Abstract
The syndromes of neurodegeneration with brain iron accumulation (NBIA) are a group of disorders defined by progressive hypo- and/or hyperkinetic movement disorders and excessive iron deposition in the brain [1]. Iron predominantly accumulates in (and around) the basal ganglia, mainly the globus pallidus, and can be detected as hypointensity on T2-weighted images due to shortening effects on relaxation time. Brain pathology demonstrates degeneration of neurons and astrocytes. Eosinophilic, roundish swellings containing degenerate organelles (i.e. spheroid bodies) are also common in many subtypes of NBIA. Several genes associated with NBIA disorders have been identified (including PANK2, PLA2G6, WDR45, C19orf12, FA2H, ATP13A2, COASY, FTL1, CP, and DCAF17).
Introduction to NBIA Disorders
The syndromes of neurodegeneration with brain iron accumulation (NBIA) are a group of disorders defined by progressive hypo- and/or hyperkinetic movement disorders and excessive iron deposition in the brain [1]. Iron predominantly accumulates in (and around) the basal ganglia, mainly the globus pallidus, and can be detected as hypointensity on T2-weighted images due to shortening effects on relaxation time. Brain pathology demonstrates degeneration of neurons and astrocytes. Eosinophilic, roundish swellings containing degenerate organelles (i.e. spheroid bodies) are also common in many subtypes of NBIA. Several genes associated with NBIA disorders have been identified (including PANK2, PLA2G6, WDR45, C19orf12, FA2H, ATP13A2, COASY, FTL1, CP, and DCAF17). These genes and their proteins play a role in mitochondrial function, lipid metabolism, and autophagy. The terminology of the syndromes associated with these genes follows a pattern in that the first word(s) (or letters in the abbreviated name) refer to the molecular substrate and the last two words read “associated neurodegeneration,” e.g. pantothenate kinase-associated neurodegeneration (PKAN), PLA2G6-associated neurodegeneration (PLAN), and so on. The recognized clinical spectrum (which is often age-dependent) and molecular understanding continue to evolve and the first controlled clinical treatment trials are underway. Here we present the main clinical features including useful clinical rating scales, genetic mechanisms, biomarkers, pathology, and therapeutic approaches of the most prevalent forms of NBIA (Table 16.1).
Condition (Acronym) | Synonym | Gene | Chromosomal position | LB pathology | Childhood-onset variant | Late-onset variant | ||
---|---|---|---|---|---|---|---|---|
Age of onset | Clinical presentation | Age of onset | Clinical presentation | |||||
PKAN | NBIA1 | PANK2 | 20p13 | No | Early childhood, around age 3 years | Typical PKAN | Teens or early adulthood | Atypical PKAN |
PLAN | NBIA2, PARK14 | PLA2G6 | 22q12 | √ | Infancy | Infantile neuroaxonal dystrophy | Teens or early adulthood | Dystonia parkinsonism |
FAHN | SPG35 | FA2H | 16q23 | Not known | Childhood | Leukodystrophy, hereditary spastic paraplegia | ||
MPAN | – | C19orf12 | 19q12 | √ | 11 (range, 4–30 years) | Pyramidal extrapyramidal syndrome | ||
Kufor–Rakeb syndrome | PARK9 | ATP13A2 | 1p36 | (√) | Childhood- teenage | Parkinsonism, pyramidal-tract signs, eye movement disorder | ||
BPAN | SENDA syndrome | WDR45 | Xp11.23 | Not known | Childhood | Encephalopathy with psychomotor regression, then static | Then: 20s to 30s | Sudden onset progressive dystonia parkinsonism |
Aceruloplasminemia | – | CP | 3q23 | No | – | – | 50s (range, 16–70 years) | Extrapyramidal, diabetes, dementia |
Neuroferritinopathy | – | FTL1 | 19q13 | No | – | – | 40s | Chorea, dystonia, dementia |
Idiopathic late-onset cases | – | Probably heterogeneous | Probably heterogeneous | Heterogeneous | – | – | Heterogeneous | Parkinsonism, in some resembling idiopathic PD |
Abbreviations: BPAN, beta-propeller protein-associated neurodegeneration; CP, ceruloplasmin; FA2H, fatty acid 2-hydroxylase; FTL1 ferritin light chain 1; MPAN, mitochondrial membrane-associated neurodegeneration; PANK2, pantothenate kinase 2; PKAN, pantothenate kinase-associated neurodegeneration; PLA2G6, phospholipase A2; PLAN, PLA2G6-associated neurodegeneration; SENDA, static encephalopathy of childhood with neurodegeneration in adulthood; SPG, spastic paraplegia; √ = present.
Clinical Phenotypes
Common Clinical Features of NBIA Syndromes
NBIA disorders are complex multisystem disorders, making the diagnosis challenging. Symptoms may be non-specific in early disease phases and consist, for example, of global development delay or delayed motor development, prior to onset of more specific signs.
Pyramidal and extrapyramidal features are core symptoms. Movement disorders manifest mainly with dystonia and parkinsonian signs (rigidity and loss of postural reflexes), but also chorea. Furthermore, cognitive decline is a core feature of these disorders. Psychiatric features such as obsessive–compulsive disorder and behavioral disturbances may be present. Autonomic involvement is also common. Notably, for some of the disorders an age-dependent phenotype has been recognized (see below).
The disease course is invariably progressive and patients lose the ability to walk usually within 10–15 years after onset. Premature death usually occurs from secondary complications or due to episodes of status dystonicus.
In most forms, NBIA disorders have an early onset and their inheritance is autosomal-recessive. Exceptions (e.g. neuroferritinopathy and aceruloplasminemia) are outlined below.
Specific Features of NBIA Syndromes
Pantothenate Kinase-Associated Neurodegeneration Caused by PANK2 Mutations (NBIA type 1)
The most common among the NBIA disorders is pantothenate kinase-associated neurodegeneration (PKAN), due to mutations in the PANK2 gene. The worldwide prevalence has been estimated at 1 in 1,000,000. The classic presentation is relatively homogeneous, with onset usually before age 6 years of a gait disorder due to dystonia and rigidity of legs [2, 3]. Oromandibular dystonia is often prominent. Pigmentary retinal degeneration, often detected by electroretinography, is almost invariably present in classic PKAN [4]. There is a well-known correlation between the age of onset and the rate of disease progression. Onset may, however, also occur late, i.e. in early adulthood, as atypical late-onset PKAN. Here, cognitive decline and psychiatric features are often the leading symptoms whereas motor involvement tends to be less severe. Movement disorders often present as unilateral dystonic tremor or focal arm dystonia [2, 5–11].
PLA2G6-Associated Neurodegeneration Caused by PLA2G6 Mutations (NBIA Type 2)
The second core NBIA syndrome is PLA2G6-associated neurodegeneration (PLAN) due to PLA2G6 gene mutations (NBIA type 2). The classic form begins early, between 6 months and 2 years of age as infantile neuroaxonal dystrophy (INAD). Progressive motor deterioration often leads to spastic or hypotonic tetraparesis with marked truncal hypotonia. Optic atrophy causes vision impairment. Seizures may occur at later stages of the disease, and dystonia has been reported in patients with long disease duration [12]. Denervation on EMG and fast rhythms on EEG are the typical electrophysiological findings that may facilitate early diagnosis [13]. In patients with later disease onset the phenotype may be atypical (atypical neuroaxonal dystrophy) and includes dystonia–parkinsonism combined with pyramidal signs, cerebellar ataxia, eye movement abnormalities, cognitive decline, and psychiatric features. Parkinsonism is characterized by the presence of tremor, i.e. a typical pill-rolling resting tremor, rigidity, and severe bradykinesia with a good response to levodopa. The latter may be in line with the finding of Lewy body pathology [14–16]. Early development of dyskinesias occurs. Development of Parkinson disease in siblings with heterozygous PLA2G6 mutations has been reported.
Beta-Propeller Protein-Associated Neurodegeneration Caused by WDR45 Mutations
Mutations in WDR45 cause beta-propeller protein-associated neurodegeneration (BPAN). The clinical presentation was initially summarized under the term “static encephalopathy (of childhood) with neurodegeneration in adulthood” (SENDA syndrome) [17–19]. Onset is in early childhood, with developmental delay and intellectual disability, which remain relatively static until adulthood. In early adulthood, affected individuals usually develop sudden-onset progressive dystonia–parkinsonism and dementia. The clinical spectrum further includes seizures, ataxia, and behavioral problems that are often similar to autism spectrum disorder. Seizure types are diverse and include focal and generalized seizures (absence, tonic, atonic, tonic–clonic, and myoclonic), infantile spasms, and epileptic encephalopathies consistent with Lennox Gastaut syndrome. Overall, seizures tend to resolve or become less prominent with age, whereas cognitive decline and movement disorders (progressive parkinsonism and dystonia) emerge as characteristic findings. Additional features include eye movement abnormalities, sleep disorders, frontal release signs, and dysautonomia.
Mitochondrial Membrane Protein-Associated Neurodegeneration Caused by C19orf12 Mutations
Mutations in C19orf12 at chromosome 19q12 cause mitochondrial membrane protein-associated neurodegeneration (MPAN) [20]. Due to a founder effect, MPAN is more common in the Polish community. Onset is in childhood to early adulthood (age range 4–30 years), with a mean of 11 years. Spastic para- or tetraparesis with muscle atrophy are typical for MPAN. Dysarthria, parkinsonism, and dystonia are very common but not present in all cases. Optic atrophy, cognitive decline, and psychiatric symptoms may occur [20–27]. Notably, a mild phenotype resembling idiopathic Parkinson disease has been reported.
Other NBIA Genes (FA2H, ATP13A2, COASY, FTL1, CP, DCAF17, SCP2, and GTPBP2)
FA2H mutations were recently identified as another cause of NBIA, FAHN [28]. The clinical spectrum overlaps with leukodystrophies and the hereditary spastic paraplegias. The clinical phenotype of FAHN is characterized by childhood-onset gait impairment, spastic quadriparesis, severe ataxia, and dystonia. Seizures and divergent strabismus may also be present and overlap with the clinical features of PLAN.
Mutations in ATP13A2 located on chromosome 1p cause Kufor–Rakeb syndrome. The clinical phenotype comprises levodopa-sensitive parkinsonism, pyramidal-tract signs, eye movement abnormalities with incomplete supranuclear upgaze palsy, facial-faucial (i.e. tonsillar)-finger mini-myoclonus, autonomic dysfunction, psychiatric features, and dementia. Disease onset is usually in adolescence [29–31].
COASY-associated neurodegeneration (CoPAN) due to mutations in the coenzyme A (CoA) synthase (COASY) gene is a form of NBIA metabolically closely related to PKAN [32]. It also presents with early-onset gait difficulty and learning disabilities, followed in puberty by bradykinesia, generalized dystonia, and spastic–dystonic tetraparesis with distal areflexia due to motor axonal neuropathy. Notably, there is no retinopathy, in contrast to PKAN and some of the other NBIA syndromes.
Neuroferritinopathy due to FTL1 gene mutations is often thought of as one of the Huntington-like disorders because of adult-onset chorea, psychiatric features, and cognitive decline. Inheritance is autosomal-dominant. However, other features such as orolingual–mandibular dyskinesia, blepharospasm, cerebellar ataxia, and parkinsonism (even at onset) may be present. As in Huntington disease, pyramidal involvement is usually absent.
Aceruloplasminemia also presents in adulthood. Core neurological features are cognitive impairment, cerebellar ataxia, and craniofacial dyskinesia. Notably, a recent case series involving 55 cases revealed that diabetes was the first symptom in almost 70% of patients, manifesting at a median age of 38 years, often accompanied by microcytic or normocytic anemia. The combination preceded neurological symptoms in almost 90% of the neurologically symptomatic patients by more than ten years [33]. Ophthalmological examination often reveals peripheral retinal degeneration secondary to iron accumulation and photoreceptor cell loss. Systemic iron accumulation also leads to increased liver iron content (without cirrhosis).
Mutations in the DCAF17 gene cause Woodhouse–Sakati syndrome, a rare autosomal-recessive multisystem neuroendocrine disorder characterized by hypogonadism, childhood-onset hair thinning that often progresses to alopecia totalis, diabetes mellitus, deafness, cognitive decline, and extrapyramidal features. Seizures, polyneuropathy, thyroid dysfunction, keratoconus, and syndactyly of hands or feet may also be present [34]. Some 30 families have been described, mostly from Middle Eastern countries. Insulin-like growth factor 1 is usually low; ECG may be abnormal, albeit patients are usually asymptomatic.
Bi-allelic mutations in SCP2, encoding the peroxisomal enzyme sterol carrier protein x (SCPx), have recently been described to cause NBIA in two unrelated patients [35, 36] The first patient presented with torticollis and dystonic head tremor, mild cerebellar signs, hyposmia, and azoospermia with motor neuropathy, with onset in late childhood. The other presented with adult-onset spinocerebellar ataxia and deafness, with brain MRI characteristic of NBIA, in the absence of movement disorders or myopathy. Abnormal pristanic acid and phytanic acid levels may be a clue. Dietary therapy, similar to the diet recommended for Refsum disease, led to improvement.
Finally, there is a recent report of GTPBP2 mutations associated with NBIA [37] in a consanguineous family with three affected children. All had delayed early developmental milestones with moderate intellectual disability. Upon presentation at ages 34, 30, and 29 years, respectively, there was dystonia, mild to moderate ataxia, and autonomic dysfunction including skin thickening, mottling, and loss of hair on the legs. Investigations demonstrated chronic motor neuronopathy and abnormal electroretinography.
Clinical Rating Scales
International validated rating scales, mainly for dystonia and parkinsonism, have been used in case series of PKAN patients in an attempt to capture clinical improvement after deep brain stimulation or iron chelating therapy. The scales used in these studies were the Burke–Fahn–Marsden Dystonia Rating Scale (BFMDRS), Unified Parkinson Disease Rating Scale (UPDRS), International Cooperative Ataxia Rating Scale (ICARS), and Unified Dystonia Rating Scale (UDRS). However, PKAN patients show an overlapping dystonia–parkinsonism syndrome that cannot be easily differentiated, as well as other motor abnormalities (i.e. chorea, pyramidal signs, or abnormal ocular movements) and neuropsychiatric disturbances (cognitive decline, behavioral abnormalities) that are not always recorded in the previously mentioned scales. As a consequence, there is a lack of specific validated instruments to measure the functional impairment and the rate of disease progression in a multidimensional and comparable manner, and we do not know yet which symptoms contribute the most to functional disability in PKAN.
A pilot study for a disease-specific clinical rating scale – the PKAN diseases rating scale (PKAN-DRS) –was recently developed for patients with PKAN [38]. The scale included 34 items (maximal score, 135) encompassing six subscales for cognition, behavior, disability, parkinsonism, dystonia, and other neurological signs. Forty-seven patients aged 6–77 years were examined with the PKAN-DRS. Dystonia and parkinsonism, core features of PKAN, were present in all cases and had a significant impact on the patients’ ability to perform activities of daily living. Disability, dystonia, and other neurological signs were more severe in patients with earlier onset of disease, whereas parkinsonian features were more common in older patients with longer disease duration.
The disability questionnaire of the scale also showed that dysarthria and dysphagia impaired or prevented speech and swallowing in most patients, and half of PKAN patients either could not walk at all or needed a walking device or assistance. The disease also showed a strong impact on school attendance and academic abilities in childhood, and later prevented patients from engaging in employment in adulthood. Finally, the analysis of the properties of the scale itself demonstrated that the PKAN-DRS is a reliable and valid instrument for the assessment of pediatric and adult patients with PKAN.
More recently, the PKAN-activity of daily living (PKAN-ADL) rating scale has been developed as a novel patient-reported clinical outcomes measure. It assesses 12 domains of ADLs using single-item questions about difficulty with speech, drooling, swallowing, writing, eating tasks, dressing, personal-hygiene tasks, turning or changing position in bed, sitting, falling, walking, and discomfort or pain [39]. The PKAN-ADL scale was developed as the primary efficacy outcome in the recently completed phase 3 trial, randomized, double-blinded, placebo-controlled pivotal trial of fosmetpantotenate in adult and pediatric patients with PKAN (NCT03041116). For other forms of NBIA, no clinical scales capable of monitoring disease progression have been developed.
Neuroimaging
MRI Techniques
Brain MRI imaging is often the key diagnostic test toward a diagnosis of an NBIA disorder [40]. T2*-weighted and susceptibility-weighted imaging (SWI) images are particularly sensitive to detect iron and confirm the distribution of iron on histological studies with the presence of both antiferromagnetic iron (ferritin) and ferrimagnetic iron.
Iron accumulation characteristically affects the globus pallidus. It may also extend to adjacent areas, which may be somewhat helpful during the work-up. However, it may not be possible to come to a final diagnosis based on imaging patterns alone as there is radiological overlap and radiological variability. Furthermore, the development of MRI findings in NBIA patients appears to be a dynamic process [3, 41]; imaging abnormalities may precede the development of clinical signs (i.e. in asymptomatic carriers of homozygous mutations), while in others iron may be absent in early disease stages or may alter over time. Thus, if the initial MRI is unremarkable, repeated MRI may be useful. Furthermore, the areas affected by iron deposition do not necessarily correlate with the clinical syndrome. Nevertheless, for some NBIA subforms, distinct imaging patterns have been recognized.
Specific MRI Patterns of NBIA Genes
Imaging Findings in PKAN
MRI [42, 43] with appropriate iron sensitive T2*-weighted or SWI sequences detects the characteristic “eye of the tiger” sign, corresponding to a hyperintensity surrounded by an hypointense rim. The latter is produced by iron accumulation in the anterior-medial part of the globus pallidus, sometimes extending into the knee of the internal capsule, subthalamic nucleus, and substantia nigra [41, 44]. Additional calcifications in the basal ganglia have been described in some cases. As mentioned above, the development of the MRI alterations may be dynamic, in some preceding the development of clinical signs, or it may rarely be absent despite clinical signs in others [6, 41, 45, 46].
Using diffusion tensor imaging, increased fractional anisotropy along with abnormal mean diffusivity was demonstrated in the globus pallidus and substantia nigra of PKAN patients, probably due to iron deposits disturbing the local magnetic field [41, 47]. Proton MRS is not consistent but sometimes shows markedly decreased N-acetylaspartate in the globus pallidus, reflecting neuronal damage. Dopamine transporter single-photon emission CT imaging, a measure of striatal dopamine function, is generally normal in PKAN, although abnormal findings have been reported in line with the clinical experience that PKAN may manifest as parkinsonism. Cardiac 123I-meta-iodobenzylguanidine imaging, which is used to assess postganglionic neuronal function of the sympathetic nervous system, was also normal in PKAN, in contrast to Parkinson disease and other Lewy body disorders, where uptake is typically reduced. Transcranial sonography demonstrates bilateral hyperechogenicity in the substantia nigra and lenticular nucleus. It was thus suggested that transcranial sonography may be used as an inexpensive and simple screening method for the diagnosis of NBIA disorders.
Imaging Findings in PLAN
Neuroimaging in the PLA2G6-associated form (PLAN, NBIA2) shows cerebellar atrophy occurring in the early stages of INAD, but this was absent in late-onset disease. Although half of INAD patients may lack signs of iron accumulation early in the disease course [13], they usually develop globus pallidus hypointensities reflecting iron as the disease progresses [12]. Notably, in contrast to the pattern seen in PKAN there is no central hyperintensity. Additional iron deposits in the substantia nigra may be present [14]. Contrary to PKAN, iron accumulation is not a universal feature of PLAN. Late-onset cases may lack signs of iron accumulation and MRI may even be completely normal. Others may show cortical atrophy or white matter changes. Thus, not all forms of PLA2G6-related neurodegeneration fall into the group of NBIA disorders but there is neuroradiological variability.
Imaging Findings in BPAN (WDR45-Associated NBIA)
Imaging features highly characteristic of BPAN are (a) iron deposition is more marked in the substantia nigra than in the globus pallidus in T2-weighted images, and (b) the hypointensity of the substantia nigra is surrounded by a halo of hyperintensity in T1-weighted images [17, 48]. Furthermore, cerebral and milder cerebellar atrophy may be seen.
Imaging Findings in MPAN (C19orf12-Associated NBIA)
In MPAN, iron deposition extends beyond the pallidal region involving the substantia nigra. Hyperintense streaking of the medial medullary lamina between the globus pallidus interna and externa (which may resemble the eye of the tiger sign) may be present in 20% of patients. Generalized brain atrophy or cerebellar atrophy may be present. Transcranial sonography in MPAN patients demonstrates bilateral hyperechogenicity of the lenticular nucleus in the absence of nigral abnormalities.
Imaging Findings in Other NBIA forms (FA2H, ATP13A2, COASY, FTL1, CP, and DCAF17)
In FAHN, MRI features include progressive leukoencephalopathy with cortical, cerebellar, and brainstem atrophy – in addition to pallidal iron accumulation. Not all patients with FAHN, however, show iron accumulation. Corpus callosum thinning may be a diagnostic clue.
Brain imaging in patients with Kufor–Rakeb syndrome may show diffuse moderate generalized atrophy, with iron accumulation affecting the putamen and sometimes the caudate.
Brain imaging in CoPAN revealed bilateral hyperintensity and swelling of the caudate nucleus, putamen, and thalamus. In addition, a thin corpus callosum and frontotemporal and parietal white matter changes have been described.
Hypointensities are more widespread in neuroferritinopathy. Here, iron deposition extends from the globus pallidus to the nigra, cortex, and other nuclei of the basal ganglia. In addition, cavitations depicted as low T1 and high T2 signals, sometimes surrounded by a rim of T2 hypointensity, are found. Scans may also show generalized cortical and, in some cases, cerebellar atrophy. Furthermore, pencil lining, reflecting pathological iron deposition in the periphery of the cortex and other gray matter structures may be a clue toward the diagnosis of neuroferritinopathy. Finally, imaging changes may also be present in asymptomatic gene mutation carriers.
Iron also accumulates widely in aceruloplasminemia, affecting the caudate nucleus, putamen and pallidum, and thalamus, as well as the red nucleus and dentate. Cerebellar atrophy may also be seen.
In Woodhouse–Sakati syndrome, MRI reveals variable abnormalities in periventricular white matter. In addition, iron deposition in the globus pallidus may, rarely, be present.
In SCP2-associated neurodegeneration, MRI brain showed bilateral hyperintense signals in the thalamus, butterfly-like lesions in the pons, and lesions in the occipital region, without gadolinium enhancement, in one patient. There was no iron in this case. However, the second patient showed mineral deposition in the basal ganglia on SWI sequences [35, 36].
In GTPBP2–associated neurodegeneration, brain MRI shows atrophy of the cerebellar vermis. SWI demonstrates abnormal signal (hypointensity) in the globus pallidus and substantia nigra suggestive of abnormal iron deposition [37].
Differential Diagnosis
The clinical phenotype of NBIA syndromes is usually complex, involving numerous systems (extrapyramidal, pyramidal tract, cortical, cerebellum). Algorhithmic thinking may help when evaluating these patients (Figure 16.1). However, the differential diagnosis is broad, including various other inborn errors of metabolism, e.g. lysosomal storage diseases, hereditary spastic paraplegias, and other forms of dystonia–parkinsonism [50]. Radiologically, iron deposition may also be found in other non-NBIA disorders, including Parkinson disease (mainly affecting the substantia nigra) and atypical parkinsonian disorders, Friedreich ataxia, and multiple sclerosis [51]. This list continues to expand with improved high-resolution imaging (i.e. 7-Tesla MRI). On the other hand, the absence of iron in early disease phases may lead to false diagnoses (i.e. recessive ataxia in PLAN) or diagnostic delay. Sequential brain MRI may uncover NBIA syndromes and facilitate the diagnostic approach.
Genetics
Ten NBIA genes are accepted (PANK2, PLA2G6, C19orf12, WDR45, FA2H, ATP13A2, FTL1, CP, DCAF17, and COASY) and, more recently, two additional genes have been taken into account, SCP2 and GTBP2 [52, 53]. However, in a substantial number of patients, the disease-causing mutation remains elusive, suggesting that further NBIA genes remain to be characterized.
NBIA Genes
The most common condition is PKAN, which ranges from 35% to 50%, followed by PLAN, with approximately 20% of patients. PKAN is caused by mutations in the PANK2 gene (OMIM 606157) transmitted in an autosomal-recessive fashion. Hypoprebetalipoproteinemia, acanthocytosis, retinitis pigmentosa, and pallidal degeneration (HARP) syndrome is allelic with PKAN, although only a few cases are known. To date, 166 different mutations linked to PKAN have been reported, which reveals a high allelic heterogeneity. However, in certain populations, some mutations are relatively prevalent due to founder effects: c.1142_1144delGAG in the Netherlands, c.680A>G (p.Tyr227Cys) in the Dominican Republic; and the c.1583C>T (p.Thr528Met) in the Gypsy population of the Iberian Peninsula. PLAN, also an autosomal-recessive entity, is due to mutations in PLA2G6 (OMIM 603604). This form encompasses a continuum of three different and overlapping phenotypes: classic INAD, atypical neuroaxonal dystrophy (ANAD), and PLA2G6-related dystonia–parkinsonism. Recently, two siblings, compound heterozygotes for PLA2G6 mutations, showed a complex hereditary spastic paraplegia with optic atrophy, expanding the PLA2G6-associated phenotypic spectrum. So far, 153 distinct clinical variants are known, with no recurrent mutation. It is striking that three PLAN patients have been published in whom the disease is caused by a PLA2G6 change together with a partial uniparental disomy affecting chromosome 22.
These types are followed by MPAN that accounts for 6–10% and BPAN that occurs in and 1–2% of cases, although an increasing number affected with the latter have been identified in recent years. Mutations in the C19orf12 gene (OMIM 614297) lead to MPAN, an autosomal-recessive disorder, and despite its low frequency, 34 clinical variants are known. A founder event has been associated with the p.Gly69ArgfsX10 change in a Polish cohort. The C19orf12 gene has also been related to hereditary spastic paraplegia (HSP) type SPG43, although in a unique case. Regarding BPAN, patients carry mutations on the WDR45 gene, often de novo, inherited in an X-linked dominant manner. To date, about 50 BPAN cases have been published with 64 different mutations.
The remaining NBIA forms are rare, with only a few patients described for each condition. Mutations in the FA2H gene (OMIM 611026) cause three distinct autosomal-recessive conditions: FAHN, leukodystrophy, and HSP type SPG35. Thus, 37 different mutations have been described, and most of them lead to SPG35. A similar scenario occurs for the ATP13A2 gene (OMIM 610513), which is responsible for Kufor–Rakeb syndrome, Parkinson disease type 9, and neuronal ceroid lipofuscinosis.
Directly linked to iron metabolism, two NBIA genes, CP and FTL1, are known. Mutations in CP, inherited in an autosomal-recessive manner, cause aceruloplasminemia (OMIM 117700). To date, nearly 50 different mutations are described. Strikingly, patients carrying only one mutation can exhibit symptoms. Mutations, mainly small insertions, in the FTL1 gene cause neuroferritinopathy (OMIM 134790), an autosomal-dominant NBIA form. Although more than 60 FTL1 variants are known, the vast majority are associated with hyperferritinemia–cataract syndrome.
Other ultra-rare aceruloplasminemia NBIA forms are due to mutations in DCAF17, COASY, SCP2, and GTPBP2. About 12 pathological changes have been related to the autosomal-recessive multisystem syndrome known as Woodhouse–Sakati syndrome, which is caused by mutations in DCAF17, also named as C2orf37 (OMIM 612515). Three distinct mutations in the COASY gene, which encodes for CoA synthase, are reported to cause CoPAN in three unrelated cases (OMIM 609855). Regarding SCP2, two NBIA cases have been reported (MIM 184755), and only one case with mutations in GTPBP2 (OMIM 607434).
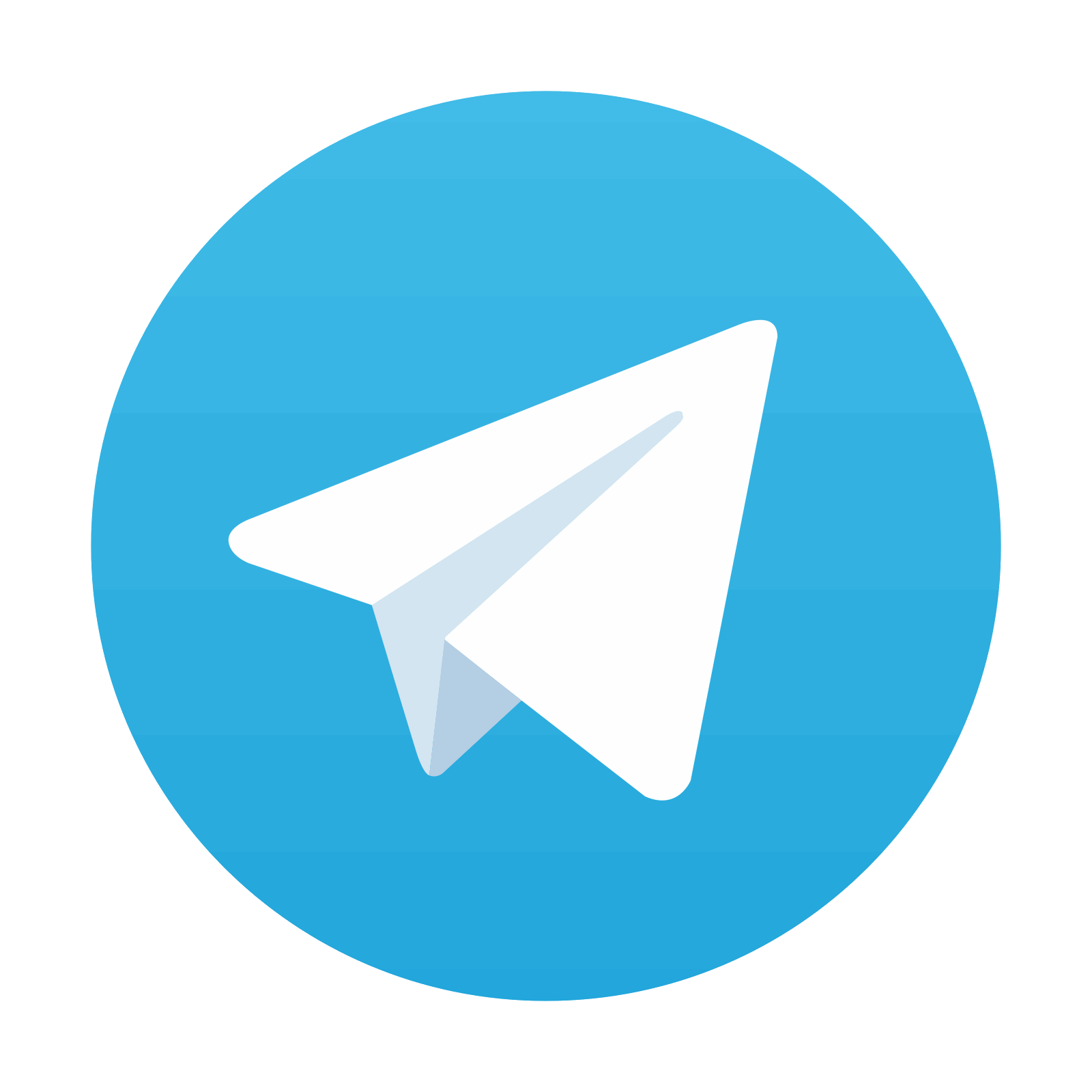
Stay updated, free articles. Join our Telegram channel

Full access? Get Clinical Tree
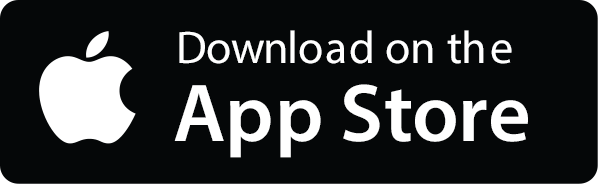
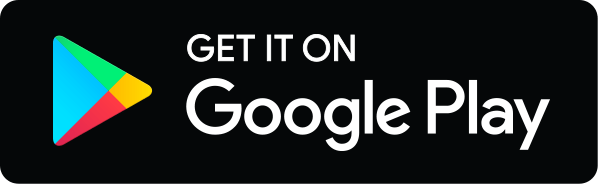
