Chapter 13 Cortical mapping in MRI-negative epilepsy surgery
MRI-Negative Epilepsy, ed. Elson L. So and Philippe Ryvlin. Published by Cambridge University Press. © Cambridge University Press 2015.
Introduction
Deciding the extension of a resection for the treatment of epilepsy should include two objectives: a. Resection of the regions originating seizures, and b. Avoidance of functional deficits resulting from the resection of functionally eloquent cortical areas. Obtaining adequate seizure control often involves extending the resection beyond the limits of the lesion or of the seizure onset zone. Since the regions originating seizures may be close to or merged with functional cortex, measures must be taken to minimize the risk of function loss. This is particularly relevant in patients with MRI-negative epilepsy, where most removed cortex may be structurally normal and may possibly be functional. Limited removal of association cortex does not usually induce severe neurological or cognitive deficits. However, removal of language, primary motor or sensory areas can be associated with corresponding transient or permanent deficits which should be avoided whenever possible. Unilateral temporal resections can induce memory deficits in the small proportion of patients where memory is lateralized to the resected hemisphere.
Identification of functional cortex (functional mapping) is therefore of paramount importance to avoid its removal and, consequently, prevent deficits associated with resection. The first approach to localization of motor, sensory and language areas can be anatomical. However, given the individual variability in the location of these areas (Figure 13.1, see also Ojemann et al. (1) and Nii et al. (2)), their detailed localization in individual patients may be required before resection to avoid their removal. For instance, although the usual anatomical landmark to avoid receptive language area is 4–4.5 cm from the temporal pole (sparing superior temporal gyrus), functional mapping has occasionally identified language areas within 3 cm of the temporal tip (3, 4).
Figure 13.1. Distribution of functional areas in epileptic patients who underwent functional mapping at King’s College Hospital, London, over the last 3 years. Patients with normal MRI are shown in red, and patients with abnormal MRI are shown in blue. The distribution of motor areas from 15 patients corresponding to the hand, wrist, and fingers are shown over the precentral cortex. The distribution of Wernicke’s area from eight patients is shown over the lateral temporal cortex. Note that there is a very significant overlap between patients with normal and abnormal MRI. However, there are some outliers among patients with normal MRI. Each circle corresponds to a subdural implanted electrode. (Figure elaborated in collaboration with Drs Susana Sainz de la Maza Cantero and Robert Morris.)
The methods used to identify functional cortex are the same for patients with MRI-negative epilepsy, for those with MRI lesions, as well as for patients undergoing resections in the absence of epilepsy (tumours, arteriovenous malformations). Electrical stimulation through intracranial electrode mats has traditionally been used for this purpose (5). In patients without epilepsy, intraoperative mapping is the preferred option, as there is no need in these patients for chronic recordings to demonstrate ictal onset. There is some evidence for reorganization of functional areas around and within macroscopic lesions (6–10), suggesting that individual variability might be larger in patients with detectable lesions than in those with normal imaging. However, the patients studied in our centre over the last 3 years showed a significant overlap in the distribution of functional maps between patients with normal and abnormal MRI studies (Figure 13.1).
Functional mapping with electrical stimulation
History: The localization of human cerebral function has long been linked to investigations in epilepsy. For over 20 centuries, epilepsy was not necessarily thought to arise from the brain and was classified as idiopathic (coming from the brain) and sympathetic or symptomatic (affecting the brain secondarily, after starting in other organs as suggested by “auras” which could start anywhere in the body). Hughlings Jackson was the first to provide a clear understanding of the significance of focal seizures as starting in the brain. The study of focal seizures then became the starting point to understand the localization of cortical function. Experimental demonstration for localization of cortical function was first provided by Fritsch and Hitzig (11), Ferrier (12) and Luciani (13). Focal movements were elicited by localized electrical stimulation of the motor cortex in animals, with focal motor seizures and generalized seizures being induced by progressively increasing stimulation intensities. Between 1901 and 1917, Sherrington reported movements of the contralateral limbs induced by stimulation of the precentral gyrus in apes, as well as conjugate eye movements induced by more anterior lateral frontal areas and occipital cortex near the calcarine fissure. Vogt and Vogt (14) confirmed the finding of contralateral eye movements elicited by stimulation of area 6 (anterior to area 4) of Brodmann, and found that motor responses elicited by area 6 required higher intensity and could be abolished by severing the fibers between areas 6 and 4. Consequently, they described area 4 as “primary motor field,” subdivided area 6 into “secondary and tertiary motor fields” and coined the term “adversive” for contralateral eye, ears and head turning as if the animal was looking and listening to something on the contralateral side to stimulation. Foerster (15) found that higher intensities applied to area 6, to area 5 (parietal) and to area 22 (temporal) induced bilateral movements and mass turning, and called these areas “extrapyramidal,” considering them as secondary motor areas, to distinguish them from the primary motor area (area 4) which gives rise to the pyramidal tract. Penfield and Jasper (5) systematically applied electrical stimulation to map human cortical function during presurgical assessment of epilepsy. They confirmed area 4 (precentral) as the primary motor cortex and areas 1–3 (postcentral) as the primary somatosensory cortex in man (Figure 13.2A). They designed their famous homunculus (Figure 13.2B) and described arrest of voluntary movements by stimulation of the supplementary motor cortex and of the second sensory area (Figure 13.2C). They emphasized the presence of connections between pre- and postcentral areas, describing both areas as a “sensorimotor unit,” a view that has been confirmed by more recent studies (16, 17).
Figure 13.2 Penfield and Jasper’s diagrams showing the topographical organization of motor and somatosensory areas. A. Sensory sequence in the postrolandic cortex shown on a cross-section of the cerebral hemisphere. Lengths of the black lines in the cortex indicate the approximate extent of the representation of sensation for each part of the body. B. Sensory and motor homunculus. This was prepared as a visualization of the order and comparative size of the parts of the body as they appear from medial and above down upon the perirolandic cortex. C. Map of the somatic motor and sensory areas. The frontal and parietal regions are spread up to form a map from the corpus calosum to the insula. Thus, the medial surface of the hemisphere lies above, the bank of the fissure of Sylvius below, and the lateral surface between the two. The supplementary motor and sensory areas, and the second somatic sensory areas are stippled. The perirolandic sensorimotor strip is indicated by parallel lines. The frontal eye field, whose stimulation induces aversive eye and head rotation, is labeled as “gaze.”
General procedure: Electrical stimulation allows functional mapping by identification and demarcation of the areas involved in a particular function. Functional mapping can be obtained during chronic intracranial recordings in the telemetry ward (extraoperative) or during surgery under local anaesthesia in the operating theatre (intraoperative). Functional mapping is best performed with the patient awake and relaxed, though motor responses can be induced under general anaesthesia, usually requiring higher intensities and inducing cruder responses, thus providing less fine spatial resolution than those seen with the awake patient. For mapping of sensory or speech areas with electrical stimulation, the patient must be awake. Patients must be informed on the expected clinical responses that may occur, as these may be distressing if unexpected (e.g., forced movements, speech arrest), and on the risk of provoking seizures. Functional mapping is best performed through mats of electrodes in order to guarantee adequate spatial sampling. Stimulation of mesial temporal structures through the deepest contacts of subtemporal strips can be painful, presumably due to stimulation of nearby cranial nerves. Electrical current can be passed between pairs of adjacent electrodes at progressively increasing current intensities and durations (typically biphasic 0.3 to 1 ms pulses of 0.5 to 15 mA at 50 Hz, lasting for up to 10 seconds), while simultaneous electroencephalographic recordings are obtained with the electrodes not used for stimulation. Stimulation is carried out with progressively increasing strength, starting at low intensities (0.5 mA) and short durations (1 second), then gradually increasing duration up to 6–10 s, then increasing intensity with short duration, then increasing duration and so forth (e.g., 0.5 mA for 1 second, then 0.5 mA for 3 seconds, then 0.5 mA for 6 seconds, then 1 mA for 1 second, then 1 mA for 3 seconds, etc.). The duration and intensity of stimulation are thus progressively increased until at least one of the following circumstances occurs:
a. Positive clinical signs or symptoms (responses) are observed associated to stimulation: Stimulation of motor cortex induces contralateral limb movements, brief dystonic postures, or muscle contractions. When somatosensory cortex is stimulated, patients may report contralateral paraesthesia (tingling, numbness, burning or sensation of electricity). When visual cortex is stimulated, patient may describe contralateral visual symptoms (flashing lights, lines, colours, moving forms).
b. Negative clinical responses are observed associated with stimulation: These responses consist of interruption of normal ongoing function. To identify such responses, the patient must be engaged in activity. Speech arrest occurs when motor speech area is stimulated, and inability to understand words occurs when receptive speech areas are stimulated. Arrest of motor activity can occur while stimulating premotor and supplementary motor cortices, which may manifest as negative myoclonus and hand or limb drop.
c. After-discharges are observed on the EEG recording immediately following stimulation: Brief runs of epileptiform discharges or ictal EEG patterns can be induced by electrical stimulation (Figure 13.3). They are usually short and asymptomatic. However, stimulation with higher intensities should be avoided whenever possible, as it can induce longer after-discharges evolving to overt seizures. This circumstance may have to be reconsidered in children (see below under “Electrical stimulation in children”).
d. The upper limit of stimulation intensity and duration are reached, usually around 7.5–15 mA for 6–10 sec.
Figure 13.3 Two examples of after-discharges in the same patient. Functional mapping was performed via a lateral frontal subdural mat and Sylvian and central 4-contact subdural strips. A. Stimulation through electrodes 21 and 22 of the mat (circled on the X-ray inset) for 3 seconds induced sustained after-discharges for several seconds at nearby electrodes (arrow). B. Stimulation of electrodes 3–4 of the Sylvian strip (circled on the X-ray inset) for 1.5 seconds induced a 2-second burst of after-discharges (arrow) at the remaining electrodes of the strip. Note the large stimulation artefact obscuring EEG recordings.
Functional mapping can also be carried out through depth electrodes. These are less appropriate than mats in terms of spatial sampling, but allow testing the same functions with lower intensities (up to 3 mA usually). Also, one Hz stimulation can be used to test the motor cortex and underlying pyramidal tract with depth electrodes.
While stimulation is being carried out, clinical personnel should observe the patient and the EEG for the occurrence of clinical responses or after-discharges. Should these occur, stimulation must be repeated for reliability after at least 30 sec. The effects of stimulation are reproducible and short lived, usually lasting for the duration of stimulation or a few more seconds, unless an epileptic seizure is induced. The onset of positive or negative signs or symptoms without after-discharges is the best indicator for localization of motor, sensory or speech areas in the cortex underlying the stimulating electrodes. Similar signs or symptoms, when associated with after-discharges, have more limited value, as they may be due to cortical activation by after-discharges, which in 8% of cases propagate to regions relatively distant from the stimulated cortex (18), possibly leading to mislocalization of function. The threshold for functional responses and for after-discharges is generally different at each stimulated site, may differ according to the intertrial period and may show day-to-day fluctuations, making it important to optimize the stimulation intensities for each tested site, progressively increasing stimulation according to the procedure described above. Should after-discharges occur, stimulation at the same site can be carried out again at slightly lower intensity, which sometimes avoids the occurrence of further after-discharges, and then stimulation can be gradually increased to test for functional threshold. In patients with large numbers of after-discharges, low doses of lorazepam or diazepam can be administered prior to stimulation.
Positive clinical responses are sometimes induced by stimulation of secondary motor and sensory areas. Stimulation of association cortex, other than speech areas, does not usually induce clinical responses.
Changes in electrical charge density induced by this procedure and responsible for the responses are very localized, attenuating rapidly over short distances, as shown by a finite element model (19) and by the fact that clinical responses are very localized, i.e., change or disappear dramatically when moving the stimulating electrodes only a few millimetres apart (20, 21).
Safety issues: The charge used for stimulation is the product of current intensity and pulse duration and can be expressed as per phase or as charge density (charge per unit of electrode area). Smaller electrodes will induce higher charge densities, which may need to be compensated with lower intensities. Chronic stimulation with charge density at 55µC/cm2/phase was not associated with pathological changes (22), suggesting that stimulation with charge density below this figure should be safe. In young children, where myelinization is not complete, high current densities may be necessary (22). In practice, the main side effect of electrical stimulation is the induction of a seizure, which in itself may carry useful information (see below). Over a 20-year period, the author has seen only one case of secondarily generalized convulsive status epilepticus induced by electrical stimulation during functional mapping in a child with epilepsia partialis continua.
It has been suggested that stimulation with high frequencies (500 Hz) applied to motor cortex can induce contralateral muscle potentials with possibly less risk of inducing seizures (23). No kindling has been reported in humans. Stimulation thresholds do not seem to decrease significantly with successive stimulation trials in man, and kindling of the neocortex has not been observed in higher primates. Apart from the specific safety issues with regard to electrical stimulation, surgical procedures carry the general risks of untoward effects associated with neurosurgery and chronic implantation of intracranial electrodes (infection, hemorrhage, subdural hematoma).
Mapping somatic sensorimotor cortex: Stimulation of the motor cortex induces contralateral muscle tonic or clonic contractions and movements of the corresponding limbs and muscle groups. Similarly, stimulation of the somatosensory cortex induces contralateral paraesthesia in the corresponding limbs, most frequently tingling, numbness, burning or tightening, and less frequently painful sensations or a sense of movement. Such positive clinical responses are usually rather localized, most frequently involving one hand or one side of the face as these are the areas with the largest cortical representation. Indeed, this was the methodology used by Penfield to design his homunculus (5). More rarely, stimulation can induce both motor and sensory responses, either because both motor and sensory areas intermingle or due to stimulation of nearby secondary cortex or association fibers. Indeed, sensory responses can be elicited precentrally and vice versa in approximately 25% of subjects (5). Cessation of ongoing motor activity and dystonia has been reported during stimulation of premotor cortex (24). Such arrest of motor activity affects particularly fine distal movements and can be associated with contralateral or ipsilateral decreased or increased motor tone. Motor arrest can be tested by asking the patient to read during stimulation and, if reading arrest occurs, stimulation is repeated while carrying out contralateral alternate movements to distinguish a negative motor response from the speech arrest elicited when stimulating speech areas. Stimulation of the primary motor cortex between upper face and finger areas, and stimulation of the premotor cortex anterior to this region, can elicit head and eye turning in the direction contralateral to stimulation (adverse rotation) (5, 25). These regions have also been called frontal eye field. Stimulation of the motor cortex around the lip/mouth area can elicit vocalization, a long drawn-out vowel sound made by symmetrical contraction of mouth, pharynx, larynx and respiratory muscles. Respiratory arrest has been described with stimulation of the lateral lower end of the sensorimotor strip (5). Unilateral stimulation of mouth, tongue or throat areas can induce bilateral movements or contralateral unilateral responses. Neck movements (aside from turning) can be induced by stimulation of two regions, below and above the face area.
In humans, the supplementary motor cortex is the region anterior to the leg area in the interhemispheric fissure and dorsal aspect of the superior frontal gyrus. Stimulation of this region can induce aversive movements (i.e., this region is also called the supplementary eye field) in addition to a variety of movements and dystonic postures (often bilateral, involving aversive head rotation, and posturing of shoulders and elbows), arrest or slowing of voluntary movements and speech, vocalization, sensations (general body sensation, sensation of flush, cephalic, epigastric or indescribable sensations, contralateral or bilateral leg sensations), autonomic changes (pupillary dilation and changes in heart rate) and aphasia (5). The supplementary motor cortex shows a somatotopic organization (26).
Motor (largely precentral) and sensory (largely postcentral) homunculi are rather similar and tend to run in parallel, except that there is no motor representation for scalp or genitalia. Sensory responses to stimulation are contralateral except those induced by stimulation of face and tongue areas which can be bilateral, and those induced by stimulation of the throat area which are usually bilateral. Stimulation of the tongue and mouth areas can induce numbness or tingling, but not taste. There is a second sensory area over suprasylvian cortex, covering pre- and postcentral regions (5). Stimulation of the second sensory area induces similar responses to those described for the primary sensory area, often associated with a desire to move or losing strength, or temporary paralysis in the corresponding contralateral (and sometimes ipsilateral) region. Interestingly, cortical stimulation can elicit somatosensory sensations in phantom limbs (27).
Single electrical pulses can induce very localized motor responses, often restricted to one finger or muscle (28).
Mapping visual cortex: Stimulation of the occipital visual cortex induces phosphenes (flickering lights, dancing lights, stars, colors, shades, gray spots) or elementary visual shapes (lines, whirling circles) seen in the contralateral visual field. In man, areas 18 and 19 are most easily accessible with subdural electrodes. Area 17 at and around the calcarine fissure (primary visual cortex) is rarely accessible, except the macula, which is represented at the occipital pole. Stimulation of the parieto-occipital region can induce adversive eye movements. In addition to visual cortex, resections should also spare visual pathways, such as optic radiations and other connections that can be identified by electrical stimulation (29).
Olfactory and gustatory sensations: Disagreeable smells can be elicited by stimulation of the uncus and nearby olfactory bulb and amygdala. Tastes, usually bad, can be induced by stimulating the insula (5).
Mapping auditory cortex: Stimulation of the posterior half of the superior temporal gyrus can induce the perception of ringing, humming, buzzing and other sounds, as well as deafness and distortion of incoming sounds. Sounds are referred to the opposite ear or to both ears. Stimulation of the superior temporal gyrus can also be associated with suppression of hearing (30).
Stimulating the insula (see also section below on autonomic function): It appears that the insular cortex is connected to two different cortical networks, a visceral network extending to the temporomesial structures and a somesthetic network reaching the opercular cortex, which induce visceral or somatosensory sensations with stimulation of the anterior or the posterior insula, respectively (31).
Mapping language: The specific areas of the brain responsible for language function were some of the first functional areas to be identified, on the basis of patients who developed aphasia after brain lesions. The initial findings showed that injuries located in the inferior frontal gyrus were associated with disruption of executing speech and writing (32). Patients with more posterior lesions, in a region located mainly over the posterior and superior aspect of the lateral temporal lobe, developed problems in the analysis and interpretation of language (33). Essentially, the region described by Broca was associated with speech production (and is often called Broca’s area or motor speech area), whereas the area described by Wernicke was engaged in speech understanding (and is commonly referred to as Wernicke’s area or receptive speech area). The distributions and connections between language areas are shown in Figure 13.4. In addition, mapping with electrical stimulation has shown that the basal temporal language area is located in the area of the fusiform gyrus, 1–9 cm posterior to the temporal tip (34). Bilateral representation of the basal temporal language function has been suggested by event-related potentials (35) and functional neuroimaging (36).
Figure 13.4 Lateral view of the human left cerebral hemisphere, highlighting the main areas involved in movement control and language. The arcuate fasciculus connects Wernicke’s and Broca’s areas.
Lateralization of language has been traditionally achieved with the amytal test (also called, amylobarbital or Wada test). Alternative methods for lateralization are presently emerging (see Chapter 8).
For localization of speech areas, rather than their lateralization, functional mapping with electrical stimulation may be required. Speech responses to electrical stimulation are usually negative. Arrest of ongoing speech can be induced by stimulation of Broca’s area and disturbance in speech understanding can be observed when stimulating Wernicke’s area. Consequently, in order to assess language function the patient must be undertaking a language task during stimulation. Different tasks can be tested which can be localized to different cortical areas (Table 13.1). Stimulation is started at the beginning of the task and patients are assessed during stimulation. If speech disturbance occurs, stimulation should be repeated for reliability.
For localization of Broca’s area, the traditional task consists of asking the patient to read, count or recite a known lullaby or song, while checking for pauses in speech associated with stimulation. For identification of the Wernicke’s area, three different methods with minor modifications are the most commonly used (Figure 13.5); see also Ojemann, 1981 (37):
a. Auditory responsive naming: During stimulation, the patient listens to a brief description (“Tell me what is a barking pet….”) and should name the described item.
b. Picture selection: During electrical stimulation, the name of an item is said aloud by the examiner, and after stimulation the patient has to point at the named item among four drawings of common objects.
c. Visual confrontation naming: During stimulation, the examiner says “What is this?” while a drawing of a common object is presented to the patient who has to name it.
Figure 13.5 Commonly used paradigms to identify receptive (Wernicke’s) language areas showing the time course of language task and stimulation. The down arrows indicate the onset of electrical stimulation.
Both auditory and visual tasks should be used in each patient, as the distribution of the auditory naming site (auditory responsive naming) appears to be more anterior in the temporal lobe than the visual naming site (picture selection and visual confrontation naming) (38, 39).
More recently other tasks have been suggested for assessment of Broca’s and Wernicke’s areas, including spontaneous speech, word repetition, object description, reading, comprehension, and verb generation (39). Results from these tasks can point to close, but different, brain regions to those identified with more traditional tasks. However, the number of different tasks to be performed in a patient is necessarily limited. A selection of the most clinically relevant tasks is required for each individual case.
Electrical stimulation of the basal temporal language area elicits a range of responses from complete expressive, receptive and repetition deficits at higher stimulus intensities to anomia and other aphasic symptoms at lower intensities (40, 41).
In essence, electrical stimulation behaves as a temporary and reversible lesion, replicating the deficits associated with resection, and is presently considered as the “gold standard” for the identification of the specific areas responsible for language (37, 39). The difficulties in mapping language in children have been reviewed elsewhere (42).
New noninvasive functional methods (PET, MEG, fMRI) have implicated larger areas of the brain in some aspects of speech. For instance, although the superior temporal gyrus appears to be related to the analysis of the speech sound, other temporoparietal regions can be necessary for language comprehension. These regions include the midtemporal gyrus, the inferior temporal gyrus, the fusiform and the angular gyrus (Brodmann areas; 20, 21, 27, 36, 39). In the case of language syntactic production and processing, different regions located in the inferior frontal gyrus appear to be responsible (Brodmann areas; 44, 45, 47). Electrical stimulation and diffusion tensor imaging can identify the arcuate fasciculus (connecting Broca’s and Wernicke’s areas), and both techniques colocalize well with anterior language areas, but less so with posterior language areas, suggesting that the latter may be more dispersed (43).
Autonomic function: Stimulation of areas just above the Sylvian fissure and in the insula can elicit autonomic changes such as salivation, taste and abdominal sensations (nausea, epigastric sensations). Stimulation of the supplementary motor cortex can induce pupillary and heart rate changes.
Mapping memory: Recent memory is processed independently by both hemispheres, particularly by medial temporal structures in either hemisphere (44, 45) although lateral temporal neocortex may also be involved (46–48), especially for verbal memory in the dominant hemisphere (49, 50). Occasionally, memory processing is lateralized to the side of the proposed resection and localization of the areas involved in memory may be desirable. This requires timing of stimulation to a memory task in order to establish if lower memory performance is associated with electrical stimulation. Such tests are time consuming (51) and are not standardized at present. Memory mapping has been restricted to those patients who failed the Wada test (see below) or those whose livelihood depends on having good memory (52). In such patients, resection could be tailored to spare the lateral temporal memory sites and the anterior portion of the hippocampus (48, 49)
Stimulation of the temporal lobe (not necessarily medial) can induce memory recollections and disturbances such as déjà vu (5). Feindel and Penfield found arrest of memory encoding when stimulating near the human hippocampus (53). However, disruption of memory performance with unilateral electrical stimulation of human medial temporal structures only occurred in a minority of patients and required the induction of after-discharges by the stimulus (54, 55). Nevertheless, temporary amnesia could be induced more readily when stimulating medial temporal structures bilaterally with trains of electrical pulses lasting 3 to 50 seconds (56–8). Thus, these early studies suggested that in order to demonstrate an effect on episodic memory, stimulation had to be either unilateral with after-discharges or bilateral. Later studies provided evidence that electrical stimulation of medial temporal structures at a level below that required for eliciting after-discharges can induce material-specific deficits in recognition memory tests, either by stimulating unilaterally at a single site (51, 59, 60) or bilaterally (61). More recently, Lacruz and colleagues (45) have shown that even a single electrical pulse applied to the hippocampus can produce memory deficits if applied bilaterally but not unilaterally. Stimulation of the lateral temporal neocortex of the dominant hemisphere can also induce errors in verbal memory (62). Memory is clearly a complex function which involves a variety of sites and tasks. For this reason, memory mapping with electrical stimulation is nonstandard, and reviews of strategies can be found elsewhere (63, 64).
Indications of electrical stimulation for mapping: The main indication for functional mapping is planning of resections close to primary motor, sensory or language areas. For language testing, it is preferable that the dominant hemisphere is identified prior to stimulation (see Chapter 8). The need for mapping in the assessment of standard temporal lobectomies is controversial, as standard temporal lobectomies are made smaller in the dominant hemisphere to minimize language deficits. In our experience, speech mapping of Wernicke’s area is often useful in patients with lateral temporal epilepsies, particularly if seizure onset is posterior.
Intraoperative vs. extraoperative functional mapping: Intraoperative mapping under local anaesthesia requires patient cooperation under difficult circumstances and time is usually limited to around 1 hour. Intraoperative mapping may not be possible in children or in patients with learning difficulties. In such patients and in those requiring chronic intracranial EEG recordings for seizure localization, mapping may be more accurately performed during chronic recordings, in one or several sessions over several hours, allowing for test and retesting of responses for reliability. Intraoperative mapping requires simultaneous acute electrocorticography to record after-discharges, since induction of seizures in the operating theatre in a patient with an open skull is highly undesirable. Stimulation at or above after-discharge threshold must be reduced to a minimum, as the risk of inducing seizures increases under these circumstances. The methodology for functional mapping during intraoperative recordings is essentially similar to that used during extraoperative recordings. However, the methods used to perform operations with intraoperative mapping vary among centers. Opening the skull with the patient awake is often disagreeable for patient and surgeon. In our centre, we tend to carry out the procedure in two consecutive days. On the first day, the craniotomy is performed and closed under general anaesthesia. On the second day under local anaesthesia, the skin and craniotomy are reopened, the electrodes are applied to the cortex, functional mapping with electrical stimulation is performed and a tailored resection is carried out. This allows for final functional evaluation during the resection. Other centers prefer to carry out the complete procedure in one session, including periods of general and local anaesthesia. Under this approach, it may be difficult to maintain an airway access while the patient is awake. Lateral decubitus and propofol anaesthesia may be helpful (65). A combination of propofol and dexmedetomidine sedation appears to be successful in transitioning patients from asleep to awake (66). There is evidence that intra- and extraoperative mapping are mutually complementary (67).
Electrical stimulation in children
Experimental and clinical evidence suggest that neuronal plasticity in the immature brain can allow reorganization of function after brain injury (68–70). Language can shift to the opposite hemisphere and full recovery can follow resections in the dominant hemisphere before the age of 6–7 (69, 71). However, resection of primary motor cortex tends to generate permanent motor deficits, particularly affecting fine hand movements. Myelinization in children is incomplete and functional mapping in children should be carried out with caution because they tend to require higher current intensities or pulse durations than adults. In children below the age of 10, the threshold for after-discharges is often lower than the threshold for functional responses. Functional mapping is then carried out by identification of the areas that consistently show the lowest functional threshold. Identification of sensory or language areas, which requires active patient cooperation, is not possible in infants, toddlers and young children below the age of 4. Somatosensory evoked potentials may prove more helpful in this age group. The challenges of functional mapping in children have been extensively reviewed (72–74).
Functional mapping without electrical stimulation
A number of alternatives to electrical stimulation have been used or are being evaluated for functional mapping. Recording of evoked responses on the surface of the cortex has been used to map somatosensory cortex (75–79), auditory areas (80) and motor cortex (81). Muscle responses evoked by cortical stimulation can be used to identify motor cortex (79, 82, 83). Fast rhythms associated with functional activities are increasingly being used to identify the areas involved in motor, sensory, language and cognitive functions, but their utility is still under evaluation (84–89). Some encouraging reports suggest that transcranial magnetic stimulation could be used for noninvasive functional mapping (90). Maps of magnetoencephalographic (MEG) responses can be used to localize sensorimotor cortex (91) and language areas (92, 93). The use of neuroimaging and the amytal test for functional mapping are reviewed in Chapter 8. It is becoming increasingly apparent that most techniques are complementary rather than exclusive.
Electrical stimulation for the identification of epileptogenic cortex
Electrical stimulation with the parameters used for functional mapping often induces runs of epileptiform discharges or other ictal patterns in the EEG. A number of terms have been used to designate such responses: after-discharges, electrically induced seizures, electrographic seizures and subclinical seizures. The latter two terms are inaccurate because electrographic/subclinical seizures can occur spontaneously and because such discharges are not necessarily asymptomatic. After-discharges usually last for a few seconds and tend to remain localized to the areas around the stimulated cortex. Under these circumstances they are usually not associated with clinical signs if they remain restricted to noneloquent cortex. However, if after-discharges propagate to eloquent areas, their presence may affect the interpretation of functional mapping, because clinical signs may be due to the disruption of functional areas by after-discharges rather than to the direct local effects of electrical stimulation. Occasionally after-discharges last longer and propagate to more widespread regions, sometimes evolving into a clinically overt seizure with cognitive impairment, sensory or motor manifestations, or other ictal signs. The use of after-discharges to detect epileptogenic areas has long been debated (94–96). After-discharges can be induced in areas other than the seizure onset zone and their presence is not a very clear marker for epileptogenic cortex. In early studies, it was suggested that the region whose stimulation produced the longest after-discharges or the only after-discharges was the origin of spontaneous seizures in 75% of cases (5). A study of 133 patients with temporal lobe epilepsy found a 77% concordance between spontaneous seizures and after-discharges (97). Another large study of 126 patients, which included 38 patients having a single seizure focus, found concordance between the topographies of focus and after-discharges in 88% of temporal lobe seizures, 92% of frontal seizures and 100% of posterior seizures (96). The areas whose stimulation induces the patient’s habitual aura or habitual seizures may be a more reliable marker for epileptogenicity. A study of 72 patients reported a high correlation between onset zone for spontaneous seizures and the sites stimulated to induce the patient’s habitual auras or seizures, particularly for medial temporal lobe epilepsy (98).
The area showing lowest current intensity required to induce after-discharges (lowest after-discharge threshold) does not seem to be a reliable predictor of spontaneous seizure onset (98). After-discharge threshold is usually decreased at the site of seizure onset but can be elevated in about 25% of patients (96), presumably due to the neuronal loss inherent to some pathologies. Furthermore, once after-discharges have been elicited, their threshold may change (94).
Interestingly, briefer stimulation with a 1-millisecond single electrical pulse can induce EEG responses which are useful to identify epileptogenic cortex (99).
What can we do if seizures arise from functionally eloquent areas?
Removal of the precentral motor cortex induces paresis of the corresponding contralateral muscle groups. In the case of the upper limb, gross movements of shoulder, arm and hand may return after a few months, but fine finger movements seldom, if ever, recover. Ablation of the lower limb areas induces partial paralysis. Excision of the face area can induce hemiparesis of the lower face, but does not usually affect the forehead or eye closure, in contrast to lesions of the cranial nerves or their nuclei. No clear paresis is observed after removal of neck or trunk areas. Removal of motor cortex in children can induce similar deficits to those seen in adults, in addition to affecting growth of the corresponding areas. Transient occulomotor paralysis has been reported, associated with lesions of the frontal eye fields (100, 101). Removal of the hand somatosensory area is associated with astereognosis, which is often more disabling than initially thought. Resection of the second sensory area does not usually induce deficits. Ablation of the supplementary motor area does not seem to induce significant permanent deficits. Transient dysphasia (in resections in the dominant hemisphere), contralateral hemiparesis or neglect, or slowing of contralateral movements and contralateral forced (reflex) grasping (difficulty in releasing objects after grasping) have been described after removal of the supplementary motor area (5, 102). Speech deficits after resections before the age of 6–7 can be followed by complete recovery (see above). Unilateral resection of the auditory areas does not usually induce deficits, as hearing is processed bilaterally. Consequently, lesions near auditory cortex may be resectable without auditory mapping (103), particularly in the nondominant hemisphere. Resection of the primary visual cortex around the calcarine fissure (area 17) is associated with contralateral visual field defects. Deficits after resections of other visual areas are less predictable as they may receive bilateral representation. The contralateral visual field deficits occasionally induced by temporal lobectomies are due to severing the optic radiations.
It is usually accepted that, once essential cortex is identified, resection must leave at least 0.5–1 cm margin around eloquent areas. The usefulness of functional mapping in standard anterior temporal lobectomies is controversial as lobectomies are smaller on the dominant hemisphere (limited to the anterior 4 cm from the temporal tip) in order to spare language areas. However, language areas have been found in the cortex to be resected in a small proportion of patients (104) and transitory aphasias have been described after such standardized resections (105). It has been suggested that intraoperative functional mapping could help prevent speech deficits after right-sided resections in right-handed patients with ictal speech and right-sided activation on fMRI speech mapping (106).
In patients where seizures arise from functional cortex in the hemispheric convexity, multiple subpial transection has been proposed (107), a procedure that can preserve function while relieving epilepsy in 33–80% of patients (108–111).
Evaluation
Electroencephalographic recording combined with functional mapping using subdural electrode grids is a well-established technique that allows a tailored, maximal resection of epileptogenic tissue with minimal injury to critical cortex (111). It is nevertheless difficult to formally evaluate the efficacy of a procedure regularly used to prevent deficits because the deficits that would have occurred had the procedure not been performed are unknown, and controlled studies are usually unethical. After detailed functional mapping, resections involving the primary motor cortex can abolish seizures and avoid motor deficits (113), or induce mild deficits in a minority of patients (10).
In temporal lobectomies performed without functional mapping, either no language deficits are observed (4), or small deficits in naming can be seen in patients undergoing temporal lobectomy in the dominant hemisphere, particularly in those with later-onset epilepsy (114). Rarely, right-sided temporal lobectomies performed on the dominant right side can induce aphasia (115).
Clinical case
Patient with unremarkable previous medical or family history presents with late-onset epilepsy at age 29. During the first alleged episode, he woke up at night with a strange dizzy feeling and being generally unwell. Approximately a year later, he had a convulsive seizure in sleep and suffered several complex partial seizures over the following 2 years. He was diagnosed with epilepsy. He was then seizure-free for 3 years until he had another convulsive tonic–clonic seizure and started to suffer daytime seizures. The convulsive seizures went away but he continued to suffer complex partial seizures, which are described as follows: his mind feels jumbled up, he tries to get out of such a situation and moves outside, he has an aura of déjà vu and loses awareness of his surroundings, according to his wife he licks his lips and rubs his fingers. The MRI examination was normal. The FDG-PET scan showed a degree of hypometabolism over the left temporal lobe.
The interictal EEG showed left temporal slowing of the background activity with intermittent sharp waves in the same region. One complex partial seizure occurred during EEG recording which was associated with nonforced turning of the head to the left and difficulty with his speech at the end of the seizure. There was prominent slowing over the left temporal area at seizure onset, although this is in the delta range and more posterior than expected in hippocampal sclerosis, possibly suggesting a lateral temporal onset.
The patient was admitted for intracranial EEG–video telemetry with subdural electrodes (Figure 13.6). A lateral mat was included, given the above suggestion of lateral temporal onset and postictal dysphasia. This approach would provide sufficient spatial sampling for speech mapping and to detect a lateral temporal seizure onset. Two complex partial seizures with a secondary generalization occurred with a clear ictal onset over the mesial contacts of the left anterior subtemporal strip electrode. Single pulse electrical stimulation showed delayed responses over the medial contacts of the anterior subtemporal electrode. The results of speech mapping following an auditory responsive naming paradigm are shown in Figure 13.6. Since Wernickés area was far from the seizure onset zone and from the delayed responses to stimulation, it was decided to offer a left temporal lobectomy.
Figure 13.6 Intracranial EEG–video telemetry with subdural electrodes. A 32-contact lateral temporal and suprasylvian mat was implanted through a left lateral craniotomy, in addition to four 8-contact subdural strips: frontal, anterior temporal, posterior temporal, and occipital. Speech mapping following an auditory responsive naming paradigm was carried out through adjacent pairs of electrodes. Stimulation at 2.5 mA through the contacts shown in blue was associated with naming deficits and, consequently, these contacts were considered to be overlying Wernicke’s area. Stimulation through contacts 7–8 of the mat (superior and posterior corner) was also associated with naming deficits but only with high intensity of stimulation (7.5 mA), and consequently these contacts were thought to be close but not overlying speech areas. The mat contacts are labelled 1 to 32, with 1 being the contact at the anterior and superior corner of the mat, and contact 8 being the contact at the posterior and superior corner of the mat.
References




















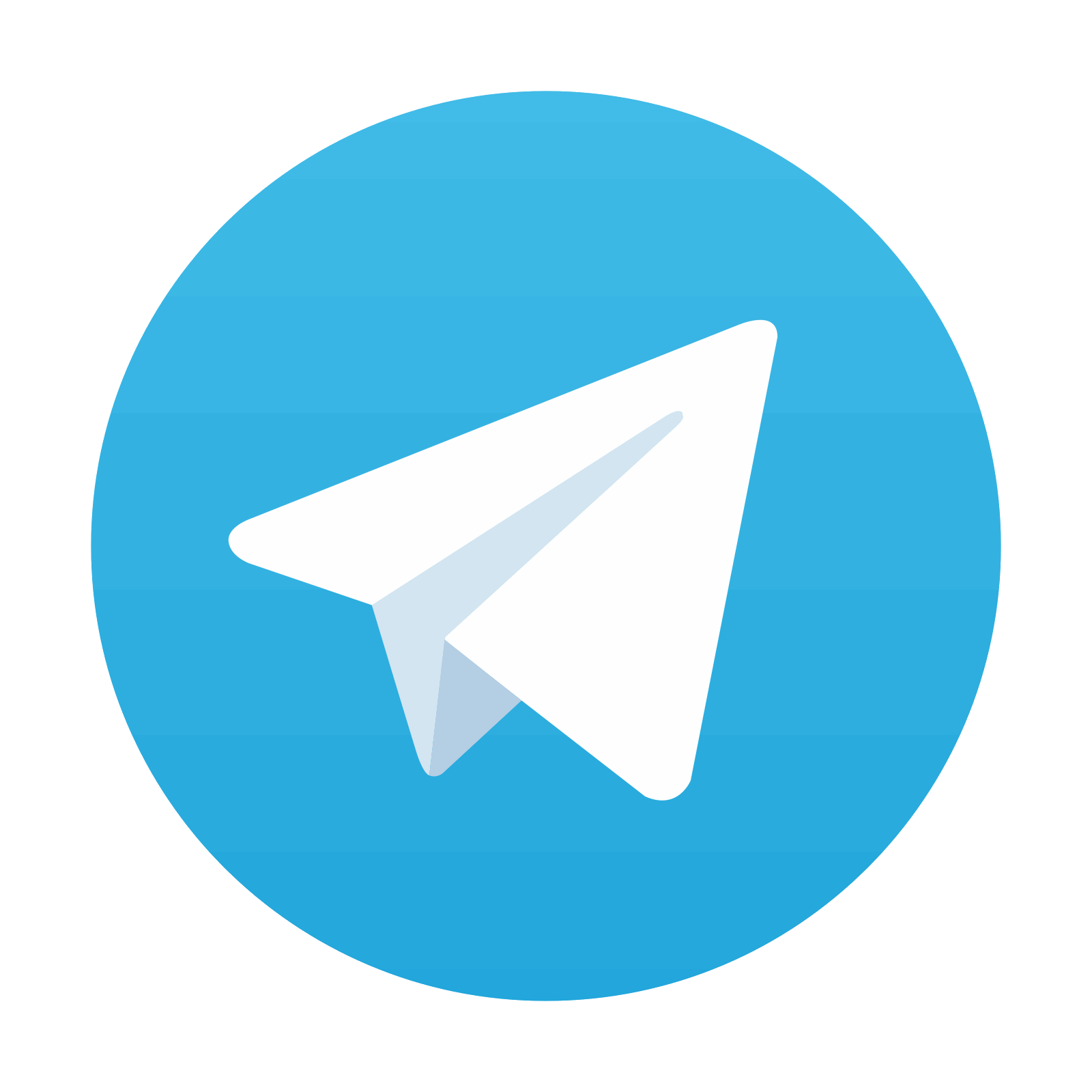
Stay updated, free articles. Join our Telegram channel

Full access? Get Clinical Tree
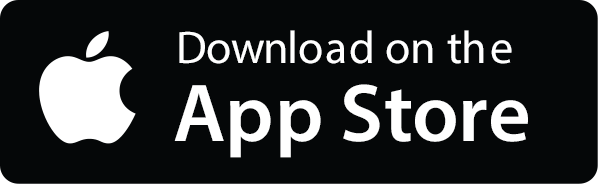
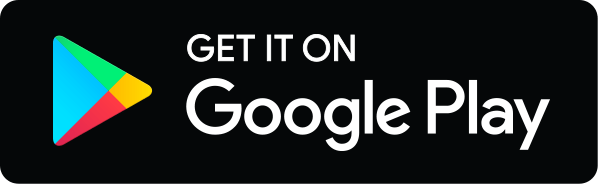
