Chapter 16 Localization and surgery in MRI-negative posterior cortex epilepsies
MRI-Negative Epilepsy, ed. Elson L. So and Philippe Ryvlin. Published by Cambridge University Press. © Cambridge University Press 2015.
Introduction
Posterior cortex epilepsies comprise epilepsies with seizures originating from the occipital lobe, the parietal lobe, the occipital border of the temporal lobe or from any combination of these regions.1, 2 No clear anatomic and neurophysiologic distinctions can be drawn between these cortical areas, and the epileptogenic zones are frequently not restricted to a single lobe.3 Therefore several authors believe that it makes sense to group these patients in a single group of so-called posterior cortex epilepsies,2 while others propose to distinguish between patients with well-defined and circumscribed seizure onset zones within subcompartments of the posterior cortex.4, 5
Posterior cortical resections comprise less than 10% of all epilepsy surgeries.4 Reports on seizure-free outcome largely vary between 25% and 90%.2, 4–20 Several important large series on posterior cortex epilepsy surgery cover time periods before the introduction of high-resolution MRI and therefore bear limitations for conclusions on surgical treatment of MRI-negative epilepsies.1, 6–8,21–23 Most recent studies on epilepsy surgery in the posterior cortex using high- resolution MRI as part of their presurgical evaluation protocol reported exclusively,2, 5, 9–11,24, 25 or predominately, on lesional cases.4, 12–16,26, 27 Therefore the data on epilepsy surgery in MRI-negative posterior cortex epilepsies are rather limited4, 12–20,26, 27 and/or include MRI-negative patients with seizure onset zones in different neocortical regions.28–31
Clinical seizure semiology
Clinical seizure semiology is the initial decisive step for the diagnosis of MRI-negative posterior cortex epilepsies. In general, one can distinguish the early seizure manifestations reflecting epileptic activity in the seizure onset zone within the posterior cortex from subsequent seizure manifestations resulting from seizure spread to the temporal or frontal lobes.6, 7, 22, 23, 32, 33
Occipital lobe seizures
Early seizure manifestations indicating an occipital seizure onset include elementary visual hallucinations, ictal amaurosis, blinking, eye movement sensations and head/eye deviation.32 Elementary visual hallucinations represent the most frequent initial symptom indicating an occipital seizure onset, and occur in 50–70% of patients.5, 6, 16, 18, 23 Elementary visual hallucinations usually consist of multicolored and circular patterns, located unilaterally contralateral to the epileptic focus. These patterns usually multiply and become larger, but without any particular movement other than changing positions and luminance within their visual territory.34 The presence of a visual aura does not correlate with a specific location within the occipital lobes.16 Furthermore, visual auras including elementary hallucinations, illusions and visual loss do not exclusively occur in occipital lobe epilepsy, but also in patients with occipitotemporal and anteromedial temporal seizure onset. On the contrary, complex hallucinations and concentric changes of visual field (tunnel vision) never occur in occipital lobe seizures, but only with occipitotemporal and anteromedial temporal seizure onsets.35
Blinking was observed in 15–56% of patients,6, 18, 23 has a forced quality, may consist of eyelid flutter and occurs after the visual hallucination phase when consciousness is impaired.34
Eye movement sensation without detectable movement and/or nystagmoid eye movements as an initial symptom are thought to reflect seizure activity in the extracalcarine occipital cortex.32 These symptoms were reported in 7–16% of patients.6, 23
Versive eye/head movements occur in 10–50% of occipital lobe epilepsy patients and are always contralateral to the seizure focus.6, 27 In about one-third of patients, versive eye/head movements are not associated with visual auras. Versive eye/head movements are accompanied by focal somatomotor manifestations in less than half of the patients. Therefore they are thought to be generated either by propagation to the frontal eye field and from there to the paramedian pontine reticular formation (PPRF) or by direct propagation to the superior colliculus and subsequent PPRF activation without frontal involvement.27
Based on clinical seizure semiology, it is not possible to distinguish mesial versus lateral occipital lobe seizures.36
Subsequent seizure manifestations resulting from medial inferior spread via occipitotemporal projections produce symptoms typical of medial temporal lobe epilepsy, including visceral sensations, experiential phenomena, loss of consciousness, motionless stare and automatisms, and occur in 34–71% of patients.5, 6, 9, 16, 23, 32 Spread to the temporal neocortex results in complex visual and auditory hallucinations. Medial suprasylvian spread produces asymmetric tonic seizures, whereas lateral suparsylvian spread produces unilateral clonic activity. These spread patterns to the frontal lobe were observed in 12–38% of patients.5, 6, 9, 16, 23 Spread to the contralateral occipital lobe can cause ictal blindness, occurring in 15–40% of patients.6, 18, 23, 32 In 29–56% of patients different seizure patterns occur due to different spread patterns.6, 9, 23, 32
When lesions were localized below an imaginary line drawn at the level of the Sylvian fissure, patients had fewer motor phenomena. Seizures originating from this inferior region seemed to have better chances to propagate to the temporal lobe, whereas seizures emanating from other posterior cortical areas probably have more complex and diffuse propagation patterns, with greater involvement of motor areas.2
Parietal lobe seizures
Early seizure manifestations indicating a parietal seizure onset consist of contralateral somatosensory auras, most commonly described as numbness or tingling sensations, and less frequently as pain, thermal sensations, disturbances of body image including a sensation of movement or spatial displacement of one extremity, twisting or turning sensations, inability to move or feeling of weakness of one extremity.33 However, only less than 25–63% of patients experience somatosensory auras because, except for the primary somatosensory area, the parietal lobe appears to be clinically silent in terms of seizure symptomatology.7, 17, 22, 32
Other auras include aphasia, vertiginous sensations, cephalic sensations and complex visual or auditory hallucinations which are not generated in the seizure onset zone but result from seizure spread.7, 17, 22, 33
Subsequent seizure manifestations are caused by posterior spread to the occipital lobe (ictal amaurosis), by anterior spread to the lateral convexity including sensorimotor cortex (focal clonic activity) and premotor eyefield (version), by anterior medial spread to the supplementary motor area (asymmetric tonic seizures) and to the temporolimbic region (automotor and dialeptic seizures).7, 17, 22, 24, 33 In one study, 61% of patients with tonic posturing had seizure onset zones that had included the superior parietal lobule, suggesting spread to the supplementary motor area; 79% of patients with automatisms had seizure onset zones that had included the inferior parietal lobule, suggesting seizure spread to the temporal lobe.7 Up to 50% of patients experience more than one seizure type, thus indicating different propagation pathways.17
Bartolomei and coworkers37 studied neural networks underlying parietal lobe seizures using stereotaxic EEG. They correlated the degree of epileptogenicity in various subcompartments of the parietal lobe with clinical seizure manifestations. They proposed to classify parietal seizures into four main subgroups with maximal epileptogenicity in the anterior superior parietal lobule corresponding to Brodmann area 5 forming the somatosensory association cortex, in the posterior superior parietal lobule corresponding to Brodmann area 7, in the inferior parietal lobule comprising the angular and supramarginal gyri corresponding roughly to Brodmann areas 39 and 40 and finally the parietal operculum involved in sensory processing. Eighty-eight percent of patients reported at least one aura, with somatosensory auras (35%), vertiginous auras (23.5%), fear (17%) and complex visual hallucinations (17%) being the most frequent subjective seizures symptoms. Somatosensory auras were reported in all four groups, particularly frequent in the operculum group. Vertiginous auras were most frequent in seizures from the superior parietal lobule and fear unexpectedly arose from the posterior superior parietal lobule. Ipsilateral version was the most frequent objective seizure manifestation (64%) and occurred most often in seizures involving the posterior superior parietal lobule. Hyperkinetic behavior (motor agitation), which occurred in 23%, was observed in seizures from inferior parietal regions. Fluchere and coworkers38 reported three patients with seizure onsets in the inferior parietal lobule or the parietal operculum with ipsilateral ictal “hemiballic-like” movements with contralateral dystonia as a localizing symptom pointing towards a posterior seizure origin. Consciousness was impaired in most cases, which probably can be explained by an excess of EEG signal synchrony within parietal associative cortices.39
Problems with clinical seizure semiology in posterior cortex epilepsies
One of the major problems in MRI-negative posterior cortex epilepsies is that 50% of patients do not demonstrate early seizure manifestations pointing towards a posterior seizure onset, with misleading auras or initial seizure manifestations arising from seizure spread to the temporal or frontal lobes.5, 16
Scalp EEG
Interictal scalp EEG in occipital lobe epilepsy
Although interictal scalp EEG is frequently abnormal in occipital epilepsy, its localizing value is limited. Especially, epileptiform discharges on the mesial and basal occipital surface may remain unrecognized on the scalp. Whereas spikes confined to the occipital lobes are infrequent and occur in less than 20% of patients, the most common findings include spikes over the temporo-occipital or posterior temporal regions that are often in a wide and bilateral independent field distribution. Furthermore, secondary bilateral synchrony with a frontal maximum can be seen. Nonspecific abnormalities include focal slowing, decreased alpha, absent or asymmetric photic responses and asymmetric posterior occipital sharp transients of sleep (POSTs).1, 3, 6, 9, 12, 16, 23, 34, 40, 41 No interictal scalp EEG feature is useful to distinguish between mesial and lateral occipital epilepsy.36
Interictal scalp EEG in parietal lobe epilepsy
Interictal spikes restricted to the parietal lobe as the sole interictal epileptiform abnormality represent an exceptional finding. Interictal spikes usually can be recorded from fronto-centro-parietal, parieto-posterior-temporal, fronto-centro-temporal, fronto-temporo-parietal, and posterior head regions or from the entire hemisphere. Spikes can be recorded bilaterally or contralaterally to the seizure focus in up to one-third of patients. Secondary bilateral synchrony with a frontal maximum occurs in up to 30% of patients.1, 3, 7, 13, 22, 24, 33
Ictal scalp EEG in occipital lobe epilepsy
Ictal EEG activity restricted to the occipital lobe can be seen only in 17–30% of patients.6, 9 Ictal EEG patterns over the posterior quadrant occur in 41–43%.16, 42 Temporal and temporo-occipital EEG seizure patterns can be recorded in up to 50% of patients.6 In some series, ictal EEG was nonlocalized, mis-localized to another lobe or mis-lateralized to the contralateral hemisphere in 43–70% of cases.9, 12, 42 Ictal scalp EEG may be useful to distinguish between mesial versus lateral occipital lobe epilepsy. Regional occipital seizure patterns were seen in four (36%) out of 11 patients with laterally originating occipital seizures, but in none of 20 patients with seizures originated mesially.36
Ictal scalp EEG in parietal lobe epilepsy
Whereas regional patterns restricted to the parietal or centroparietal regions can be recorded in a minority of patients, ictal EEG changes more frequently are merely lateralized to the hemisphere ipsilateral to the side of seizure onset without further localizing evidence, or remain nonlocalized. Frequently, ictal EEG patterns become visible only after propagation to the temporal or frontal lobes, suggesting a wrong localization, or after propagation to the contralateral hemisphere, suggesting even a wrong lateralization of the seizure onset zone.7, 13, 17, 22, 24, 42
In conclusion both interictal and ictal scalp EEG are of limited localizing value in posterior cortex epilepsies.
Other noninvasive tests
Because clinical seizure semiology and scalp EEG – especially in MRI-negative patients – can be misleading for the localization of posterior cortex epilepsies, other noninvasive methods are needed for optimal planning of invasive evaluation and surgery. Ictal SPECT proved useful in MRI-negative patients with occipital lobe epilepsy to correctly lateralize and localize the seizure onset zone.26 However, it should be kept in mind that ictal SPECT can also be nonlocalizing, or mis-localizing, by showing temporal hyperperfusion due to rapid seizure spread to the temporal lobe.16 Other potentially valuable noninvasive methods include high-resolution interictal EEG source imaging (ESI),43 FDG-PET coregistered with MRI44 and magnetoencephalography (MEG).45, 46 However, both high-resolution interictal ESI and MEG rely on localization of the irritative zone, which is often widespread and can provide false localizing information in posterior cortex epilepsies. High-resolution ictal ESI might prove more reliable (see Chapter 7).
Invasive evaluation
All patients with MRI-negative posterior cortex epilepsies definitely need an invasive evaluation before resective surgery, in order to delineate the seizure onset zone and map eloquent cortex. Planning of the invasive evaluation needs to take into account all information provided from noninvasive investigations, including clinical semiology, scalp EEG and ictal SPECT. Whether other techniques like PET, high-resolution ESI, MEG or spike-triggered fMRI are useful in this regard needs to be clarified. One important question to address is whether the temporal lobe and insular cortex need to be covered by invasive electrodes. Markers of significant early temporal involvement include temporal type auras such as epigastric rising sensations and déjà vu sensations,15 as well as temporal spikes on scalp EEG.4 Most invasive studies of the posterior cortex have used subdural strip and grid electrodes.4, 5, 11–14,16, 28, 36 However, excellent localization could also be achieved by stereoelectroencephalography.8, 47, 48 Jobst and colleagues16 showed that patients in whom all three surfaces of the occipital lobe were covered by subdural electrodes had a better outcome than those with only limited coverage. A more comprehensive intracranial study will increase the chances to better delineate the epileptogenic zone, and will also facilitate the precise delineation and preservation of eloquent cortex.5, 16 In this respect, a sublobar categorization of the occipital lobe into medial, lateral and basal zones, based on anatomical landmarks, seems to be useful.5
Occipitotemporal epilepsy
Patients with posterior cortex epilepsies frequently show signs of temporal involvement as evidenced from clinical semiology, electrophysiological abnormalities, or both. Olivier and colleagues49 reported a patient with clinical and scalp EEG features indicating both occipital and temporal involvement. On stereotaxically implanted depth electrodes, most seizures started in the occipital lobes, but clinical symptoms only occurred after spread of ictal discharges to the temporal lobe. The patient was rendered seizure-free after temporal lobe resection. Palmini and colleagues48 selected eight patients out of a group of more than 40 with presumed occipital lobe epilepsy when they fulfilled the following discrepant criteria: on the one hand (a) an aura considered to be of occipital origin, (b) an occipital interictal spike focus, (c) an occipital lesion or (d) a combination of all of these; on the other hand all patients showed scalp EEG changes and clinical seizure semiology suggesting temporal involvement. The seizure onset zone could not be localized by scalp EEG. Depth electrodes showed that the seizure onset could be ordered along an occipitotemporal gradient. A consistent occipital lobe seizure onset was seen in patients who had only elementary visual auras. Those patients who had inconsistent or no aura suggesting an occipital lobe onset most often had temporal lobe seizures. However, temporal lobectomy was of limited benefit in the majority of these patients. Aykut-Bingol and Spencer50 described the syndrome of nontumoral occipitotemporal epilepsy in 16 patients who had at least two characteristics indicating occipital lobe involvement (clinical symptoms, interictal spike focus, ictal onset or a lesion on MRI) and one pointing towards temporal lobe involvement (interictal spikes or seizure onset); 69% of patients had neuronal migration disorders. Clinical seizure semiology indicated an occipital lobe seizure onset in 81% of patients. Scalp EEG showed temporal spikes in nine patients and occipital spikes in one patient. On intracranial EEG, the seizure onset zone could be localized to the temporo-occipital junction in 77% of patients. Postoperative seizure control was best with occipital and temporal resections, but a good outcome could be achieved in some patients after occipital resections alone, even with ipsilateral temporal EEG findings. On the contrary, temporal resections uniformly failed to control seizures.
Several recent studies on posterior cortex epilepsies showed that temporal type auras like epigastric rising sensations10, 18, 20 and déjà vu5 were predictors of an unfavorable surgical outcome. Jehi and colleagues4 showed that patients with preoperative ipsilateral temporal spiking were twice as likely to fail surgery compared to patients with no extraposterior (outside of parieto-occipital) spikes. The authors attributed surgical failure in this group to an incomplete resection of a rather large epileptogenic region extending anteriorly to the insula or to the anterior temporal lobe. Indeed, five of 11 patients re-evaluated after seizure recurrence had seizures arising from the temporoparietal junction. Potential pathophysiologic bases underlying these occiptotemporal epilepsies include vascular mechanisms, intrahemispheric occipitotemporal secondary epileptogenesis or an underlying developmental disorder.4, 48, 50
In conclusion posterior cortex epilepsies showing clinical or electrophysiological signs of temporal involvement should undergo comprehensive invasive exploration of the temporal lobe and insula.
Outcome
Seizure-free outcome after posterior cortex epilepsy surgery varied between 25% and 90% in different series.2, 4–20 Jehi and colleagues4 presented a longitudinal study of surgical outcome following posterior cortex epilepsy surgery in 57 patients with a mean follow-up of 3.3 years. However, only two MRI-negative patients were included in this study. The estimated chance of seizure freedom was 68.5% at 1 year, 65.8% between 2 and 5 years and 54.8% at 6 years and beyond. The outcome after occipital (seizure freedom at 5 years: 89%) and parieto-occipital resections (seizure freedom at 5 years: 93%) was better than after parietal resections (seizure freedom at 5 years: 52%), which could be explained by more conservative resections in the parietal lobe. Thus outcome after posterior cortex epilepsy surgery seems to be more favorable than after frontal lobe surgeries.51
Predictors for postoperative seizure control
Several studies tried to identify possible prognostic variables for postoperative seizure control in posterior cortex epilepsies. It should be noted that in all series, only small numbers or single cases of MRI-negative patients were included. Therefore it remains a matter of debate whether the proposed prognostic factors remain valid for MRI-negative patients.
Factors that have been reported to be predictive for favorable postoperative seizure control included early age at epilepsy onset,12 shorter epilepsy duration,2, 12 normal neurological examination prior to surgery,2 lateralizing aura and clinical seizure semiology,10 absence of a temporal-type aura including epigastric rising sensations, déjà vu sensation and abdominal auras,15 no versive head turning unaccompanied by visual aura,15 absence of a history of generalized tonic–clonic seizure,11 absence of ipsilateral temporal spikes on scalp EEG,4 a focal ictal scalp EEG onset,8 complete coverage of all three occipital surfaces on invasive EEG,16 focal interictal intracranial activity,8 complete resection of the irritative spiking zone delineated on invasive EEG,52 complete resection of the ictal onset zone defined by invasive EEG,8 absence of spikes on postresection electrocorticography,6, 7 lobectomy or multilobar resection as opposed to lesionectomy,4 presence of a histopathological lesion,53 low-grade tumor or cortical dysplasia on histological examination,4, 15 and absence of spikes on postoperative EEG.4, 11 However, most of these parameters – except presence of a histopathological lesion – could not be reproduced in other studies.
Neurological complications
One of the major concerns of epilepsy surgery is the avoidance of new neurological deficits caused by the surgical procedure. In posterior cortex epilepsy, potential neurological deficits induced by surgery include visual field deficits, hemisensory syndromes, hemiparesis, Gerstmann syndrome and language deficits.
Occipital resections bear a high risk of creating or worsening a visual field defect. Pre- and postoperative visual field testing is therefore mandatory. In a large series from Binder and colleagues,12 visual field defects were present preoperatively in 36% of patients, whereas 42% suffered new or aggravated visual field defects after the operation. These findings are similar to other reports in the literature.1, 2, 4, 5, 10, 11, 15, 16, 18, 36 Visual function can be compromised by lesioning either the primary visual cortex or the optic radiation.5 Resections of the left ventral occipitotemporal (vOT) area in the vicinity of the occipitotemporal sulcus, which lies between the lateral fusiform gyrus and the medial surface of the posterior inferior temporal gyrus, can result in reading deficits while speech production and comprehension remain intact.54, 55 The fMRI technique and diffusion tensor imaging tractography should be useful to preserve visual function in occipital lobe resections.56, 57 In any case, the high probability of this postoperative neurological deficit and its functional implications need to be discussed frankly with the patient before the surgery.12
In parietal resections, in addition to visual field deficits, hemisensory syndromes, hemiparesis, Gerstmann syndrome and aphasia constitute the main surgical complications.4, 11, 13, 15, 17 Gerstmann syndrome consists of agraphia, acalculia, finger agnosia and right–left disorientation, and results from damage of the dominant angular gyrus, and also of the supramarginal gyrus and the intraparietal sulcus.13,58 In a series of 40 patients, transient postoperative deficits were observed in 30%, whereas permanent deficits were seen in only 7.5% of patients.13 When the epileptogenic zone overlaps with eloquent cortex, additional multiple subpial transections may be an option.13 The safety of parietal lobe surgery can be improved by preoperative fMRI mapping and intraoperative stimulation studies.
Neuropsychology
Studies on systematic neuropsychological testing before and after posterior cortex epilepsy surgeries are scarce. Luerding and colleagues59 performed systematic neuropsychological testing in 28 patients prior to and 6 months after posterior cortical resections. Whereas postoperative verbal intelligence consistently increased, performance intelligence decreased regardless of lesion side, postoperative seizure control or visual field deficits after surgery. In another study, complete occipital lobectomy increased the risk of postoperative decline of the Wechsler intelligence score.15 There is a definite need for specially designed neuropsychological tests corresponding to the specific functions of the cortical areas being considered for resection.59
Reports on MRI-negative posterior cortex epilepsies
Series that included well-documented cases of epilepsy surgeries in MRI-negative posterior cortex epilepsies
Some well-documented illustrative cases of epilepsy surgeries in MRI-negative posterior cortex epilepsies are summarized in the following.
Barba and colleagues8 presented 14 patients with posterior cortex epilepsies including one MRI-negative patient. This patient showed multifocal interictal spikes and a focal seizure onset on scalp EEG. After acute electrocorticography, a right occipital corticectomy rendered the patient seizure-free. Histology showed irregular distribution of neurons and glial cells. The patient suffered from a postsurgical homonymous left hemianopia.
Bien and colleagues53 presented 190 MRI-negative patients who underwent presurgical evaluation, 29 (15%) of them were eventually operated; 11 patients (38%) became seizure-free (45% if including those with auras only). They distinguished between patients with (nine patients) and without (20 patients) a distinct histopathological lesion. Seven of nine histopathologically lesional patients (78%) became seizure-free compared with only four of 20 patients (20%) without histopathological lesions. Three-fifths of the histopathologically nonlesional patients had multifocal or extensive epileptogenic areas. One MRI-negative patient with posterior cortex epilepsy was included in this study. Clinical seizure semiology indicated an occipital or frontomedial seizure onset zone, interictal scalp EEG showed left and right frontocentral spikes, ictal scalp EEG was localized to the right and left frontal lobe, but was almost generalized. Ictal SPECT including SISCOM showed a left occipital hyperperfusion. Depth electrodes showed right and left fronto-parieto-occipital ictal EEG changes. After a left parieto-occipital resection, seizures remained unchanged. A diffuse epileptogenic area was hypothesized postoperatively.
Caicoya and colleagues14 presented 11 patients with occipital lobe epilepsy, seven of whom underwent epilepsy surgery, including one MRI-negative patient. In this patient, seizures started with amaurosis and were then characterized by a temporal semiology, including nonreactivity, stereotypic language, and deviation of the left corner of the mouth. Preoperative visual field perimetry was normal. Interictal scalp EEG showed left occipital spikes and generalized slowing with a left posterior maximum. Ictal scalp EEG was localized to the left occipital region. Intracranial subdural EEG showed a diffuse ictal onset. Histological examination showed displaced neurons in the white matter. The patient became completely seizure-free after a resection guided by electrophysiological findings, and only suffered from a right inferior homonymous quadrantanopia.
Cukiert and colleagues28 studied 16 patients, with medically refractory extratemporal epilepsy and normal or nonlocalizing MRI in ten and six patients, respectively. All patients were evaluated with extensive coverage by subdural electrodes. Invasive ictal EEG showed a focal seizure onset in all patients. Resections were maximized to include both the ictal onset zone and the predominant interictal irritative zone; 13 patients (81.2%) became seizure-free after surgery, the other three patients (18.8%) experienced a more than 90% reduction of seizure frequency. Histology showed gliosis in ten specimens, cortical dysplasia in five and no abnormalities in one case. Their series included six patients with posterior cortex epilepsies (two parietal, one temporo-occipital and three posterior quadrant resections with removal of occipital, parietal and posterior temporal cortex). Three of these six patients had normal MRI scans including one patient with a temporo-occipital resection and two patients with parietal resections. All three patients became seizure-free. Histology showed dysplasia in two and gliosis in one patient.
Jehi and colleagues4 presented 57 patients with posterior cortex epilepsy surgery including three MRI-negative patients (two patients with MRI-negative MCD and one patient with normal histology). Only one patient with MRI-negative MCD became seizure-free. No further details regarding these patients could be derived from the paper.
Jobst and colleagues16 presented 14 patients with occipital lobe epilepsy including three MRI-negative patients; 12 patients including two MRI-negative patients underwent surgery. The first patient experienced an aura with blurry vision and simple visual hallucinations (bright object followed by dark object); subsequently, random but not lateralized body movements, screaming, head deviation to the left, automatisms and altered consciousness were observed. Interictal scalp EEG showed bilateral independent midanterior temporal sharp waves with equal frequency between sides. Ictal scalp EEG showed either right midanterior or posterior temporal rhythmic theta activity, which then became diffuse. Ictal SPECT scans were performed twice, one showed a left medial temporal hyperperfusion, the other a left lateral temporal hyperperfusion. After invasive evaluation with subdural grid electrodes, a small right-sided inferior occipital resection was performed. Histology showed neuronal loss. Seizures were unchanged after the operation (Engel class IV). The other MRI-negative patient experienced an aura with bilateral hand paresthesias, then right hand automatisms, oral automatisms, and head turning to the right accompanied by altered awareness. Interictal scalp EEG showed rare right posterior temporal spikes. On ictal scalp EEG, a right posterior temporal build-up could be observed. Two ictal SPECT scans showed a right posterior temporal hyperperfusion. After invasive evaluation, a right-sided inferior occipital resection plus a hippocampectomy rendered the patient seizure-free. Histology was normal for the occipital specimen, but showed mesial temporal sclerosis. The operation caused a partial visual field defect.
Sturm and colleagues26 presented ictal SPECT and interictal PET data in six patients with occipital lobe epilepsy including two patients with normal MRI. The first patient had bitemporal seizure onsets on scalp EEG. Whereas interictal PET showed bitemporal hypometabolism, conventional ictal SPECT without subtraction showed a unilateral left occipital hyperperfusion. Invasive EEG (subdural strips and bilateral hippocampal depth electrodes) confirmed an occipital seizure onset congruent to hyperperfusion on ictal SPECT. Pathology showed cortical dysplasia. Outcome after a follow-up of 28 months was Engel class III. The second patient also had bitemporal seizure onsets on scalp EEG. On interictal PET, a left temporal hypometabolism could be observed; on ictal SPECT, a left occipital and bitemporal hyperperfusion. Invasive EEG showed a left occipital seizure onset. Pathology again showed cortical dysplasia. Outcome after a follow-up of 32 months was Engel class II.
Series of posterior cortex epilepsy surgeries including MRI-negative patients but without detailed data
Binder and colleagues12 presented two MRI-negative patients in a series of 52 patients with occipital lobe epilepsy. A topectomy was performed in both patients. On histology, one patient showed a scar and the other patient a cortical dysplasia.
Binder and colleagues13 also included two MRI-negative patients in their series of 40 patients with parietal lobe epilepsy. A topectomy guided by intracranial EEG recordings was performed in both patients.
Davis and colleagues15 presented 43 patients who had undergone posterior quadrant epilepsy surgery. Surgical resections were occipital in 18 patients (42%), occipitoparietal in 14 patients (33%), occipitotemporal in six patients (14%) and occipito-temporal-parietal in five patients (12%). The primary sites of resections were occipital in 28 patients (65%), parietal in ten patients (23%) and temporal in five patients (12%). At 1 year follow-up, 22 patients (51.2%) were Engel class I, ten patients (23.3%) Engel class II, five patients (11.7%) Engel class III and six patients (14.0%) Engel class IV. Eight patients (19%) were MRI-negative.
Hong and colleagues29 presented presurgical evaluation and surgical outcome in 41 MRI-negative patients with neocortical epilepsy including seven patients with occipital lobe epilepsy and four with parietal lobe epilepsy. For the whole group, ictal scalp EEG had the highest diagnostic sensitivity for localization of the epileptogenic lobe in patients with a good surgical outcome (69.7% vs. 42.9% for FDG-PET and 33.3% for conventional ictal SPECT). Engel class I outcome could be achieved in 4/7 patients with occipital lobe epilepsy and in 2/4 patients with parietal lobe epilepsy.
Kim and colleagues,17 from the same group as the above, presented a series of 40 patients with parietal lobe epilepsy: 27 patients underwent epilepsy surgery (26 with a follow-up for more than 1 year) including 14 patients with normal MRI; 14 patients (54%) became seizure-free (9/12 [75%] with MRI lesions and 5/14 [35.7%] with normal MRI), while 12 patients had persistent seizures (3/12 [25%] with MRI lesions and 9/14 [64.3%] with normal MRI). The presence of an MRI lesion was a marginally significant predictor for postoperative seizure control.
Lee and colleagues,18 from the same group as the above, presented 26 surgically treated patients with occipital lobe epilepsy including 16 patients with normal or multiple lesions on MRI: 16 patients (61.5%) were rendered seizure-free after the operation (7/10 [70%] with localized MRI lesions; seven with normal MRI and two with multiple lesions on MRI); ten patients had persistent seizures (3/10 [30%] with MRI lesions and 7/16 [43.8%] with normal or multiple lesions on MRI). No significant association could be found between a localized lesion on MRI and postoperative seizure control.
Lee and colleagues30 also presented 89 patients with MRI-negative neocortical epilepsy (35 patients with frontal lobe epilepsy, 31 with neocortical temporal lobe epilepsy, 11 with occipital lobe epilepsy, 11 with parietal lobe epilepsy and one with multifocal epilepsy). A seizure-free outcome was achieved in 42 patients (47.2%) of the whole cohort, in 7/11 patients (63.6%) with occipital lobe epilepsy and in 3/11 patients (27.3%) with parietal lobe epilepsy. Diagnostic sensitivities (defined as localization concordant with the resected lobe) of interictal EEG, ictal scalp EEG, FDG-PET and subtraction ictal SPECT were 37.1%, 70.8%, 44.3% and 41.1%, respectively. Localizing values of individual modalities for seizure-free patients were as follows: interictal EEG – whole cohort 20/42, occipital lobe epilepsy 4/7, parietal lobe epilepsy 0/3; ictal scalp EEG – whole cohort 33/42, occipital lobe epilepsy 7/7, parietal lobe epilepsy 1/3; FDG-PET – whole cohort 23/40, occipital lobe epilepsy 4/7, parietal lobe epilepsy 0/3. Localizations by FDG-PET and interictal EEG were significantly associated with a seizure-free outcome, whereas localization by ictal EEG and subtraction ictal SPECT were not. Surgical outcome was not influenced by whether invasive ictal EEG onsets were focal or regional or by the frequency of invasive ictal EEG patterns. Concordance between two or more presurgical evaluation modalities was significantly related to a seizure-free outcome. Pathology specimens were available for 80 patients showing cortical dysplasia including microdysgenesis in 58 cases, migration abnormalities in ten, focal neuronal loss with gliosis in nine, ischemic change in two and cortical dysplasia associated with dysembryoplastic neuroepithelial tumor in one patient. Pathology results were not related to surgical outcome.
McGonigal and colleagues47 presented a series of 100 patients who had undergone SEEG evaluation including 43 patients with normal MRI and 57 with lesions on MRI. Their series also included five MRI-negative patients with posterior cortex epilepsies (three with occipital lobe epilepsy, one with parietal lobe epilepsy and one with temporo-parieto-occipital junction epilepsy). All three patients with occipital lobe epilepsy underwent corticectomy. Histology showed dysplasia in one patient rendered seizure-free and gliosis in two patients with persistent seizures. Gamma knife surgery was performed in the patient with parietal epilepsy with no outcome available yet. Surgery was offered, but declined by the patient with temporo-parieto-occipital junction epilepsy.
Urbach and colleagues19 reported 42 patients who had undergone epilepsy surgery in the parietal and occipital lobe. Two MRI-negative patients were operated on electrophysiological basis. Histopathology was unrevealing, and both patients continued to have seizures.
Yu and colleagues20 presented 43 patients with posterior cortex epilepsies including 11 patients with parietal lobe epilepsies, 13 with occipital lobe epilepsies and 19 with seizures originating from the parieto-occipito-posterior-temporal cortex. A favorable outcome could be achieved in 31 patients (72.1%) (Engel class I in 26 cases [60.5%] and Engel class II in 5 cases [11.6%]); MRI showed focal epileptogenic lesions in 24 patients, MRI was nonlocalizing in 19 patients including 13 MRI-negative patients, two patients showed bilateral posterior cortical lesions, and four patients showed lesions in the contralateral hemisphere or in the frontal or temporal lobe which were considered as incidental and unrelated to seizures. A favorable outcome (Engel class I or II) could be achieved in 14/19 cases (73.7%), which was comparable to patients with MRI-visible lesions in the resected posterior cortex (17/24 patients [70.8%] with favorable outcome).
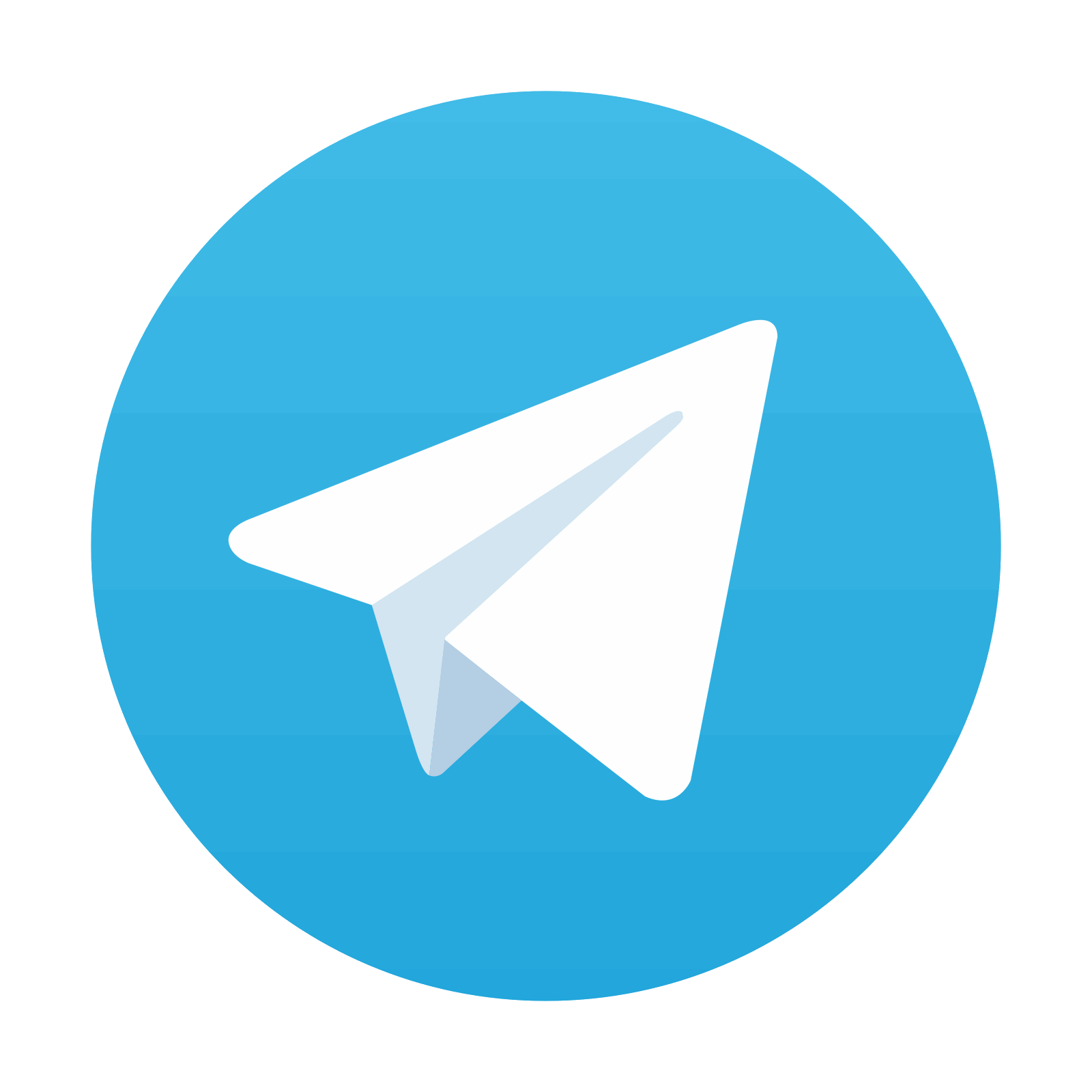
Stay updated, free articles. Join our Telegram channel

Full access? Get Clinical Tree
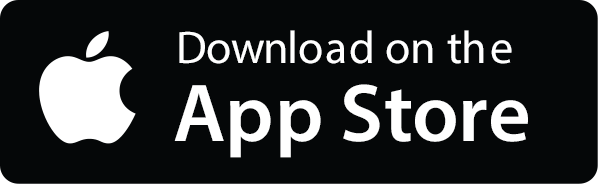
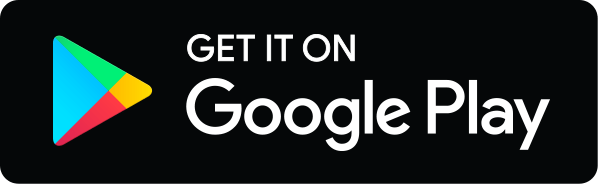
