Chapter 15 Localization and surgery in MRI-negative frontal lobe epilepsies
MRI-Negative Epilepsy, ed. Elson L. So and Philippe Ryvlin. Published by Cambridge University Press. © Cambridge University Press 2015.
Introduction
Frontal lobe epilepsies (FLE) account for 20% to 25% of all patients with antiepileptic drug (AED)-resistant focal epilepsies undergoing resective epilepsy surgery, second only to temporal lobe epilepsies (TLE).1, 2 The presence of an MRI-defined focal lesion is the single most important predictor of favorable seizure outcome following surgeries involving frontal lobes.2–4 Therefore, selection of patients with AED-resistant epilepsies suspected to be originating from the frontal lobe for resective surgery, whose MRI fail to show a lesion, poses one of the most challenging problems in the field of epilepsy surgery. Many epilepsy surgery centers even exclude MRI-negative FLE patients from presurgical evaluation. However, recent advances in the understanding of the various FLE sub-syndromes through meticulous study of successfully operated patients, as well as advances in noninvasive source localization and intracranial EEG studies, have made it worthwhile to pursue presurgical evaluation in MRI-negative patients with a reasonable chance of favorable postoperative seizure outcome.2–4 In this chapter, we intend to review the diagnosis, presurgical evaluation, and surgical treatment of MRI-negative and AED-resistant FLE, and also highlight the various challenges encountered en route.
Prevalence of AED-resistant MRI-negative FLE
Antiepileptic drug-resistant epilepsy is diagnosed when there is inadequate control of seizures despite use of potentially effective AEDs at tolerable levels for 1 to 2 years. Among patients with AED-resistant epilepsies, it is difficult to compute the true prevalence of MRI-negative FLE. The majority of the surgical series do not report on the patients who are not selected for surgery, and at many centers, patients with normal MRI do not undergo presurgical evaluation. On the other hand, in nonsurgical series, in the absence of an MRI lesion, reliable localization of the epileptogenic zone may often not be possible. In a study (which included both temporal and extratemporal epilepsies) from the Department of Epileptology, University of Bonn, Bonn, Germany (hereafter referred to as the Bonn series), out of 1192 patients who underwent presurgical evaluation during a 7-year period, 191 MRI-negative patients were identified (16% of all presurgical patients).3 While 73% of patients with a demonstrable lesion in the MRI underwent surgery, only 15% of MRI-negative patients were operated on.3 At the authors’ epilepsy center, out of 285 consecutive patients with extratemporal lobe epilepsy who underwent presurgical evaluation, 122 (42.8%) had normal MRI; nearly half of them had FLE.5 Only 25% of these patients could be selected for surgery; the most common reason for exclusion from surgery was a normal MRI.5 Despite advances in MRI techniques, MRI-negative cases account for 18% to 43% of all presurgically studied patients.6, 7 In the studies that had specifically reported the surgical outcome of FLE patients, the proportion of patients with normal MRI has varied from 25% to 45%.2, 4 Many a time, the quality of the MRI performed may not be satisfactory enough to detect the lesion or the neuroradiologist reading the MRI may not be experienced enough to identify occult lesions. In the Bonn series, among nine “MRI-negative” patients who underwent surgery and had distinct histopathological lesions in the resected specimens, repeat review of their presurgical MRI detected lesions in eight.3
Challenges in the diagnosis of MRI-negative FLE
Frontal lobe is the largest of all lobes in brain, accounting for one-third of the total hemispheric volume. Large areas of frontal lobes, especially the mesial and basal frontal regions, are inaccessible to standard 10–20 system of EEG electrode placement. The extensive connections between the frontal lobes and other lobes through corpus callosum, cortical and subcortical pathways, allow rapid spread of interictal and ictal activity contributing to the low sensitivity and specificity of the scalp EEG. Due to the same reasons, there is a considerable overlap in the semiological characteristics and the EEG patterns of the seizures originating from different frontal regions, making it difficult to distinguish between them. The sensitivity and specificity of various functional imaging techniques like positron emission tomography (PET) and single photon emission computed tomography (SPECT) are lower in FLE than in TLE. The larger areas of frontal lobes often require extensive coverage during invasive EEG studies, which escalates the cost, complications and sampling error. Lastly, the extent of surgical resection is often compromised by the close proximity of the epileptogenic zone to eloquent cortical regions. Because of these reasons, in the absence of a clearly identifiable MRI lesion, reliable localization of the epileptogenic zone in FLE becomes a daunting task that necessitates the need for a multimodal and comprehensive diagnostic evaluation, which often ends up as an expensive and unrewarding undertaking.
Diffrential diagnosis of FLE-like seizures in MRI-negative patients
Unilateral clonic seizures, tonic asymmetric seizures with preserved consciousness, hypermotor seizures and more frequent occurrence during sleep are generally considered to be typical of FLE.8 In patients presenting with FLE-like seizures and normal MRI, a heterogeneous variety of nonepileptic and epileptic paroxysmal events needs to be considered in the differential diagnosis before proceeding with the presurgical evaluation (Table 15.1). Although careful clinical histories can distinguish most of the disorders listed in Table 15.1 from FLE, video-EEG monitoring and/or polysomnography may be required to establish the diagnosis in difficult cases. Psychogenic nonepileptic spells (PNES) is the most frequent condition misdiagnosed as frontal lobe seizures. A very gradual onset and termination, discontinuous, irregular or asynchronous motor activity, side-to-side head shaking, opisthotonic posturing, pseudosleep, stuttering and weeping are characteristics that are strongly associated with PNES.9 Contrary to the usual perception, nocturnal frontal lobe epilepsy (NFLE) is neither a benign nor a homogenous disorder. Familial and sporadic forms of NFLE exist, and both can present with different seizure types, including paroxysmal arousal, nocturnal paroxysmal dystonia and episodic nocturnal wanderings.10 About 30% of patients with NFLE can be resistant to AEDs.10 More than one-third of patients with NFLE present with personal or family history of parasomnias, thereby complicating the differential diagnosis between these two disorders.11 Researchers in Australia demonstrated that clinical history alone can accurately discriminate between NFLE and parasomnias.12 In the test battery they developed, called FLEP scale, while later age at onset, longer duration (> 2 minutes) of events and occurrence during later part of sleep favored parasomnia, stereotypy, clustering and ability to recall were more frequently associated with NFLE.12 A recent study that compared the diagnostic value of FLEP scale against nocturnal polysomnography showed that FLEP scale failed to give a diagnosis of NFLE in only four out of 71 (5.6%) patients.13 The FLE-like seizures might originate in the temporal or parietal lobes or in the insula.10, 14 Hypothalamic hamartoma is known to present as pseudotemporal and pseudofrontal epilepsy syndromes.15 Even an experienced neuroradiologist might fail to identify a small sessile hypothalamic hamartoma in the MRI. Ring chromosome 20 should be suspected in patients with recurrent frontal lobe nonconvulsive status epilepticus and normal MRI.16 Individuals with Ring chromosome 20 syndrome may have normal cognition despite poorly controlled seizures and no dysmorphic features, making the diagnosis difficult unless there is a high index of suspicion.17
Table 15.1 Differential diagnosis of MRI-negative AED-resistant frontal lobe epilepsies
Localization of seizure onset
In patients with FLE-like seizures and normal MRI, an earnest effort should be made to obtain as much clinical and EEG clues as possible that can help to localize the ictal onset to areas within the frontal lobe as depicted in Figure 15.1. Thus, broadly dorsolateral frontal, mesial frontal and basal frontal epilepsies, and several sublobar syndromes within them are recognized (Table 15.2); however, as discussed below, many of them may not be discernible clinically or electrographically.
Figure 15.1 Anatomy diagram showing the localization of important functional areas on the dominant frontal lobe. Clockwise from top: lateral view; inferior (basal) view: and medial view.
Seizure semiology
The video-EEG recorded semiology has severe limitations in localizing origin of seizures to different frontal regions because of rapid spread within the frontal lobe and extrafrontal regions.18 However, seizures originating from a particular area of the frontal lobe more often follow a pattern of propagation and careful analysis of the sequence in which different semiological features occur (cluster analysis) can help in localization of the epileptogenic zone. Based upon such analysis in patients who are rendered seizure-free following restricted resections as well as in patients with well-defined focal MRI lesions, certain seizure semiologies have been correlated with distinct frontal regions, which can be a useful guide while evaluating MRI-negative FLE patients.
General semiological characteristics of frontal lobe seizures
Compared to temporal lobe seizures, frontal lobe seizures are briefer, more frequent, abrupt in onset and offset with minimal postictal confusion, and have a tendency to occur during sleep.8, 19, 20 Similarly, the presence of motor features in the early part of semiology like tonic posturing, clonic jerks and version, generalized tonic or clonic movements with preserved consciousness, motor agitations and bicycling pedal automatisms strongly suggest frontal lobe seizures.19, 20 Even though abdominal auras can rarely occur in FLE, abdominal aura progressing on to hypomotor seizures with oroalimentary automatisms is typical of TLE, which allows its discrimination from FLE.21 On the other hand, certain combinations of features suggest against frontal lobe seizures. These include fear behavior associated with olfactory and gustatory auras, absence seizures without focal features and presence of visual auras.8, 19, 20
Frontal lobe seizure types and their localization significance
Frontal seizures can be divided into six main types: clonic seizures, asymmetrical tonic seizures, complex motor seizures, absence-like seizures, versive seizures and operculoinsular seizures. Clonic motor seizures indicate seizure origin in the contralateral perirolandic area. Due to the close proximity of the sensory area, one-third of patients may have associated somatosensory aura. Depending upon the initial site of activation in the central area, clonic jerks can start in the face, distal upper limb or foot, and then spread to other regions. Asymmetrical tonic seizures in its classical form is associated with abduction at the shoulder along with flexion of elbow and head deviation giving rise to a fencing posture or as if one is looking at one’s own hand.22 Ajmone-Marsan and Ralston23 named this semiology as “M2e sign” corresponding to the secondary motor area in French. The asymmetrical tonic seizures indicate activation of SSMA which may also occur due to spread from mesial parietal, dorsolateral frontal or anterior cingulate cortex. Hypermotor seizures (HMS) are brief, tend to occur during sleep and do not usually generalize. Rheims and colleagues,24 based on seizure semiology and intracranial EEG, distinguished two types of hypermotor seizures: HMS1 characterized by marked agitation that included body rocking, kicking, boxing and associated facial expression of intense fear; and HMS2 characterized by mild agitation that included horizontal body movements or trunk rotation while lying in bed usually associated with tonic/dystonic posturing. While HMS1 had more often a ventromedial frontal cortex epileptogenic zone, HMS2 was more frequently associated with a mesial premotor cortex epileptogenic zone. Frontal absence seizures are the least common type of frontal seizures. They are usually brief, but may be quite prolonged in some patients. The frontal absence seizures have been typically associated with anterior cingulate cortex but may arise from the frontal basal region.25 These areas have rich callosal connections or connections to the thalamus which result in rapid bilateral spread with loss of awareness. Versive seizures are characterized by the forced, either tonic or clonic, eye and head version to the contralateral side, which may be followed by asymmetrical tonic limb posturing and secondarily generalization. Versive phenomena occurring at the onset usually indicate seizure origin from premotor or dorsolateral prefrontal cortex at or near to frontal eye field. The manifestations of operculoinsular seizures include contralateral facial jerks, hypersalivation, mastication, perioral paresthesia and choking sensations along with autonomic features. These seizures subsequently spread to involve other frontal or temporal lobe regions.25
Pure aphasic seizures without any other ictal manifestations occur with the involvement of Broca’s area on the dominant side. Seizures from frontal basal and cingulate cortex can rapidly propagate to temporal lobes and may manifest as pseudotemporal epilepsy.25 Akinetic seizures or motor inhibitory seizures, characterized by the sudden inability to initiate or maintain (negative myoclonus) movements, are rare manifestations of frontal lobe seizures. These are thought to be caused by the activation of negative motor areas which are situated just in front of the motor face area in the inferior frontal gyrus and just in front of the SSMA.26 Gelastic seizures can arise from anterior cingulate cortex, while autonomic seizures with visceral or peripheral autonomic manifestations along with olfactory aura can arise from frontal basal regions.
Scalp EEG in frontal lobe epilepsy
The pitfalls in the interpretation of the scalp EEG in FLE are listed in Table 15.3. Placing additional electrodes within frontal regions as per the 10–5 system may improve localization. In a study of 53 patients from the Mayo Clinic, Rocheter, MN, USA, who were seizure-free after localized frontal resections, Vadlamudi and colleagues27 found that ten (18.9%) patients had no detectable IEDs, five (9.4%) had only extrafrontal or generalized IEDs, while two (3.8%) had only contralateral IEDs. The IEDs restricted to the region of the seizure focus were seen in only 19 (35.8%) patients; whereas an additional 14 (26.4%) had concordant IEDs, but also with generalized or extrafrontal IEDs. Localized IEDs are more common in dorsolateral FLE as compared to mesial or frontal basal epilepsies due to the greater proximity to scalp electrodes (Figure 15.2). In the study by Vadlamudi and colleagues,27 concordant IEDs were seen in 72% of patients with dorsolateral FLE and 33% of those with mesial FLE. Similarly, the localized and fast ictal discharges are also more likely to occur in dorsolateral FLE as compared to other sites (Figure 15.2). Presence of localized interictal or ictal beta activity is one of the most reliable localizing features, and predictor of good seizure outcome, as it indicates proximity to the underlying source.28 On the other hand, mesial FLE either shows bifrontal, generalized or no IEDs (Figure 15.3).29 Patients with epileptogenic zones involving the SSMA have IEDs restricted to the central regions. These patients can also have intermittent rhythmic theta activity over the central region during wakefulness which can provide useful localizing information. Ictal rhythms in mesial FLE usually consist of a diffuse burst attenuation pattern followed by diffuse beta activity which may be more prominent or occur earlier over the central regions. Patients with frontal basal or anterior cingulate epilepsies commonly have unilateral or bilateral temporal IEDs and temporal ictal discharges (Figure 15.4). Finally, ictal rhythms are usually obscured by the myogenic and movement artifacts in patients with hypermotor seizures (Figure 15.3).
Figure 15.2 The imaging and EEG findings in an 18-year-old male with AED-resistant seizures from 5 years of age. The semiology consisted of head version to left side followed by bilateral tonic posturing. (A) MRI axial fluid attenuated inversion recovery (FLAIR) sequence and (B) saggital 3D-FLAIR sequence show left middle frontal gyrus focal cortical dysplasia (FCD) at the bottom of the sulcus; (C) interictal EEG in common average montage shows left fronto-centro-parietal interictal discharges and occasional right centroparietal discharges spreading to right frontal region; (D) ictal EEG shows left frontal focal beta activity at the onset.
Figure 15.3 The imaging and EEG findings in a 27-year-old female with AED-resistant hypermotor seizures from 5 years of age. The semiology consisted of extreme fear followed by vocalization and restlessness lasting for 15 seconds, occurring 3–4 times in a day. (A) MRI coronal fluid attenuated inversion recovery (FLAIR) sequence and (B) sagittal T2W sequence shows right anterior cingulate focal cortical dysplasia (FCD); (C) interictal EEG in common average montage shows generalized spike-wave discharge; (D) ictal EEG shows movement and myogenic artifacts at ictal onset. There was no discernible ictal rhythm during the seizure. Patient underwent lesionectomy and is seizure- and AED-free for the last 4 years. Histopathology revealed type IIB FCD.
Figure 15.4 The imaging and EEG findings in a 26-year-old female from an area endemic for neurocysticercosis having AED-resistant hypomotor seizures from 13 years of age. Semiology was characterized by the behavioral arrest along with bimanual and oral automatisms not preceded by any aura. (A) MRI axial 3D-fluid attenuated inversion recovery (3D-FLAIR) sequence and (B) sagittal proton density sequence shows right frontal–basal calcified lesion with surrounding gliosis; (C) interictal EEG with sphenoidal electrodes shows bilateral anterior temporal spikes; (D) ictal EEG at onset shows 3.5 Hz rhythmic spikes over the right temporal region, which subsequently evolved into 6 Hz rhythmic theta activity over the same region.
Advanced structural imaging in MRI-negative FLE
A careful analysis of the available MRI sequences is one of the most important aspects of the evaluation in patients with apparent MRI-negative FLE, as detection of even subtle abnormality can serve as a substrate for intracranial EEG and improve postoperative outcome. Most of the patients with MRI-negative FLE have focal cortical dysplasia (FCD) as underlying pathology, which is usually located at the bottom of the sulcus, especially in the regions of the F1/F2 sulcus and the F2/F3 sulcus, and a careful image analysis focused at these regions can yield valuable results.30 Other methods to improve the spatial resolution, such as the use of phased-array surface coils combined with higher MRI fields, and the newer sequences like 3D-FLAIR imaging can help in detection and better delineation of subtle abnormalities (Figure 15.5).31 Certain post-processing MRI methods like 3D and curvilinear reformatting, texture analysis and quantification of gray and white matter by voxel-based morphometry can help in identifying the subtle areas of cortical thickness and focal cortical dysplasias. Decrease in N-acetyl aspartate/phosphocreatine–creatine ratio on proton magnetic resonance spectroscopy has been found to correlate with the epileptogenic zone in patients with FLE and can be useful for lateralization purposes.32
Figure 15.5 MRI findings in a patient with AED-resistant right-sided focal motor clonic seizures. (A1) 1.5 T fluid attenuated inversion recovery (FLAIR) axial sequence showing ill-defined hyperintensity over left motor area. This was initially interpreted as normal. The 1.5 T 3D-FLAIR axial sequences (A2 and A3) clearly show focal cortical dysplasia involving left motor area.
PET in MRI-negative FLE
The FDG-PET is probably the most important investigation in patients with MRI-negative FLE as it can provide a substrate for placement of intracranial electrodes. However, there is a relative dearth of data about the sensitivity and specificity of FDG-PET in MRI-negative FLE patients. In a series of 29 patients with FLE who were seizure-free following surgery, 14 had normal MRI. Of these 14 patients, localized PET hypometabolism was found in five (36%) patients, while it provided lateralizing information in another three (21%).33 In another study, FDG-PET provided correct localization in four of 14 (28%) patients with cryptogenic FLE who were seizure-free following surgery.34 In a series of 24 patients with extratemporal lobe epilepsy and either normal MRI or discordant noninvasive data, FDG-PET was negative in nine (37%) patients, provided localizing information in four (17%) patients and showed nonlocalizing (ambiguous, lateralizing or multifocal) hypometabolism in another 11 (46%) patients.35 In spite of the small numbers reported in the literature, it appears that FDG-PET can provide localizing information in one-third of patients with MRI-negative FLE (Figure 15.6). Use of the newer techniques like statistical parametric mapping (SPM) and 3D-stereotactic surface projection (3D-SSP) images can improve the localizing value of FDG-PET, especially in patients with more extensive areas of hypometabolism. Similarly the coregistration of PET scans with MRI can allow the detection of subtle areas of hypometabolism which usually corresponds to type II FCD. In patients with Taylor-type FCD (type IIA-B), which are usually located at the bottom of the sulcus, a recent study has shown that FDG-PET coregistered with MRI could detect hypometabolism in 18 of 23 (78%) patients, which was subsequently found to correlate with the epileptogenic zone.36
Figure 15.6 MRI and FDG-PET findings in a patient with AED-resistant hypermotor seizures. (A1) Normal 1.5 T fluid attenuated inversion recovery (FLAIR) coronal sequence; FDG-PET axial (A2) and coronal (A3) sequences showing hypometabolism involving left basifrontal region extending to dorsolateral region.
Use of other radioligands and receptor-based PET imaging such as 11C-flumazenil (FMZ) PET, alpha-11C-methyl-L-tryptophan (AMT) PET and opioid-based ligands has been studied in a small number of patients. In a study which compared the utility of FDG-PET and FMZ PET in patients with refractory focal epilepsy, FDG-PET was abnormal in five of 11 patients with MRI-negative FLE while FMZ PET was abnormal in six patients.37 However, most of the PET scans utilizing radioligands other than FDG have not been standardized and are available only in a research setting.
SPECT in MRI-negative FLE
Only limited data is available about the utility of ictal SPECT specifically in MRI-negative FLE. Objective evaluation methods like subtraction ictal SPECT coregistered with MRI (SISCOM) and statistical parametric mapping (SPM) are superior to visual interpretation (see Chapter 5 for more information on SPECT). Overall, SISCOM and ictal SPECT evaluated with SPM provide correct localization in 50–60% of patients with extratemporal lobe epilepsy and lateralization in another 20%, although few studies have suggested correct localization rates as high as 90% in patients with FLE.38, 39 Correct localization with SPECT and inclusion of the areas of hyperperfusion detected on SISCOM in the surgical resection have been found to be associated with improved seizure outcomes.38 Importantly, SPECT can provide false localization and lateralization in 10% to 15% of patients with FLE, usually to the ipsilateral temporal lobe, or more commonly with multiple areas of hyperperfusion, the significance of which needs to be determined with regard to other presurgical data.40
Magnetic source imaging
Magnetoencephalography (MEG) combined with source modeling and coregistered to MRI, called magnetic source imaging (MSI), can provide the source localization of the interictal spikes irrespective of the lesion status. The MEG technique is less sensitive in detection of deeper sources and hence its sensitivity is higher in dorsolateral FLE than in mesial or basal FLE. In one of the prospective studies which evaluated the localizing value of MSI, PET and SPECT in relation to the intracranial EEG localization of the epileptogenic zone in 60 patients with nonlocalizing or discordant information on noninvasive studies, MSI had a sensitivity of 64% and specificity of 79%, which were better than those of PET and SPECT.35 All three modalities, however, provided complementary information in different patients. Other studies have also shown that postoperative seizure outcome is better if MSI-identified dipole clusters are included in the resection.41
Electric source imaging
The electric source imaging (ESI) is a source modeling technique which utilizes realistic head models and inverse source estimation methods to detect the source of EEG spikes (see Chapter 7 for more information on ESI). It is a relatively newer method in presurgical evaluation and hence its validity and utility in routine presurgical evaluation is still being investigated. In a large clinical series of 152 operated patients, ESI had a sensitivity of 84% and a specificity of 88% in correctly localizing the epileptogenic zone if the EEG was recorded with a large number of electrodes (128–256 channels) and the individual MRI was used as head model.42
EEG-functional MRI
Spike-related blood oxygen level dependent (BOLD) activation can be used for source localization in difficult cases by simultaneous continuous fMRI with scalp EEG recording (EEG-fMRI). A few small clinical studies have demonstrated its utility in source localization when other conventional tools fail to provide the localizing information. Inclusion of areas of BOLD activation detected by EEG-fMRI within surgical resection has been shown to improve the surgical outcome.43 Newer techniques like analysis of topographic spike maps when there are no spikes during fMRI session and use of ictal EEG-fMRI are being explored to improve its utility (see Chapter 8 for more information).
Functional MRI
Almost all patients with MRI-negative FLE will require intracranial EEG and functional mapping if the mapped epileptogenic zone is at proximity to the eloquent regions. Preoperative language fMRI is important for deciding the hemispheric dominance as many patients with left hemispheric epilepsy may have atypical language lateralization.44 Functional MRI for language and motor functions can be used as an additional tool to minimize the deficits while planning resection.
Multimodlity integration in a single space
The information provided by the different methods of source localization is diverse. The spatial relationship between the localization provided by these different modalities can be better assessed by integrating these modalities in a single 3D space. Thus the areas of PET hypometabolism, SISCOM hyperperfusion, MSI and ESI dipoles, and EEG-fMRI-detected BOLD signals can be coregistered with 3D volumetric MRI images. These can be further supplemented by the inclusion of language and motor activation areas on MRI. Finally, the CT scan showing the position of intracranial electrodes can be coregistered within the same space, which can depict the spatial relationship between the ictal onset zone, irritative zone, important functional areas and localization provided by the different modalities over the anatomical space. The final image can even be included in the neuronavigation system during surgery so as to provide the real-time view of different regions during surgery.
Intracranial EEG
Intracranial EEG remains the gold standard for defining the epileptogenic zone and guiding the final resection in patients with MRI-negative FLE. The strategy of placement and area of coverage is defined by the primary hypothesis regarding the likely epileptogenic zone, which is generated by the careful analysis of all the noninvasive data. Success of any epilepsy surgery depends upon the accurate localization and complete removal of the epileptogenic zone without compromising the important functional areas. All the possible noninvasive resources should be exhausted even at the expense of redundancy before planning the invasive evaluation so as to avoid the sampling error during intracranial EEG. However, the number of tests undertaken and the strategy of intracranial EEG are also determined by the availability of various resources and expertise at the individual center.
The strategy of intracranial coverage should be optimized to detect the ictal onset zone, sites of early propagation and the irritative zones for the localization. The nearby eloquent regions also should be covered for the electrical stimulation and mapping. In the absence of an anatomical landmark in MRI-negative patients, this is usually guided by the results of various functional imaging and other source localization methods. Various studies have shown that the inclusion of the areas defined by the FDG-PET, SISCOM, MSI and EEG-fMRI within the surgical resection tends to improve the outcome.36, 38, 41.43 This often results in the extensive coverage over large areas of the frontal lobes. In patients with uncertain lateralization, sometimes bilateral coverage is required which is associated with lowest rates of success and highest rates of complications, which again underscore the need for the careful analysis of each modality starting from the seizure semiology to detect subtle clues about the laterality. In spite of the extensive coverage, one should be prepared to modify or supplement the coverage during the same setting, if the need arises. Many patients may require multistage intracranial monitoring.
The types of electrodes used vary in different centers according to the philosophy and the available expertise. Using large grids and multiple strips improves the spatial coverage and facilitates functional mapping. However, these may fail to detect or falsely localize the ictal onsets from deeper sources, which are more readily detected by depth electrodes. Hence, the policy of combining depth and subdural electrodes is most likely to give the best possible results. Some centers which routinely perform stereo EEG (SEEG) have reported the best results in nonlesional FLE even with nonlateralized noninvasive data.7 However, the technique of SEEG is complex and expertise is available only in few epilepsy centers in the world.
Management of patients with MRI-negative FLE
A management algorithm for patients with MRI-negative FLE is illustrated in Figure 15.7. Complete seizure control is virtually unachievable even after resective surgery in more than half of patients with MRI-negative FLE, necessitating a very careful discussion through a patient management conference about the pros and cons of surgery. Useful palliation can sometimes be achieved by modification of AED treatment, ketogenic diet and vagus nerve stimulation in those who are not favorable surgical candidates. In this chapter, we shall restrict our discussion to resective surgery.
Figure 15.7 A management algorithm for AED-resistant MRI-negative frontal lobe epilepsy.
Resective surgery
The resection following the intracranial EEG in nonlesional epilepsy is often designed to include the ictal onset zone, early propagation sites and areas showing frequent interictal discharges. The irritative zone is often widespread on intracranial EEG and the removal of all the areas showing spikes is neither warranted nor feasible. However, inclusion of areas showing frequent rhythmic spikes (1/sec) or bursts of fast activity within the surgical resection has shown to improve the postoperative outcome.45 Few studies have also shown that removal of the areas showing persistent slow (delta) waves also improves the outcome.46 An ictal onset confined to three to four electrodes in the form of fast beta activity (30–40 Hz) is the best indicator of a localized onset. However, ictal onset may take the form of rhythmic spike-wave discharges or slower rhythms. Recently, the detection of high-frequency oscillations (HFO) on intracranial EEG in extratemporal epilepsies has been considered as an important advancement in localizing the epileptogenic zone. The areas showing HFO often colocalized with the epileptogenic zone and its removal has been shown to improve the surgical outcome.47 The elicitation of electrographic seizures and after-discharges on cortical stimulation can also provide further localization information. The areas showing after-discharges on single pulse stimulation has been shown to help in detection of ictal onset zone in FLE.48 Due importance should also be given to the localization provided by the noninvasive source localization methods while planning the resection. As far as possible all areas showing abnormalities on different modalities should also be included within the resection without involving eloquent cortex.
Patients might have widespread epileptogenic zones in MRI-negative FLE which can involve large parts of a frontal lobe. Fortunately, large areas of frontal lobe in front of the precentral gyrus can be resected, if required, without producing any major permanent deficits. Originally, four different types of frontal resections were proposed:49 (1) Total frontal lobectomy, which involves resection of the three frontal gyri on the lateral side along with the resection of anterior cingulate gyrus; (2) Paramedian frontal resection, which involves resection from the frontal pole to precentral gyrusi extending laterally to the middle frontal gyrus and medially to include anterior cingulate cortex and SSMA–This may be planned for the seizure originating from medial frontal region including anterior cingulate cortex and SSMA; (3) Frontopolar resection, which includes removal of frontal pole and adjacent basal and lateral frontal cortex–This is usually undertaken for frontobasal and frontopolar epilepsiesi. (4) Central resections, which consist of removal of the lower central areas up to 3 cm above the Sylvian fissure. These anatomically defined resection strategies can help in defining the final resection areas after intracranial EEG. Surgical strategies which make use of the electroclinical characteristics of frontal sublobar syndromes are summarized in Table 15.4.
Table 15.4 Electroclinical characteristics and surgical treatment of frontal sublobar syndromes
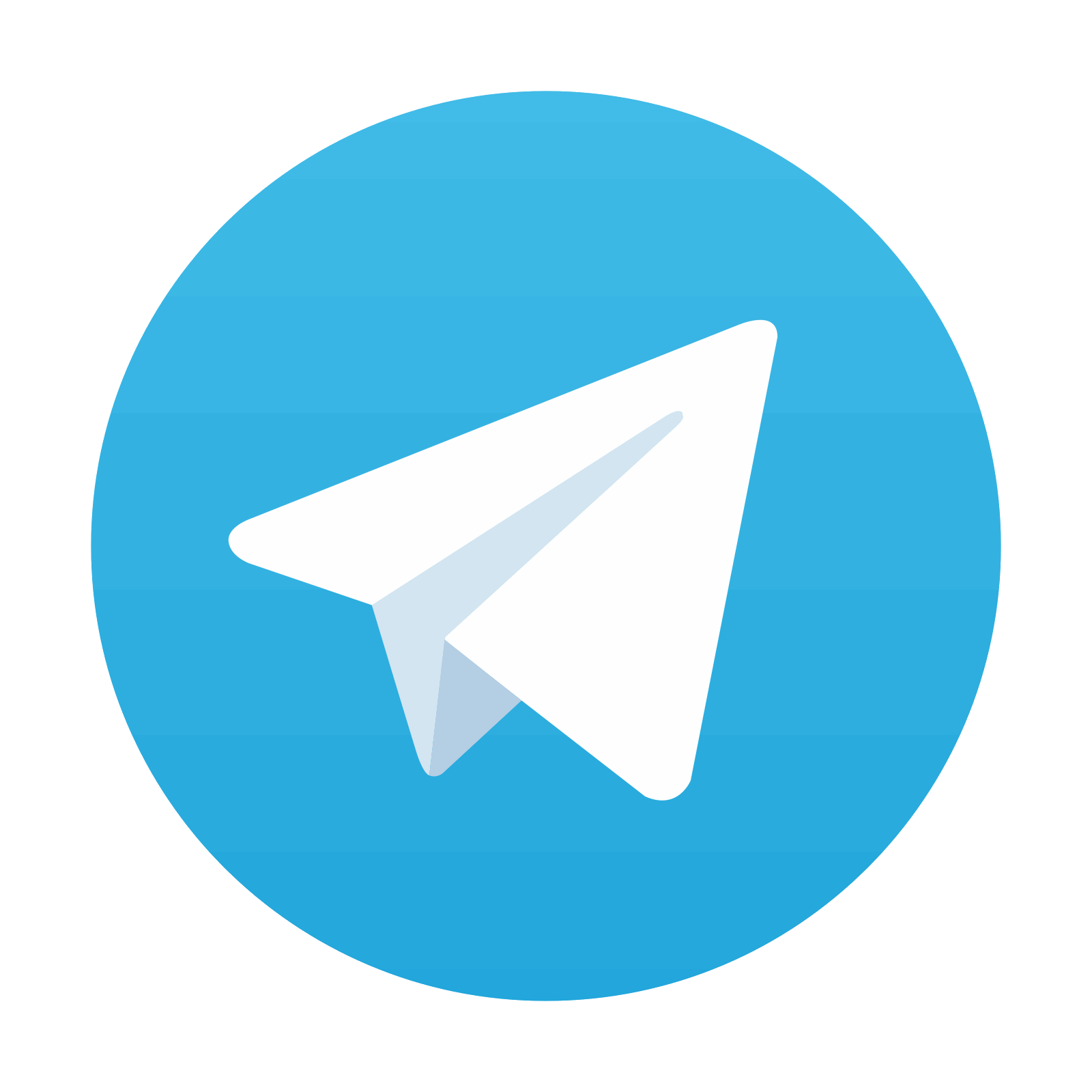
Stay updated, free articles. Join our Telegram channel

Full access? Get Clinical Tree
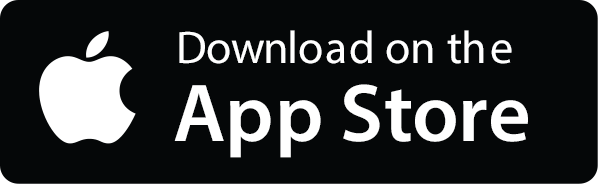
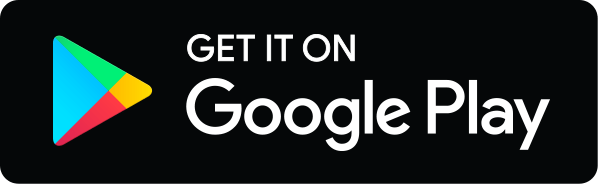
