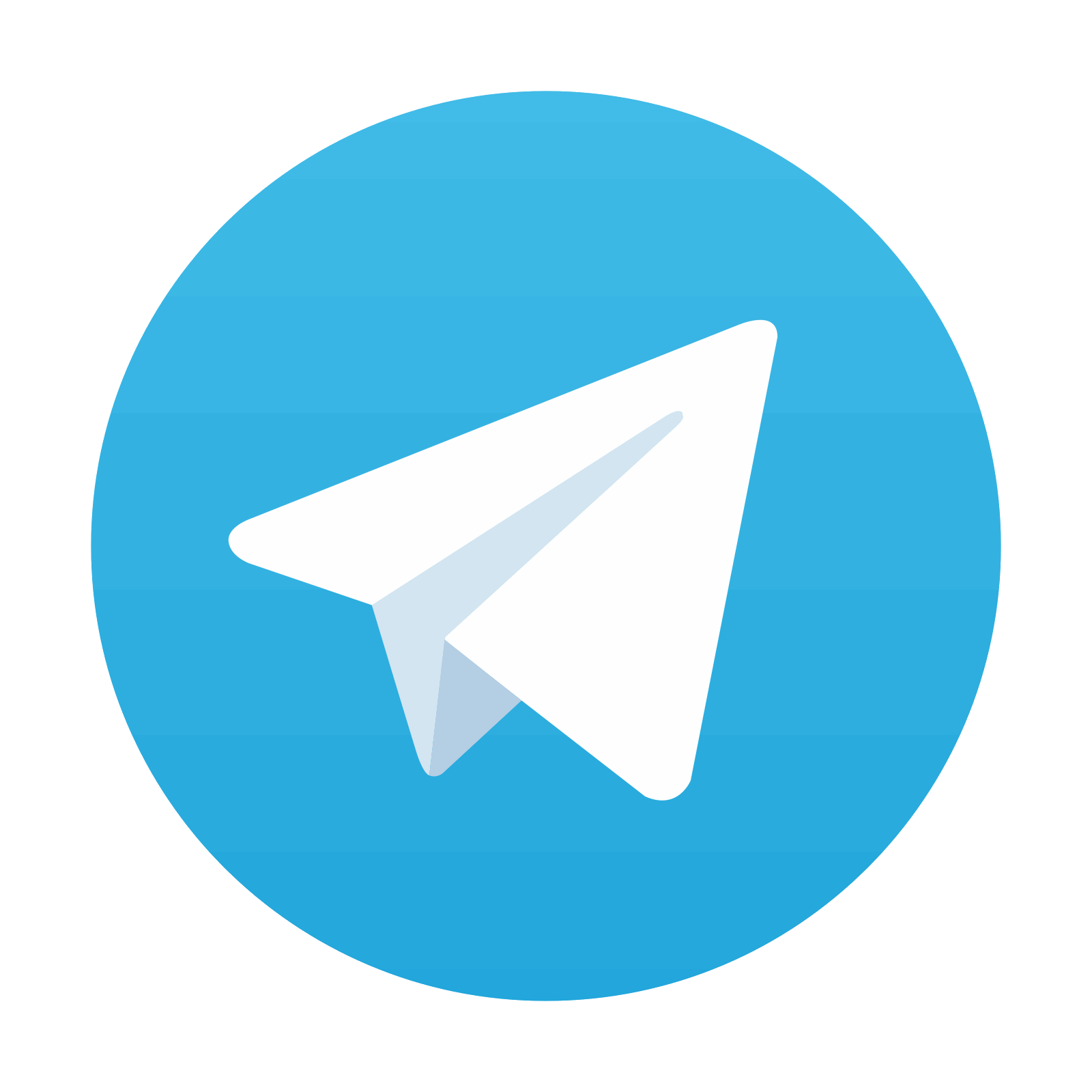
Stay updated, free articles. Join our Telegram channel

Full access? Get Clinical Tree
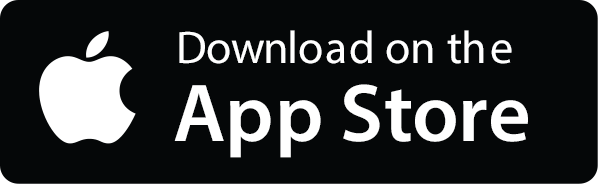
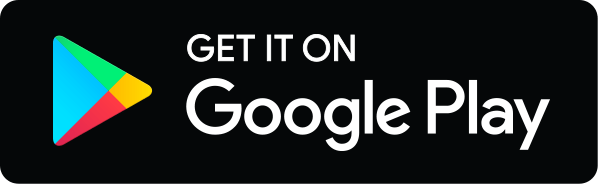
