Chapter 17 MRI-negative refractory focal epilepsy in childhood
MRI-Negative Epilepsy, ed. Elson L. So and Philippe Ryvlin. Published by Cambridge University Press. © Cambridge University Press 2015.
Introduction
The referral of children with refractory epilepsy for surgical intervention is now axiomatic. Recurrent seizures that begin early in life are associated with later cognitive decline and a reduced quality of life, and thus mandate earlier rather than later referral. Long-term seizure freedom rather than improvement is the primary surgical goal. In response, most pediatric epilepsy surgery centers now accept infants and very young children as potential candidates, and have protocols in place to localize seizure origin, maximize postoperative success and reduce potential surgical complications.
The importance of MR imaging in the preoperative evaluation for epilepsy surgery cannot be overestimated. In a recent review of current practice worldwide by the ILAE taskforce [1], MR imaging was performed in 99.5% of pediatric epilepsy surgical candidates. Utilizing high-resolution epilepsy protocols and expert interpretation, MR imaging can detect focal abnormalities in as many as 85% of patients with previously negative studies [2]. The demonstration of a focal lesion helps define the epileptogenic region (ER) and assists the surgical strategy either in preparation for single stage procedures, or in guiding placement of intracranial electrodes to gather further electrophysiological data.
In contrast, the inability to detect a focal lesion on MRI presents significant challenges to the successful localization of the epileptogenic zone. Histopathological analysis of tissue removed in MRI-negative cases often reveals microscopic abnormalities. The incidence of MRI-negative cases in the ILAE taskforce series was 17% [1], although meta-analysis of patients of all ages reveals a considerably higher incidence of MR negativity in the pediatric population, 31% in children versus 21% in adults [3]. Referral bias is undoubtedly important as some centers report an incidence of MRI-negative cases as high as 53% [4].
This chapter focuses on select aspects of epilepsy surgery in the MRI-negative cohort that are unique to childhood. We review specific challenges to the definition of the cohort, candidacy and special syndromes, evaluation and outcomes.
Definition of the cohort
As in adults, the MRI-negative cohort in children includes not only patients with normal MRI scans but also with nonspecific findings such as cortical atrophy or ventriculomegaly and incidental focal abnormalities [5]. A word of caution is in order with regard to the MRI investigation of focal malformations of cortical development (FCD) in early childhood. The MR appearance of FCD often changes during brain maturation. Although FCD may be detected in infancy, being hypointense on T1-weighted images and hyperintense on T2-weighted images, these findings may become more apparent or occasionally completely disappear on later scans [6]. This problem is particularly significant in Taylor-type FCD. Unfortunately, there is considerable variability across centers on specifics of MRI sequences and the ILAE is proposing age-specific recommendations for children under age 2 years [7].
Utilizing 3 T MRI or advanced computerized postprocessing to define statistical variations in cortical thickness, signal intensities or gray–white blurring may increase the diagnostic yield but are not an absolute requirement for clinical definition of the MRI-negative cohort. Furthermore, the argument can be made that identification of the lesion does not necessarily simplify the evaluation process or influence outcome in cases of FCD; thus children revealing subtle FCD lesions may be considered within the broader definition of an MRI-negative cohort.
Special MRI-negative refractory focal epilepsy populations in childhood
Focal cortical dysplasia
Focal cortical dysplasia (FCD) accounts for the majority of MRI-negative epilepsy surgical cases. Indeed the MRI evaluation is negative in approximately one-quarter of children with FCD [8]. It comprises a significant proportion of cryptogenic childhood cases and likely contributes to the over-representation of MRI-negative cases in childhood. In the largest reported MRI-negative pediatric epilepsy surgical series [5], FCD was observed in 77 of 102 (75%) surgical specimens; 71 of the 77 specimens contained lower-grade features (92%). In a smaller pediatric series, all 14 patients harbored FCD [9], and it is not unusual to observe different subtypes of FCD in different lobes of multilobar resections [9, 10].
Medically resistant cases of pediatric focal epilepsy due to FCD typically begin in the first few years of life [8]. Affected patients evidence significant rates of neurological disability, daily seizures and status epilepticus [8]. Pre- and perinatal lesions occur in 12.5% of patients with FCD, particularly low-grade dysplastic subtypes [11], whereas postnatal comorbid disorder such as head injury and infection are unusual [8]. This population is thus extremely high risk for morbidity and resource-intensive for their medical care.
Given the high incidence of FCD in the MRI-negative pediatric epilepsy surgical cohort, expertise with the underlying neurological issues is imperative. Electrophysiologic investigations reveal a high incidence of focal intermittent slowing and slow background activity on EEG [8]; a normal EEG is observed in only a small minority of children. Regional ictal patterns occur in over half of children with FCD [8]. Because of widespread dysplastic involvement in the very young, large resections including multilobar procedures and hemispherectomy are common, and a high proportion of children require invasive EEG recording.
West syndrome
Infantile spasms were viewed historically as a form of primary generalized epilepsy until surgical resection of discrete cortical lesions in symptomatic patients resulted in dramatic cessation of the spasms [13]. This “cortical hypothesis” for epileptogenesis in West syndrome was supported by PET studies revealing localized regions of hypometabolism in the epileptogenic zone [14] which reformulated the spasms as a rapid secondary generalized epilepsy. In medically refractory cases, focal or regional excisional procedures can achieve cessation of spasms, long-term seizure freedom and improved cognitive status [14, 15].
MRI-negative cryptogenic cases of West syndrome with hypsarrhythmic EEGs that are medication-resistant are an important sub group for surgical consideration. The EEG evaluation may yield important information supporting potential surgical candidacy. In a study of hypsarrhythmic EEG patterns, Gaily et al. [16] showed that hypsarrhythmic EEGs were asymmetric in 25% and asynchronous in 7% of cases. These lateralized features correlated with ictal EEG discharges contralateral to the clinically involved side, and demonstrated that coexistent partial motor seizures are particularly frequent. Localized EEG features including paroxysmal fast frequencies, rhythmic discharges and subclinical seizures are now recognized frequently in MRI-negative surgically treated cases of infantile spasms [17].
Use of PET scans plays an important role in the evaluation of medically refractory infantile spasms [18]. If concordance can be demonstrated between the zone of hypometabolism and focal EEG findings, seizure freedom is distinctly possible. Unfortunately, only about 20% of infants demonstrate a focal hypometabolic zone, the remainder showing either multiple hypometabolic foci (65%) or diffuse and symmetric hypometabolic changes (5%) [18]. Bi-temporal metabolism occurs in 10% of cases and is associated with a high rate of autism [19].
Preoperative investigations
MRI-negative children should undergo an intense evaluation for exclusionary criteria: genetic/idiopathic (e.g., SCN1A, atypical BECTS), neurodegenerative or metabolic syndromes that may present as refractory partial seizures (Table 17.1). They also require serial assessments to document temporal consistency of clinical semiology and diagnostic localization. The time frame over which consistency is conferred depends on the degree of acuity of presentation, including catastrophic seizures and neurocognitive or behavioral deterioration.
Consistent focal findings on the scalp EEG (Figure 17.1) and clearly delineated ictal semiology are the cornerstones of the evaluation of children with MRI-negative focal epilepsy. Both are influenced by maturational factors, and the potential for significant changes over time must always be considered. This is especially evident in infants whose symptomatic focal epilepsy is more likely to propagate to motor pathways leading to apparent electroclinically generalized epileptic seizures [20].
Figure 17.1 Scalp EEG showing semi-periodic discharges consistently over the right centrotemporal region.
All children with refractory MRI-negative focal epilepsy should initially undergo video-EEG recording with ictal capture. The demonstration of a focal region of epileptogenesis should prompt reinvestigation of the MRI for evidence of subtle abnormalities. Ideally, the epileptogenic region should be convergent with the semiologically suspect anatomic region of seizure onset. Particular attention must be given to the identification of electrographic features at seizure onset that suggest lateralized or regional seizure origin.
The role of functional imaging in pediatric cases is well established. Ictal SPECT and PET are both sensitive and specific tools to delineate temporal and extratemporal seizure onset zones [21–24]. Functional imaging is generally required in all MRI-negative patients and is obligatory if there is clinical and electrophysiological divergence. Although ictal SPECT is considered superior for localizing extratemporal foci and PET superior for temporal foci, a comparison of both modalities in pediatric temporal lobe epilepsy reveals diagnostic accuracy in the range of 80–90% for both [25].
More recently, magnetoencephalography (MEG) has been employed successfully in MRI-negative cases. In a cohort of 22 children with subtle or nonfocal MRI findings, Ramachandran Nair and colleagues [26] identified 17 (77%) with good surgical outcome and eight (36%) who became seizure-free. Postoperative seizure freedom correlated with the presence of MEG clusters within the resection margin whereas bilateral MEG dipole clusters or scattered dipoles correlated with seizure persistence. Seizure freedom was greater when there was complete concordance between MEG and EEG localization. Multiple seizure types predicted poor postoperative outcome.
The use of multiple modalities to define the epileptogenic zone in MRI-negative pediatric refractory focal seizures is widely regarded as state-of-the-art [5, 9, 27, 28]. Which modalities are utilized depends to a large extent on local institutional protocols and resources, and there is only limited data to support the superiority of a single approach. In a study of 14 children undergoing comprehensive multimodal investigations for refractory MRI-negative focal epilepsy, SISCOM and MEG showed better concordance with intracranial EEG data than PET [9]. However, PET and SPECT data do not guarantee a seizure-free outcome [28]. As the number of patients in these studies was limited, it is difficult to draw any definitive conclusions.
In the largest cohort of MRI-negative children with refractory focal epilepsy reported to date, 102 patients underwent multimodal investigation that integrated PET and SPECT with video-EEG, extra operative subdural recording and electrocorticography [5]. This comprehensive pre- and perioperative approach facilitated excisional procedures in 102 children, including 66 unilobar and 36 multilobar resections. Of note, preoperative evaluations were individualized according to individual profiles rather than being rigidly tracked through a preset protocol. This multimodal flexible approach did not aim to employ all possible diagnostic modalities yet achieved successful surgical planning and a reasonably good rate of postoperative seizure-freedom (44% at 2 years).
Functional considerations
Patients with MRI-negative refractory focal epilepsy are more likely to exhibit atypical language representation. In a study of 102 patients with left hemisphere epileptogenic zones evaluated with fMRI language tasks [29], atypical language was more prevalent if the MRI was normal (36%) compared to 21% with hippocampal sclerosis (HS) and 14% with other focal cortical lesions (dysplasia, tumor, vascular malformation). Of particular relevance for pediatric epilepsy surgery, atypical language was over-represented in patients with early seizure onset and atypical handedness. Atypical language representation was associated with lower verbal abilities and a trend towards lower nonverbal abilities.
Receptive language competence appears particularly vulnerable to reorganization. In a study of the relationship of age of onset of temporal lobe epilepsy to subsequent intellectual ability, Cormack et al. [30] found that seizure onset in the first year of life was associated with a particularly high (82.4%) incidence of later intellectual impairment. Epilepsy originating from either temporal lobe in early life was sufficient to produce intellectual dysfunction. In contrast, seizure frequency and the duration of the epilepsy were not significant variables.
The selective vulnerability of the temporal lobe to early-life seizures and lack of any relationship to underlying pathological substrate was further confirmed in two recent investigations [31, 32]. Both studies, one in an adult cohort, and one in a pediatric cohort, revealed an increased incidence of atypical language representation on functional MRI and a higher incidence of left-handedness. The children in the Kresk et al. [32] study additionally evidenced linguistic deficits and relatively greater vulnerability for receptive versus expressive language networks.
The collective evidence from several lines of investigation therefore suggests that MRI-negative patients, particularly if the temporal lobe is the epileptogenic source, must undergo a careful evaluation of language representation. Assumptions of cortical sites for language cannot be taken for granted, and the sites are most likely to be atypical in MRI-negative temporal lobe patients. By contrast, data on motor representation in MRI-negative children are limited, although anecdotal cases have demonstrated atypical functional organization that may have relevance to surgical planning.
Invasive investigations
Invasive EEG recording is considered the gold standard in MRI-negative cases, but data on its utility in children are limited [33]. Utilization occurs mainly where there is inconclusive or divergent noninvasive data. Also, utility may be greater in extratemporal or multilobar foci as compared to temporal foci that are often amenable to standard lobectomies. Rates of explantation without resection vary considerably across centers, and given the additional risk and costs, added caution is recommended in patient selection [34] or utilizing invasive EEG as an exploratory procedure without an a priori hypothesis of the epileptogenic region to guide electrode placement.
Invasive EEG can be recorded via subdural, depth or a combination of electrodes. The electrodes may be placed through an open craniotomy or stereotactic depth placement; the latter is generally feasible only above age 3 years. As in adults, the ictal onset zone is considered the most localizing marker but other markers such as significant background abnormalities, repetitive or rhythmic interictal discharges, or intraictal activations deserve added consideration in the absence of a structural lesion. More recently, interictal high-frequency oscillations have attracted attention but their role in localizing seizure onset in children is uncertain.
Electrocorticography (ECoG) is influenced by anesthesia and generally provides only interictal data, which limits its usefulness [35, 36]. In a subset of patients with FCD however, the ECoG may reveal continuous focal ictal–interictal discharges. These discharges often correlate with the ictal onset zone (Figure 17.2). They thus serve as a reliable marker of the epileptogenic region and may alleviate the need for ictal capture through extraoperative invasive EEG recording.
Figure 17.2 Subdural EEG recording showing interictal semi-periodic discharges. Ictal onset (arrow) is characterized by alteration in the rate of the discharges followed by their disappearance and focal attenuation.
Decision algorithm
Each center generally adopts its own specific protocol to define the epileptogenic region in this cohort. Nonetheless, given the escalating number of diagnostic tests and the costs and risks incurred without necessarily documented benefit, the ILAE is proposing recommendations to achieve a certain degree of standardization [7]. As mentioned earlier, MRI-negative children should have a greater emphasis on exclusionary criteria: genetic/idiopathic (e.g., SCN1A, atypical BECTS), neurodegenerative and metabolic syndromes as compared to lesional cases, and they should undergo serial assessment to document temporal consistency of localization.
Centers dealing with MRI-negative cases are expected to have capabilities for ancillary investigations that include most of the following: 3D EEG source, MEG, FDG-PET or SPECT to allow a hypothesis for location of the epileptogenic region that can then be confirmed by either ECoG or extraoperative invasive recordings; FDG-PET and SPECT are the most widely used ancillary tests. Between the two functional imaging modalities, FDG-PET is easier to perform and has been proposed as the initial test in MRI-negative cases, particularly if FCD is the predominant substrate; SPECT is better suited than FDG-PET in patients with prior resections, but is technically more challenging and requires seizures to be of sufficient frequency and duration to permit adequate ictal capture. Both PET and ictal SPECT are susceptible to the effects of seizure propagation and are thus useful mainly for defining overall ER location but not necessarily its extent. Subtraction of ictal and interictal SPECT potentially overcomes this limitation but imposes the need for an additional SPECT test and computational expertise. Additional electrophysiologic corroboration is therefore strongly recommended.
The MEG technique is advocated at some centers while 3D EEG source imaging is generally underutilized. Given the complementary nature of 3D EEG and MEG, usage of both tests optimally as simultaneous recordings, is encouraged. However, in consideration of the significant cost-differential between the two tests, it is suggested that 3D EEG be used first, and MEG used when the former is inconclusive or divergent. The MEG method may be particularly justified in MRI-negative cases where the dipole source is suspected to be tangential such as rolandic, sylvian or interhemispheric foci, or in postoperative failures where the EEG fields are likely to be distorted.
Outcome of surgical intervention
There is a paucity of information about postoperative seizure outcome in MRI-negative pediatric epilepsy patients. In an early report of 75 children with refractory focal epilepsy undergoing excisional procedures, 59% were seizure-free at 2-year follow-up. Seizure outcome for the 40 MRI-positive cases was not statistically different from the 35 MRI-negative cases [4]. When Engel class I and II outcomes were combined, the MRI-positive group maintained a slight overall advantage (80% versus 74%). This cohort was a pure pediatric population as the mean age at seizure onset was 2.8 years and mean age at surgery was 7.7 years. A much higher proportion of MRI-negative cases were extratemporal, and 73% of patients underwent extraoperative intracranial EEG evaluation. Completeness of resection of the epileptogenic zone was the only significant predictor of surgical success, thus confirming that neither the pathological substrate nor the presence of a focal MRI lesion are critical variables for postoperative seizure freedom.
In a cohort of purely MRI-negative children, the rate of seizure freedom at 2 years was 44% [5]. Although the rate of seizure freedom was lower than the Paolicchi and colleagues’ [4] study, the 44% 2-year seizure-free rate remained stable at 5 years and decreased only slightly to 38% at 10 years. Thus, long-term seizure freedom is possible in MRI-negative children with pharmacoresistant focal epilepsy. Completeness of resection was again found to be the most important variable affecting surgical success; the presence of convergent interictal spike discharges on scalp EEG also correlated with seizure freedom.
Comparable rates of seizure freedom were reported in three other pediatric cohorts [9, 26, 28]. The incidence of postoperative seizure freedom ranged between 36–50% at time of follow-up, and the best predictor of seizure freedom was completeness of resection. Neuropsychological testing obtained pre- and postoperatively revealed no significant deterioration in overall cognitive status [28]. Scalp-recorded MEG clusters correlated with a favorable outcome, a finding consistent with the predictive power of interictal epileptiform discharges [5]. Of some significance, the performance of multiple subpial transections (MST) either as the sole procedure or in conjunction with excisional procedures was significantly associated with poorer seizure outcome. This finding likely reflects the known suboptimal outcome if eloquent cortical regions are found in proximity to the epileptogenic zone [4].
While the numbers of children with normal or nonspecific MRI scans who undergo surgical therapy is small, the existing evidence indicates that by using a multimodal approach, favorable outcomes are indeed possible and that rates of seizure freedom are comparable to adults. While focal cortical dysplasia is the underlying pathology in the majority of candidates, outcome is pathology-independent and only depends on the completeness of resecting the epileptogenic region. Incomplete resections generally lead to less favorable outcomes, yet, some children may achieve seizure freedom or substantial reduction of seizure burden and should not be excluded from surgical consideration.
The number of children with refractory focal epilepsy representing the MRI-negative cohort is unknown but it is important to increase awareness of the promising outcomes of resective surgery to promote early referrals and optimize the benefit of surgical interventions.
References




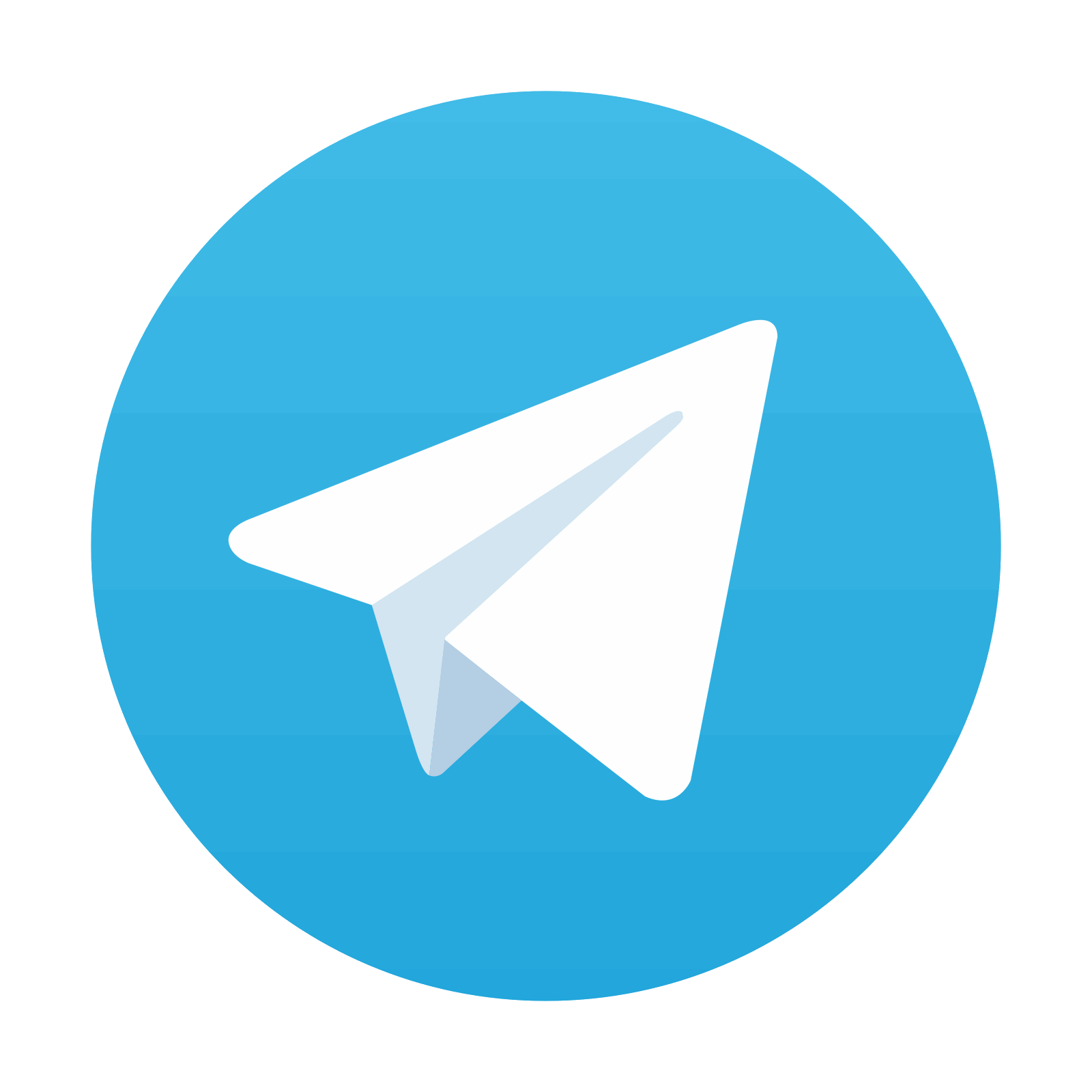
Stay updated, free articles. Join our Telegram channel

Full access? Get Clinical Tree
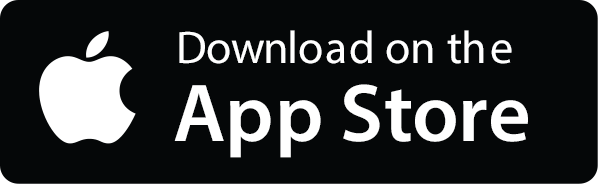
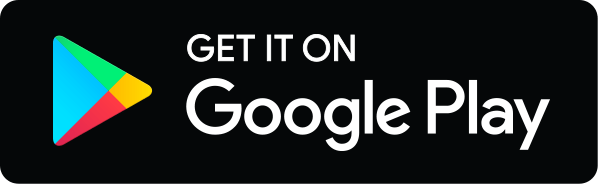
