Abstract
The study of abnormal movements has been an important topic in neurology since over a century ago. In fact, movement disorders are a rapidly growing subspecialty that receives contributions from different disciplines including adult neurology, child neurology, neurosurgery, psychiatry, and physical medicine and rehabilitation. The main focus is on normal and abnormal functioning of the motor systems and on possibilities to treat motor dysfunction. The traditional approach to study and treat movement disorders has been largely based on the analysis of the clinical phenomenology that results from the interaction of a number of brain regions that provide motor control under different functional states. Therefore the development of anatomical and physiological studies of the neural circuitry of motor systems, together with the precise description of corresponding clinical signs, has encouraged the study of this broad topic during the last decades. Brain imaging, neurophysiology, and basic research studies in animal models have provided an increasing amount of knowledge.
Introduction
The study of abnormal movements has been an important topic in neurology since over a century ago. In fact, movement disorders are a rapidly growing subspecialty that receives contributions from different disciplines including adult neurology, child neurology, neurosurgery, psychiatry, and physical medicine and rehabilitation. The main focus is on normal and abnormal functioning of the motor systems and on possibilities to treat motor dysfunction. The traditional approach to study and treat movement disorders has been largely based on the analysis of the clinical phenomenology that results from the interaction of a number of brain regions that provide motor control under different functional states. Therefore the development of anatomical and physiological studies of the neural circuitry of motor systems, together with the precise description of corresponding clinical signs, has encouraged the study of this broad topic during the last decades. Brain imaging, neurophysiology, and basic research studies in animal models have provided an increasing amount of knowledge. However, even though inborn errors of metabolism (IEMs) have more than 100 years of history [1], they have only become a relevant part of the movement disorders field in the last couple of decades. This is due to the fact that IEMs historically were considered a pediatric subspecialty and therefore have been the province predominantly of pediatricians. As neurological manifestations have been appreciated to be a major contributing factor, an increasing body of literature has highlighted the vulnerability of the nervous system in IEMs. Some of the first systematic approaches to well-defined neurological syndromes were reported in 2007 and 2009 [2–5]. From these first descriptions until now, both neurology and the field of IEMs have experienced a significant transformation driven by the advent of next-generation sequencing techniques.
The recent updated nosology of IEMs [6] includes more than 1,100 diseases and almost 80% of them exhibit neurological symptoms. In particular, during the last 5 years, more than 300 new IEMs have been described as emerging causes of neurological dysfunction. These new disorders have changed paradigms, transforming the concept and classification of IEMs and are contributing enormously to our understanding of mechanisms in neurological diseases. Therefore, there is a great need for both fields – neurology (and in particular the field of movement disorders) and metabolism – to converge and share perspectives. Crucial aspects such as clinical phenomenology, pathophysiological categories, and therapeutic approaches need to be redefined.
General Concepts
Prevalence and Types of Movement Disorders in IEMs
There are no detailed epidemiological studies that provide the exact prevalence of movement disorders in IEMs. However, if we consider that 80% of IEMs exhibit neurological symptoms and that movement disorders include a broad spectrum of manifestations appearing at any age, one would estimate that they are present in some 70% of IEMs. Movement disorders in IEMs include ataxia, athetosis, chorea, dystonia, myoclonus, stereotypies, tics, tremor, and parkinsonism. They may be accompanied by hypotonia, weakness, spasticity, apraxia, and other motor deficits [7]. Movement disorders have been traditionally divided into “hyperkinetic” disorders, in which there is excessive movement, and “hypokinetic” disorders, in which there is a paucity of voluntary movements. Hyperkinetic disorders include chorea, dystonia, athetosis, myoclonus, stereotypies, tics, and tremor. They consist of abnormal and often repetitive involuntary movements. Hypokinetic movement disorders mainly present as akinetic–rigid syndromes.
All kinds of movement disorders are present in IEMs [8]. As a general rule, hyperkinetic and complex movement disorders (a combination of different types of movement disorders) are common in children. In fact, parkinsonism is very rare in the pediatric age group. In a case series of pediatric movement disorders including 673 individuals seen at a single center, Fernández-Álvarez and Aicardi found a prevalence of parkinsonism of 2%, and the average age at onset was 11.3 years [9]. In contrast, in adolescents and adults, hypokinetic movement disorders are more frequent, particularly in neurodegenerative diseases.
Special Characteristics of Movement Disorders in IEMs
The diagnosis of IEMs that primarily affect the nervous system is a major challenge. The same neurological symptoms and pattern of disease progression may be caused by non-metabolic disorders. Over the last years, this has become even more challenging because of the rapidly increasing number of new disease entities and novel pathophysiological categories. Early detection remains key, as outcomes tend to be better, particularly in treatable IEMs. Some characteristics of movement disorders that may lead to the suspicion of an IEM are highlighted (Box 2.1). The diagnostic challenge is even greater in early childhood, where many features of neurodevelopmental disorders can mimic a movement disorder. Similarly, patients with advanced neurodegenerative disease as a result of different IEMs may present with similar movement disorders.
Box 2.1 Special characteristics of movement disorders in IEMs
Diffuse clinical picture with other neurological or systemic symptoms
Atypical presentations, such as the coexistence of different neurological features, that do not localize to a distinct neuroanatomical region
Acute, subacute, or recurrent presentations including severe presentation such as with status dystonicus
Progressive movement disorders
Focal movement disorders (such as unilateral dystonia) do not exclude an IEM, however as a rule, focal signs tend to generalize or be associated with other symptoms over time.
Fluctuation of symptoms: For example, in neurotransmitter defects with dopaminergic dysfunction, dystonia and oculogyric crises may worsen over the day and improve with rest. In glucose transporter type 1 (GLUT1) deficiency syndrome, symptoms like ataxia, dysarthria, and dyskinesia may worsen with fasting and improve after eating or rest; in urea cycle disorders, altered mental status, hyperkinesia, and other symptoms may develop with intercurrent illnesses or fluctuate depending on protein intake.
Symmetrical lesions on brain MRI
Lack of response to symptomatic treatment
Movement disorders that are not explained by classic etiologies (acquired brain injury, hypoxia, stroke, trauma, infection, inflammation, demyelinating disease)
Positive family history, particularly if suggestive on an autosomal-recessive inheritance
Biochemical and Neurobiological Aspects of Movement Disorders in IEMs
Neurobiology of Movement Disorders
The complex neurobiology of movement disorders goes beyond the scope of this chapter. Nevertheless, it is interesting to summarize the main characteristics of brain circuitries involved in motor dysfunction, in order to better understand the reason why movement disorders are frequent in IEMs. Correlations of involuntary movements with pathological changes in the basal ganglia were established in the first half of the twentieth century. The classic teaching is that the basal ganglia and the cerebellum are the two major motor centers controlling voluntary movements [10]. Dystonia and parkinsonism have been explained based on the classic model of basal ganglia circuitry. Cortical input to the striatum is relayed to two main output nuclei: the globus pallidus internus (GPi) and substantia nigra pars reticulata (SNpr), through indirect and direct pathways. The activity in the direct pathway facilitates movement by reducing the inhibitory output from the GPi. The activation of the indirect pathway increases inhibitory outputs and reduces movement. Dystonia is thought to result from an imbalance in the direct and indirect pathways, which leads to reducing the inhibition of the thalamus and increasing the excitability of the motor cortex. Conversely, parkinsonism results from the overactivity of GPi and SNpr output, which causes an increased inhibition of the thalamus and reduced motor cortex excitability [11].
Other hyperkinetic involuntary movement disorders such as chorea or ballismus are thought to result from a disinhibition of motor output caused by the disturbance of inhibitory mechanisms in the basal ganglia [10]. This dynamic model of basal ganglia function (Figure 2.1) is mediated by dopamine, acetylcholine, glutamate, and gamma-aminobutyric acid (GABA). Pathways that connect motor structures are myelinated axons organized in complex circuits. Glutamate and GABA are key molecules in both energy and intermediate metabolism. Excess glutamate can lead to excitotoxicity, a process that is common not only in intoxication-type IEMs but in many neurodegenerative diseases. Disorders of complex molecules such as peroxisomal and lysosomal disorders strongly affect white matter composition and integrity [12]. Moreover, axonal transport is a high-energy-consuming mechanism. Therefore, the proper function of the basal ganglia, together with other motor structures and the circuits between them, depends on a perfectly regulated metabolism, which explains the high prevalence of movement disorders in IEMs.
Figure 2.1 Dynamic model of basal ganglia function. The hyperdirect pathway, the cortical-subthalamo-pallidal circuit, regulates the execution of voluntary movement. This pathway inhibits all voluntary motor tasks. Glutamate and GABA are involved in the modulation of this circuit. Abbreviations: G. PALL. globus pallidus; INT: interna; EXT: externa; RET: reticularis; ST: substantia.
Biochemical Properties of Motor Structures and Circuitries
Although elementary concepts have been introduced in the paragraph above, we are far from having a detailed knowledge about the biochemical properties of the basal ganglia and other brain structures involved in motor function. Additionally, brain metabolism evolves over time, being markedly different across the diverse pre- and postnatal developmental stages, as well as throughout adulthood and with aging.
Recent neurochemical studies of the striatum reveal a heterogeneous pattern with separation into discrete compartments known as striosome and matrix compartments. These differ in their expression of neurochemical markers, and have also been suggested to have different involvements in a range of neurological diseases [13]. The striosome compartments form a three-dimensional labyrinth-like structure that interdigitates the matrix and expresses high levels of μ-opioid receptor, substance P, dopamine 1-receptor, met-enkephalin, calretinin, nuclear receptor subfamily 4 group A member 1 (Nr4a1), pro-dynorphin, generalized anxiety disorder 2-item (GAD-2), and growth response protein 1 (ERG-1). The extrastriosomal matrix, by contrast, is enriched with calbindin, somatostatin, enkephalin, DA2-receptor, and cholinergic markers including acetylcholine esterase and choline acetyltransferase [13–16]. Striosome and matrix compartments are thought to have distinct anatomical connections and developmental programs and may be separately involved in pathological processes [17].
A recent study investigating the longitudinal increase of brain metabolite levels in 5–10-year-old children [18], reported increasing levels of glutamate (Glu) and glutamine, N-acetylaspartate (NAA), and NAA+NAAG (N-acetyl-aspartyl-glutamate). The increase of NAA+NAAG levels with age was about twice that of NAA, implying similar increases in NAAG levels. The age-related rise of both NAA and Glu levels are likely linked to the production of NAAG (NAA is combined with Glu to form NAAG). A possible explanation for increased NAAG levels in the basal ganglia is its role in regulating the release of glutamate and dopamine [19], indicating an age-related increased demand to modulate neurotransmitters during this developmental stage. The age-related rise in Glu could also be associated with an increase in intracellular Ca2+ that triggers biochemical events involved in both functional and structural plasticity of the synapses in childhood [20].
This complex metabolic regulation across different life stages may interact with the abnormal network of metabolites and cellular mechanisms that are characteristic of IEMs.
Pathophysiological Categories of IEMs That Cause Prominent Movement Disorders
The recently updated nosology of IEMs lists more than 1,100 IEMs, provisionally classified into 110 groups [6]. These groups are very diverse and may imply either one compound, one pathway, one cofactor common to many pathways, one system common to several compounds, and multiple pathophysiological mechanisms involving energy production, mitochondrial machinery, signaling and trafficking, various organelles, nucleic acid metabolism, or metabolite repair. An extensive list of conditions may be overwhelming to clinicians and has little in common with the clinical approach to IEMs. A simplified and updated classification of IEMs proposed by Professor Jean-Marie Saudubray mixes elements from the practical diagnostic approach with pathophysiological considerations into three large categories, based on the size of molecules (“small and simple” or “large and complex”) and their implication in energy metabolism [21] (Box 2.2)
Movement disorders in intoxication-type disorders may present acutely at any age. They may be also one of the prominent neurological symptoms in the long-term evolution of the disease; i.e. generalized dyskinesias and chorea in glutaric aciduria type 1 (GA-1), tremor in phenylketonuria, diverse movement disorders in galactosemia.
Movement disorders in small-molecule deficiencies are associated with early and severe global encephalopathies in the majority of cases. Spasticity is the most common sign in amino acid and fatty acid defects whereas dystonia and dyskinetic movements are common in metal storage diseases
Movement disorders are frequent in defects of energy metabolism since the basal ganglia and cerebellum are particularly vulnerable. Movement disorders can appear abruptly in acute crises in “classic” energy defects such as Leigh syndrome. GLUT1 deficiency syndrome is an important disorder with a wide range and expanding phenotype of movement disorders including ataxia, dyspraxia, spasticity, abnormal ocular movements, chorea, and paroxysmal dyskinetic attacks.
Movement disorders are common in complex molecule defects and tend to be chronic and progressive.
Disorders of Small and Simple Molecules
Almost all IEMs in this category have metabolic markers and their diagnosis relies on plasma, urine, and cerebrospinal fluid (CSF) investigations (amino acid, organic acid, and metals analyses, etc.) with a metabolic signature. There are two subcategories in small-molecule disorders, depending on whether the phenotype primarily results from an accumulation or a deficiency:
1. Diseases linked to an accumulation: “Intoxication” disorders. These IEMs result from the abnormal accumulation of compounds proximal to the block and potentially reverse as soon as the accumulation is removed. Disorders in this category share some characteristics: (i) They do not interfere with fetal development and present after a symptom-free interval (days to years) with clinical signs of intoxication (acute, intermittent, chronic, and even progressive) provoked by fasting, catabolism, fever, intercurrent illness, or food intake. (ii) Most of these disorders are treatable and require prompt removal of the “toxin or stressor.” Treatments include special diets, scavengers, and cofactors. (iii) This group encompasses IEMs of amino acid metabolism (phenylketonuria or maple syrup urine disease), urea cycle defects, organic acidurias (methylmalonic aciduria, propionic aciduria, or GA-1), disorders of carbohydrate metabolism, metal storage diseases (i.e Wilson disease), and the porphyrias. Some purines/pyrimidines and metabolite repair defects (D/L-2-OH-glutaric aciduria and others) could be also included in this group.
2. Diseases linked to a deficiency: Symptoms result primarily from the defective synthesis of compounds distal to a block or from the defective transport of an essential molecule. These are, at least in theory, treatable by providing a missing compound. Most of these defects interfere with fetal development, have a prenatal or neonatal presentation, present with congenital defects, and share many characteristics with disorders in the complex molecule group. This group encompasses amino acid and fatty acid synthesis and transport defects and cofactor deficiencies (e.g. zinc, manganese, and copper) [22, 23].
Energy-Metabolism-Related Defects
These consist of IEMs with symptoms due, at least in part, to a deficiency in energy production or utilization within one or more tissues.
1. Membrane carriers (glucose, fatty acids, ketone bodies, monocarboxylic acids). GLUT1 (the glucose cerebral transporter) deficiency is an example.
2. Mitochondrial defects encompass aerobic glucose oxidation defects, presenting with congenital lactic acidemia (pyruvate transporter, pyruvate carboxylase, pyruvate dehydrogenase, and Krebs cycle defects), mitochondrial respiratory-chain disorders, mitochondrial transporters of energetic molecules, amino acids, ions, metals and vitamins, coenzyme Q biosynthesis, fatty acid oxidation, and ketone body defects. A large and growing group of already more than 110 disorders involves the mitochondrial machinery: Mitochondrial fusion, fission, replication, mitochondrial protein import and mitochondrial protein quality control, ribosomopathies, mitochondrial DNA depletion and intergenomic modification, mitochondrial transfer RNA (tRNA) synthetases and tRNA modification, phospholipid membrane metabolism, and other biological processes.
3. Cytoplasmic energy defects include disorders of glycolysis, glycogen metabolism, gluconeogenesis, hyperinsulinism, and GLUT1 deficiency syndrome (all treatable), creatine metabolism disorders (partially treatable), and, finally, inborn errors of the pentose phosphate pathways (untreatable).
Complex Molecules
This expanding group encompasses diseases that disturb the metabolism of complex molecules and involves metabolic processes in all organelles (mitochondria, lysosomes, peroxisomes, endoplasmic reticulum, Golgi apparatus, lipid droplets, and the synaptic vesicle). Cellular membranes are formed from a chemically diverse set of lipids. Lipids have several major functions in cells, including membrane structural components, energy and heat sources, signaling molecules, protein recruitment platforms, and substrates for post-translational protein–lipid modification [24]. In this complex molecule category there are two main subgroups:
1. Defects of catabolism, leading to storage of macromolecules (classic lysosomal defects, e.g. sphingolipidoses or mucopolysaccharidoses).
2. Synthesis, remodeling, processing, trafficking, and quality–control defects:
This rapidly expanding group encompasses classic organelle disorders (lysosome and peroxisome defects), congenital disorders of glycosylation (CDG) syndromes, and glycosaminoglycan synthesis defects [25], inborn errors of cholesterol and bile acid synthesis, the vast new group of complex-lipid synthesis and remodeling defects (triglycerides, phospholipids, glycosphingolipids), fatty acid synthesis and transport (elongation of essential and non-essential fatty acids, branched-chain fatty acids, fatty alcohols, and eicosanoids like arachidonic acid derived compounds and leukotrienes) [12, 26]. These all share some characteristics. They may interfere with embryonal and fetal development and have a pre- or neonatal presentation. Symptoms are permanent, very often progressive, independent of intercurrent events, and unrelated to food intake. Furthermore, many other defects affecting intracellular vesiculation, trafficking, processing of complex molecules, and quality-control processes (like protein folding and autophagy) have been recently discovered using the next-generation sequencing (NGS) technique. Some examples include CEDNIK due to mutation of the SNAP29 gene implicated in intracellular vesiculation [27], hereditary spastic paraplegia due to AP5Z1 mutations [28], Rabenosyn-5 mutations resulting in defective endocytic trafficking [29], synaptic vesicle cycle disorders [30], and congenital disorders of autophagy [31].
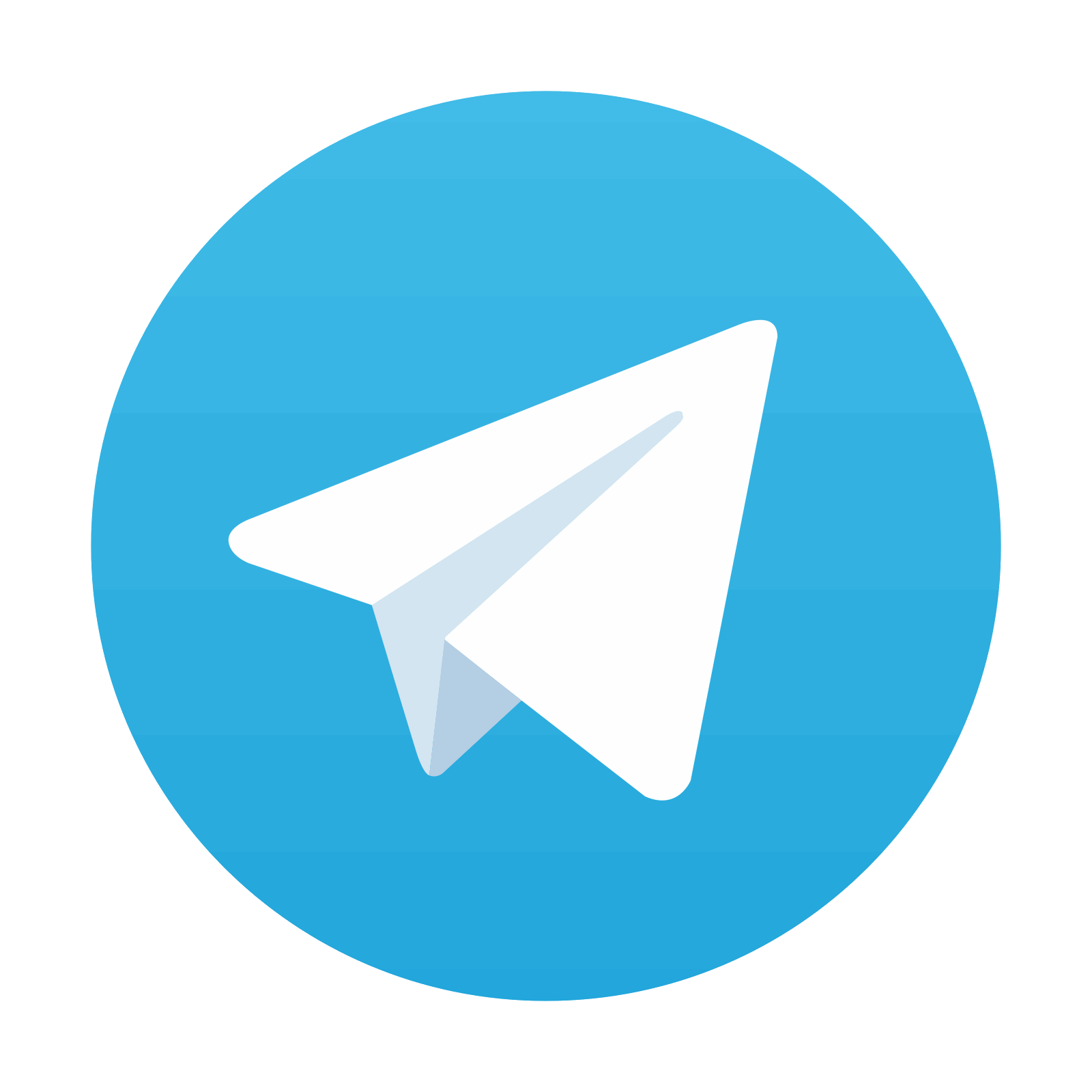
Stay updated, free articles. Join our Telegram channel

Full access? Get Clinical Tree
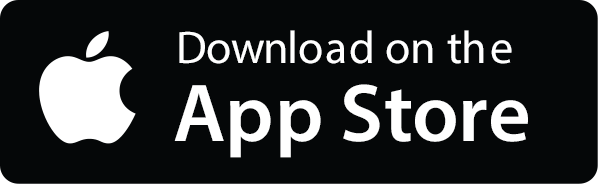
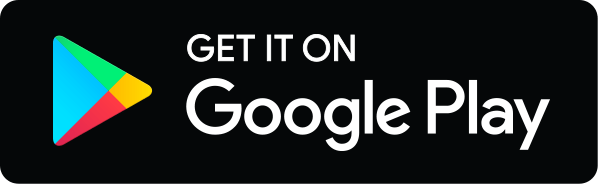
