Introduction
It is well known that stroke is a global healthcare problem that is very common and very disabling [1]. According to the World Health Organization (WHO) rehabilitation is a set of interventions designed to optimize functioning and reduce disability in individuals with health conditions in interaction with their environment [2]. Although impressive developments have been made in the medical and acute management of stroke, it still needs specific, effective, and individual rehabilitation interventions [3].
A 67-year-old man wakes up in the morning at 7 am and can’t move his right arm and his right leg at all. He can no longer speak coherently. In an attempt to call his wife he is unable to do that. Minutes later his wife calls the emergency services. At 7.30 am an ambulance team consisting of a paramedic and emergency medical technician is notified of an emergency. Five minutes later the ambulance arrives at the patient’s address and the emergency team find the slightly overweight patient sitting. The very agitated wife explains that her husband woke up beside her, but that he was much less responsive than usual. Upon making this discovery, she had attempted to lift him out of bed. During this maneuver she noticed that his speech was slurred and his answers to her questions were “jibberish” as she phrases it. The man is unable to stay sitting up on the edge of the bed, and repeatedly falls over onto the bed. The patient has also lost his motor control of the left arm and leg and also his bladder control, and he is unable to answer the emergency team’s questions adequately. The paramedic makes a diagnosis of a stroke with aphasia and impaired language comprehension. A couple of minutes later the team prepares to transfer the patient to the ambulance.
During the trip to the nearest hospital stroke unit, the paramedic phones the team of neurologists and the emergency department and gives them further details concerning the patient’s status. On arrival at the emergency room, the paramedic hands over the patient to the on-call neurologist. The MRI reveals an extensive infarction in the area of the middle cerebral artery.
Rehabilitation
Rehabilitation can be divided into three sections, starting with the acute care in hospital at stroke units and early rehabilitation in the first hours. Also important is the second part: the transition from hospital to specialized inpatient rehabilitation or to home (e.g. early supported discharge) and the community rehabilitation and reintegration. One central aspect of rehabilitation is the provision of a coordinated program from an interdisciplinary team of health professionals, doctors, nurses, physiotherapists, occupational therapists, speech pathologists, and dieticians. The rehabilitation team may be expanded to include other disciplines, e.g. psychologists and neuropsychologists.
According to Langhorne and colleagues, the individual rehabilitation after stroke typically involves [4]:
the assessment and evaluation, to identify and quantify the patient’s needs
the individual goal setting, to define realistic and attainable goals for improvement
individual and effective interventions, to assist patients in the rehabilitation process and in the achievement of their goals
the reassessment, to prove if individual goals are reached.
Acute Phase and Early Mobilization
A very important first question is when rehabilitation after stroke has to start. From a logical point of view and taking the definition of the WHO, rehabilitation after stroke has to start as early as possible. Okay, but when exactly, for example, should we start with getting people out of bed to stand and to exercise? In the last years research showed us that, for instance, early mobilization as an integral part of rehabilitation can be started within the first 24 hours after stroke as shown by a recent Cochrane review [5]. The latest research, however, from the AVERT trial, including more than 2 500 patients, showed that early mobilization can be done as in the first 24 hours, but shouldn’t start before 18 hours after acute stroke [6]. Certain subgroups of patients should wait a day or two longer before early mobilization, e.g. older persons with severe stroke or those with a hemorrhage stroke [6].
A recent analysis showed that shorter, more frequent mobilization early after acute stroke is associated with greater odds of favorable outcome at 3 months when controlling for age and stroke severity [7]. This means that patients should be mobilized out of bed early and frequently, but shorter periods of mobilization out of bed are better than one period with longer duration.
Our patient should therefore be mobilized because more than 18 hours have now passed since his stroke. This mobilization is done by the stroke unit team out of the bed. With the help of a physiotherapist giving support, the patient changes from the supine into a lateral position and from there he gets help until he sits on the bed. After a short period of checking the vital parameters while sitting on the bed supported by the therapist, the patient will be encouraged to stand up. The physiotherapist encourages the patient and supports him, e.g. for balance, while he stands up from the bed.
Rehabilitation can be divided into three sections, starting with the acute care in hospital at stroke units, early rehabilitation in the first hours, and specialized rehabilitation, including community rehabilitation and reintegration. Early mobilization should start in most patients 18–24 hours after the stroke.
Prognosis and Prediction
A very important aspect is to give a prognosis for recovery and rehabilitation for patients after stroke even if the acute phase has just started. Longitudinal studies which started with the first day after stroke clearly demonstrated that the continuum of rehabilitation after stroke is not linear, but follows a non-linear pattern [8]. It seems clear that the main effects of rehabilitation after stroke can be found in the first 3 months after stroke [8].
The prediction of gait arm function will be explained in our case example.
Our patient wants to know how good or bad his prognosis is. He thinks that the size of his brain lesion should provide some information in this regard. At the stroke unit he has a large MCA infarct and the MRI shows a large infarct volume. But is the infarct volume alone a very good predictor? Several studies have examined the predictive value of the infarct volume and the evidence is not very convincing [9].
One study at least concluded that neuroimaging variables on conventional MRI scans do not have added value in long-term prediction of activities of daily living (ADL) and that clinical variables in the second week after stroke are good predictors for independency in ADL 1 year after stroke [10].
Our patient has, however, lost much strength in his left leg and also cannot hold sitting balance for 30 seconds. This means that his prognosis for regaining normal ambulatory function is not very good and specific interventions are needed to improve the balance to reach gait ability in this person. A recent study showed with a multivariable modeling approach that patients with an independent sitting balance (trunk control test-sitting; 30 seconds) and strength of the hemiparetic leg (motricity index leg; e.g. visible contraction for all three items, or movement against resistance, but weaker for one item) on day 2 post-stroke had a 98% probability of achieving independent gait at 6 months [11]. It was shown that in absence of these features in the first 72 hours after stroke the probability of independent gait performance was just 27%, declining to 10% by day 9 after stroke [11].
Our patient has also clear motor deficit in his hand and arm – the hand-arm strength reaching from 2 to 4 points on a five-point MRC scale. What does this mean for regaining his hand-arm function?
According to a recent study describing a SAFE algorithm based on two simple bedside tests, finger extension and shoulder abduction, the functional recovery of the hemiplegic arm at 6 months can be predicted early in a hospital stroke unit within 72 hours after stroke onset [12]. In detail, for our patient who has some voluntary extension of the fingers and some abduction of the hemiplegic shoulder on day 2, he has a probability of 0.98 of regaining some dexterity at 6 months [12]. If he had no voluntary function in his left fingers and shoulder, the probability would be as low as 0.25.
Scrutinio et al. very recently described a prediction model [13] for patients during inpatient rehab after stroke. The first model included age, time from stroke onset to rehabilitation admission, motor and cognitive Functional Independence Measure scores at admission to rehabilitation, and neglect. The second model included age, male gender, time since stroke onset, and admission motor and cognitive Functional Independence Measure score. In this study both models demonstrated excellent discrimination [13].
Predicting how to recover from aphasia is much more difficult. A number of studies conclude that a prediction of aphasia recovery is currently too difficult [14, 15].
There are some studies for the prediction of outcomes for people with dysphagia. One study investigated 20 patients with stroke with videofluoroscopy to assess dysphagia. They found as prognostic factors for persisting aspiration infarcts of the posterior vascular territory lesions, oropharyngeal reflex abolition, palatoglossal seal alteration, and pharyngeal delay time at baseline [16]. Another study followed a cohort of 128 patients where swallowing function was assessed clinically and videofluoroscopically, within a median of 3 and 10 days, respectively, of stroke onset, using standardized methods and diagnostic criteria [17]. The predictors of subsequent swallowing abnormalities and complications were videofluoroscopic evidence of delayed oral transit, a delayed or absent swallow reflex, and penetration [17].
Assessment
The consequences and the sequelae after acute stroke should be classified according to the WHO’s international classification of functioning (ICF) [4]. The ICF has three main domains from body function, activity, and participation, and two subdomains, and provides a framework for the effects of stroke (the pathology) on the individual in terms of
body functions and structure
activities (related to tasks and actions by an individual) and
participation (involvement in a life situation).
The ICF also provides additional information on contextual and environmental factors.
A very good and illustrative example for the application of the ICF can be found by Langhorne and colleagues (Table 25.1) [4].
Table 25.1 The most relevant parameters after stroke and common and relevant scales per category
ICF category | Parameter | Commonly used assessments/scales |
---|---|---|
Body structures and functions | Most relevant structures affected
| Neurological scales
|
Activities | Most relevant activities affected
| Global ADL scales
|
Participation | Most relevant restrictions in participation
|
|
Environmental and personal contextual factors | Most common affected contextual factors (environmental and personal)
| Contextual factors
|
Different prediction models and assessments can give a prognosis for recovery and rehabilitation for patients after stroke even if the acute phase has just started. The WHO’s international classification of functioning (ICF), for example, has three main domains – body function, activity, and participation – and should be used to classify the consequences of an acute stroke (Table 25.1).
Principles of Stroke Rehabilitation
Current guidelines for rehabilitation and recovery after stroke conclude that the provision of comprehensive rehabilitation programs with adequate resources, dose, and duration is an essential aspect of stroke care [18]. Rehabilitation after stroke should be given in a stimulating environment to facilitate neural reorganization and to improve functional recovery [19]. The rehabilitation environment should include the appropriate delivery of tailored, individual, motivating, and challenging task-specific training approaches in order to provide an effective and efficient training that promotes motor skill acquisition [19, 20]. In general, patients after stroke have to be seen as active learners and not as passive participants. In this vein the challenge of rehabilitation after stroke is to provide those active learners with appropriate opportunities for intensive exercises and therapy, in particular to improve motor learning, plasticity, and important adaptations [19, 20]. Task-oriented training is nowadays seen as one of the most beneficial rehabilitation approaches to improve ADL after stroke [18–21]. The process of task-specific motor learning is dependent on mechanism of plasticity and also on adaptations of the central nervous system and adaptive physiological changes, e.g. to muscles and connective tissue [19, 22]. Task-specific training after stroke is a stimulus to learning, for the acquisition of skills, and might increase strength, endurance, and also fitness [21]. Task-specific training optimizes motor performance in functional and meaningful actions and may improve secondary adaptations associated with physical inactivity, may improve the biomechanics of functional actions, and may influence brain reorganization and neuroplasticity after stroke [19]. Brain reorganization and neuroplasticity after stroke may include changes that accompany brain recovery, repair, and also adaption and compensation [23]. For instance, in the very early phase after stroke there are a number of injury-induced changes with the potential for plasticity, including exuberant production, then activity-based pruning of new synapses or upregulation of specific growth factors [19]. In general, neural processes of neuroplasticity after stroke are relatively consistent with the neural processes that implement skill acquisition in healthy motor learners [21, 24–27]. Practice, easily measured with the number of successful repetitions or attempts during task-specific training, is generally considered one of the most critical contributors to motor learning [28, 29]. More specifically, to improve walking function after stroke, practice should be activity-specific and functional, and progressively more difficult and challenging, and of sufficient intensity, frequency, and duration [18].
We have now very good evidence for the effectiveness of practice from several studies and systematic reviews [30]. For instance, the current evidence of physiotherapy after a stroke encompasses more than 53 different interventions in almost 500 studies involving more than 25 000 patients [30]. The effect sizes for treatment after stroke are, however, at best moderately high and some studies even suggest that therapies applied during rehabilitation account for just 5–15% of the improvement after a stroke [31, 32].
However, it has been pointed out that a large number of studies used inadequate methods and were poorly conducted, and thus positive results were implausible. For example, inadequate measurement methods, therapies, and comparisons between individual therapies were used in many studies. Similarly, recovery time after stroke has not been sufficiently taken into account in many clinical studies and some studies have not been sufficiently planned and the dosing parameters were not well defined (possible recent examples are the ATTEND trial [33], the ICARE trial [34], and the EVREST trial [35]).
The main weaknesses of many studies can be described as follows: (1) often physiotherapy was not sufficiently dosed (regarding frequency, duration, extent, and also intensity); (2) the time of physiotherapy after a stroke was not adequately determined; (3) the measurement instruments used in studies were unsuitable for measuring changes; (4) patients and their potential in physiotherapy were insufficiently categorized; and (5) the underlying theories of therapy were vague.
Therefore, one significant aim for the future is to initiate high-intensity studies with clear disease-specific and temporal implications. Patients should therefore not all receive the same treatment after a stroke, but an adequate and different therapy approach depending on their initial severity and individual prognosis.
It should also be critically outlined that differentiation between recovery (restitution) and substitution (compensation) is a difficult concept [36]. Improvement after a stroke is mainly if not entirely due to substitution (compensation) of function and activities [36] and there is so far not a single therapeutic approach that has been proved and can lead to “real” restitution after stroke. The aim of neurorehabilitation should be, therefore, to provide a framework of evidence-based interventions to improve ADL which are important for individual patients and to allow participation in real life (e.g. occupational work, self-care, housework, recreation and leisure activities, and fulfilling the roles of family and/or partnership).
Patients should receive an adequate and different therapy approach depending on their initial severity and individual prognosis after a stroke.
Upper Motor Neurone Syndrome – Positive, Negative, and Adaptive Features
Lesions involving the corticospinal pathways can occur at any level – in the cortex, internal capsule, brainstem, or spinal cord. In neurology, since Hughlings Jackson’s contributions [37], the dyscontrol characteristics associated with the upper motor neuron (UMN) syndrome have been referred to as either negative features (such as impaired muscle activation, weakness or paralysis, and loss of motor control) or positive features (such as stretch-sensitive muscle overactivity or spasticity) [38, 39]. Additionally, there is a third group referred to as adaptive features, since it is evident that adaptive physiological, mechanical, and functional changes develop in the neural system, the muscles, and other soft tissue during the post-lesion period [40–42].
Positive Features
Historically, the positive features have been considered the result of abnormal excitability of stretch reflexes (also called reflex hyperactivity) [42]. The somewhat technical definition of spasticity developed by a consensus of neurologists [40, 41] and reported by Lance is “a motor disorder characterized by a velocity-dependent increase in tonic stretch reflexes (‘muscle tone’) with exaggerated tendon jerks resulting from hyperexcitability of the stretch reflex as one component of the upper motor neuron syndrome” [43].
Quantification of spasticity remains somewhat dissatisfying [44]. Previous efforts have concentrated on subjective clinical measures such as the Modified Ashworth Scale (MAS) or on more objective measures such as the electrophysiological reflex studies and biomechanical analysis of limb impedance to mechanical perturbation, voluntary movement, or gait [45, 46]. In spite of these efforts, no uniformly useful objective measurements have emerged, and therefore clinical measures such as the MAS are widely used. In recent years, the Tardieu Scale, first described by Tardieu et al. in 1954 [47] and later modified by Held et al. [48] (and therefore called, e.g. in France, the Held Test), has been suggested to be more appropriate for use in the measurement of clinical spasticity [49, 50]. The data collection procedure involves the use of two speeds of passive movement (one very slow, the other as fast as possible) and recording the angle at which a clear “catch” in the passive movement is felt (quality of muscle reaction and angle of muscle reaction are documented) [51]. In children with cerebral palsy, Boyd and Graham showed that the Modified Tardieu Scale (MTS) may have less variance and might be more reliable than the MAS [52]. When comparing the reliability of the MTS and the MAS in adult patients after brain injury to quantify spasticity, the MTS yields significantly better results compared to the MAS [53].
According to O’Dwyer and other researchers, it remains open if spasticity per se should be treated in rehabilitation [41]. In other words, it is questionable whether spasticity should be the main target of rehabiliation.
Other positive features includes cocontraction/coactivation of agonist and antagonist muscles and so-called associated movements (AM) [42]. Such AMs are unintended movements that accompany, but are not necessary for, volitional movement, and they are also seen in able-bodied patients in complex tasks, under stress, and when generating maximum force levels [54, 55]. AMs are illustrating lack of skill in attempts at a specific task and can be easily seen as children grow up. It is important to reflect that there is no evidence to support the idea that associated movements are a manifestation of spasticity [42, 55, 56]. This is contrary to the outdated and obsolete beliefs of some therapeutic schools (e.g. Bobath) [42]. Some research even indicates that associated movements themselves are a positive prognostic marker for recovery of upper limb function after stroke [57].
It seems now clear that the positive features described above should not be the first target of neurorehabilitation when aiming to improve ADL.
Negative Features
Following a brain injury, there may be insufficient descending impulses converging on the motor neuron population either to activate muscles or to increase the firing rate or frequency of active motor units [42], which results in a decreased muscle activation and therefore weakness, but also in slowness of movement and loss of motor control (e.g. incoordination).
Although weakness directly affects motor function, it is also the slow build-up to peak force that is a very critical parameter for slowness of movements after stroke, e.g. in walking [42]. Negative features result in a loss of motor control which is illustrated by the inability to generate and time muscle forces, and to control these and other forces generated by the movement of many body segments and gravity, in order to produce effective motor performance [42]. It is now very clear that the above-described negative features should be the most important target of neurorehabilitation to improve ADL.
Adaptive Features
In many patients the immobilization of paretic limbs during the acute phase in hospital and rehabilitation alters mechanical properties of the muscles. Both reflex hyperexcitability and altered mechanical properties lead to muscle hypertonia [42], which is defined as an increased resistance to passive stretch. In prolonged immobilization, the constant flexed joint position, a transformation of the muscles, and changes in periarticular connective tissue lead to a shortening of muscles and connective tissue, resulting in reduced active and passive joint mobility [40, 41, 53, 58, 59]. The reduced passive range of motion (ROM) is defined as contracture [40, 41]. Muscle contractures and spasticity often complicate patient care, delay early rehabilitation, and/or reduce function of the limbs [45, 60, 61].
Our patient has slightly increased tone in his affected arm, especially in hand and finger flexors and also in the flexors of the elbow (positive features). If he tries to lift his affected arm it looks very uncoordinated and he uses his shoulder and trunk very much to elevate his affected arm (the movement pattern, not a positive feature per se). It also takes a very long time for him to reach a target with his affected hand (slowness of movement and loss of dexterity are typical negative features). If the therapist tries to stretch the patient’s elbow muscles he feels a slightly increased resistance and the range of motion is slightly restricted (this is the adaptive feature: hypertonia, as defined as an increased resistance to passive movement, and also contracture, because the full range of motion is not reached). After his assessment the physiotherapist chooses a couple of task-specific exercises to improve coordination, velocity of movement, arm strength, and dexterity while reaching targets.
The dyscontrol characteristics associated with the upper motor neuron (UMN) syndrome have been referred to as negative features (e.g. weakness), positive features (e.g. spasticity), and adaptive features (e.g. contractions). Negative features should be the most important target of neurorehabilitation to improve activities of daily living.
Current State-of-the-Art Approaches in Rehabilitation after Stroke
Current recommendations and guidelines for rehabilitation and recovery after stroke recommend science- (evidence-) based rehabilitation programs [18, 62–64]. In the following paragraphs scientific evidence about the effects of current approaches in neurorehabilitation and their potential to drive motor learning and recovery will be summarized.
Approaches to Improve Sit-to-Stand, Gait, Balance, and Mobility
There are many interventions for improving sitting to standing, gait, balance, and mobility after stroke and a list of all the interventions would go beyond the scope of this chapter. Therefore, only a few promising interventions are discussed here.
Sitting to Standing
Standing up from a seat is one of the most frequently performed tasks in daily life. The ability to move from sitting to standing, as an essential prerequisite to walking, is often overlooked in clinical settings. Furthermore, the ability to sit-to-stand independently is very important for independent living and preventing falls [65].
A recent Cochrane review included 13 studies with a total of 603 participants. Only one study, with a total of 48 participants, reported the ability to sit-to-stand independently as an outcome measure, and found that training increased the odds of achieving independent sit-to-stand compared with control (odds ratio [OR] 4.86, 95% confidence interval [CI] 1.43–16.50) [65]. Training for sit-to-stand improved the time taken to sit-to-stand and the weight distribution between the legs during sit-to-stand, and these improvements were maintained at long-term follow-up [65].
There have only been a few trials assessing the effect of sit-to-stand training on peak vertical ground reaction force (one study, 54 participants) and functional ability (two studies, 196 participants) and no effect of sit-to-stand training on the number of falls was found.
Mobility
A recent Cochrane review attempted to determine whether physical rehabilitation approaches are effective in recovery of function and mobility in people with stroke, and wanted to determine if any one physical rehabilitation approach is more effective than any other [66].
The authors identified 96 studies, involving 10 401 stroke survivors, and concluded that it appears to be most beneficial when the therapist selects a mixture of different treatments for an individual patient from a wide range of available treatments. The authors did not find evidence showing that one physical rehabilitation approach was more effective than any other [66].
Gait
Electromechanical-Assisted Gait Training
One gait-training approach involves electromechanical-assisted gait training to improve walking function. In the last decades electromechanical-assisted gait and walking training has been developed as an adjunct to overground gait training after stroke [67]. This consists of either a robot-driven exoskeleton orthosis [68] or an electromechanical end-effector solution, with two driven foot plates simulating the phases of gait [69]. One example of exoskeleton-based electromechanical gait rehabilitation is the “Lokomat” [68]. A robotic gait orthosis combined with a harness-supported body weight system is used together with a treadmill. However, the main difference compared with treadmill training is that the patient’s legs are guided by the robotic device according to a preprogrammed gait pattern. A computer-controlled robotic gait orthosis guides the patient and the process of gait training is automated [69]. An example of an end-effector system is the “Gait Trainer,” which is based on a double crank and rocker gear system [69]. In contrast to a treadmill, the electromechanical “Gait Trainer” or the “GEO” (Figures 25.1, 25.2) consists of two foot plates positioned on two bars, two rockers, and two cranks, which provide the propulsion. The harness-secured patient is positioned on the foot plates, which symmetrically simulate the stance and swing phases of walking [69] and a servo-controlled motor guides the patient during walking exercise. During gait training the vertical and horizontal movements of the trunk are controlled in a phase-dependent manner. In both examples the process of gait training is automated and is supported by an electromechanical solution.
Figure 25.1 Example of an end-effector system, “GEO,” for the electromechanical-assisted gait training after stroke, frontal view.
Figure 25.2 The end-effector system “GEO” for the electromechanical-assisted gait training after stroke, lateral view.
Other similar, more recently developed electromechanical devices include the “Haptic Walker” [70], and also in recent years powered (mobile) exoskeleton solutions [71, 72], which will be described in detail below.
Electromechanical and robotic devices (such as those described above) can be used to give non-ambulatory patients intensive practice (in terms of high repetitions) of complex gait cycles. The advantage of these electromechanical devices, compared with treadmill training with partial body weight support, may be the reduced effort required of therapists, as they no longer need to set the paretic limbs or assist trunk movements [73]. A recent update of our Cochrane review included 23 randomized controlled trials (RCTs) involving 999 participants [67]. As a main result, electromechanical-assisted gait training in combination with physiotherapy increased the odds of participants becoming independent in walking (OR [random effects] 2.39, 95% CI 1.67–3.43, p <0.00001), but did not significantly increase walking velocity (mean difference [MD] = 0.04 meters/s, 95% CI 0.03–0.11, p = 0.26) or walking capacity (MD = 3 meters walked in 6x minutes, 95% CI 29–35, p = 0.86). The results, however, should be interpreted with caution because some trials investigated people who were independent in walking at the start of the study, variations exist between the trials with regard to devices used and duration and frequency of treatment, and some trials included devices in combination with functional electrical stimulation. The pre-planned subgroup analysis suggests that people in the acute phase may benefit from electromechanical-assisted gait training, but people in the chronic phase may not. Further post-hoc analysis showed that people who were non-ambulatory at intervention onset may benefit, but ambulatory people may not benefit from electromechanical-assisted gait training. There were no differences between the types of above-mentioned devices used in studies regarding ability to walk alone. In contrast, they found significant differences between devices in favor of end-effector devices for improving walking velocity after stroke.
Treadmill Training With or Without Body Weight Support
Another gait-training approach for walking after stroke is treadmill training with or without body weight support. In a recently published Cochrane review, the authors identified 56 relevant trials, involving 3 105 participants. Twenty-six studies (1 410 participants) compared treadmill training with body weight support to another physiotherapy treatment; 20 studies (889 participants) compared treadmill training without body weight support to other physiotherapy treatment, no treatment, or sham treatment; two studies (100 participants) compared treadmill training with body weight support to treadmill training without body weight support.
As a key result, the authors found that people after stroke who receive treadmill training with or without body weight support are not more likely to improve their ability to walk independently [74]. However, treadmill training with or without body weight support may improve walking speed and walking capacity compared with not receiving treadmill training at all. More specifically, people after stroke who are able to walk at the start of therapy appear to benefit most from this type of intervention, but people who are unable to walk independently at therapy onset do not benefit [74].
For people treated at a later stage (more than 6 months after their stroke), the effects were less significant. More frequent treadmill training (e.g. five times per week) appears to produce greater effects on walking speed and endurance. Brief periods of treadmill training (duration of 4 weeks) provided a modest improvement in walking speed, but not enough to be clinically important. Effects of the age of participants or the type of stroke were not investigated in this review.
As advice for clinical practice: it appears that people who can walk after stroke, but not those who cannot, may benefit from treadmill training (with and without body weight support) to improve their walking abilities. Further research should specifically investigate the effects of different frequencies, durations, or intensities (in terms of speed increments and inclination) of treadmill training, as well as the use of handrails.
Powered Robotic Exoskeletons
Relatively new technologies for people after stroke are so-called powered robotic exoskeletons [71, 72]. These “mobile” solutions for electromechanical-assisted gait training in principle offer the potential to increase repetitive walking practice. However, research-based recommendations for the use of “mobile” robotic exoskeletons for gait rehabilitation after stroke are lacking. One recent review found that “mobile”-powered exoskeletons are feasible if used for gait training of patients with lower extremity paresis in a professional setting [72]. The reviewer states that beneficial effects on gait function and independence in walking were observed, but data are not mature enough to allow any insightful conclusions [72]. A recently published scoping review identified 11 studies in the field, which were all published within the last 5 years, involving 216 participants [71]. Only three of the included studies, however, were performed along an RCT fashion, but also with a low number of participants. The authors reported an improvement with mobile exoskeleton-based gait training as more apparent in the subacute phase after stroke. In additional, these studies observed that these powered robotic exoskeletons can be used relatively safely for gait training after stroke [71]. In summary, we are a long way from making recommendations on an evidence-based level on “mobile”-powered exoskeletons for clinical routine. Furthermore, significant concerns still exist with regard to the cost-effectiveness of using such devices in clinical practice.
Network Meta-Analysis Including All Gait-Training Approaches
More recently, a systematic review of RCTs with network meta-analysis was undertaken [75]. The authors were able to include 95 RCTs with a total of 4 458 patients after stroke. For the primary outcome of walking speed, end-effector-based electromechanical-assisted gait rehabilitation reached significant improvements compared to conventional walking rehabilitation (MD = 0.16 m/s, 95 % CI 0.04–0.28). All other interventions did not improve walking speed significantly. For the secondary outcome of walking distance, both end-effector-assisted training and treadmill training with body weight support increased the distance walked significantly more than conventional rehabilitation (MD = 47 m, 95% CI 4–90 and MD = 38 m, 95% CI 4–72, respectively). The authors conclude that in a comparison of different gait therapy approaches after stroke, gait rehabilitation with end-effector devices seems to be advantageous for improving walking speed, and treadmill therapy with body weight support or with speed approach is advantageous for improving gait capacity [75].
A protocol for a so-called speed-dependent treadmill training is provided in the case study (according to [76]).
Because our patient walks very slowly (below 5 m/s) and he cannot walk more than 20 m without pausing, the therapist offers him a special treatment protocol.
Our patient gets the following gait-training protocol from his therapist on 3 days a week for 30 minutes of therapy (speed-dependent treadmill training [76]):
warm-up on the treadmill (5 minutes)
Vt1 (maximum over ground walking speed).
During a period of 1–2 minutes, the belt speed is increased, via communication with the patient, to the highest speed at which the patient can walk safely and without stumbling (hold Vt1 for 10 seconds), followed by a recovery period.
Vt2 Increase speed by 10% during the next attempt.
(Vt1 + 10% = Vt2)
Vt3 Increase speed by 10% during the next attempt.
(Vt2 + 10% = Vt3)
Vt4 Increase speed by 10% during the next attempt.
(Vt3 + 10% = Vt4)
Vt5 Increase speed by 10% during the next attempt.
(Vt4 + 10% = Vt5) Next session use Vt5 instead of Vt1
Cool down for the rest of the session.
Our patient uses this walking training protocol three times per week over 2 weeks (on Monday, Wednesday, and Friday) [76].
There are many interventions for improving sit-to-stand, gait, balance, and mobility after stroke. It appears to be most beneficial when the therapist selects a mixture of different treatments for an individual patient from a wide range of available treatments, including electromechanical and robotic devices and treadmills.
Hand-Arm Training
There are many interventions for improving upper limb function after stroke and a list of all the interventions would go beyond the scope of this chapter. Therefore, only a few promising interventions are discussed here. For an evidence-based overview, the reader is referred to an outline of arm-training approaches [77].
Forced Use, Constraint-Induced Movement Therapy
Constraint-induced movement therapy (CIMT) was developed to overcome a learned non-use and upper limb impairments after stroke and is according to a recent systematic review the most investigated intervention for the rehabilitation of patients [78]. The original version of CIMT includes constraining of the non-paretic arm and 6 hours of task-oriented training. Later modified versions (mCIMT) constrained also the non-paretic arm, but with less intensive additional treatment (30 minutes to 3 hours) as the original CIMT. When the so-called “forced use therapy” is applied, the non-affected arm is constrained in rehabilitation without any additional therapy (constraint alone as therapy). According to a recent review the original and modified types of CIMT have beneficial effects on motor function, arm-hand activities, and self-reported arm-hand functioning in daily life, immediately after treatment and at long-term follow-up, whereas the authors do not report evidence for the efficacy of constraint alone [78]. The review authors found, somewhat surprisingly, that the type of CIMT, timing, or intensity of practice does not seem to affect patient outcomes [78]. Almost all studies about this type of therapy were undertaken in inpatient settings, although recently, a randomized trial reported small but significant effects of patients’ self-reported quality of movement when they used CIMT in an outpatient setting [79]. Other important parameters such as daily activities or arm function did not improve [79].
Impairment-Oriented Therapy
After stroke there are many people with either mild or severe arm activity limitations, but fewer with moderate activity limitations [80]. These patient subgroups have different motor control deficits and accordingly different therapeutic needs [80–82]. Thus, a specific training concept would have to provide at least two training strategies in order to improve arm paresis after stroke [80, 82]. However, specific and highly structured unilateral training methods for the paretic arm have been developed, such as Arm Ability Training (AAT) for people with mild arm paresis and Arm Basis Training (ABT) for people with severe arm paresis [83]. AAT, for example, targets different sensorimotor abilities, e.g. speed of finger movements, grasping, dexterity, steadiness, tracking, aiming, and endurance in a systematic standardized repetitive training structure with eight training tasks [80, 83]. ABT, however, focuses on the capacity for selective movements in all segments of the arm within a systematic repetitive training structure, starting with single joint movements without anti-gravity control, followed by single joint movements with anti-gravity control to multi-joint movements as individually indicated [80, 83]. Overall, three RCTs and at least one meta-analysis using individual patient data [81] supported the clinical efficacy of AAT and ABT: AAT improved dexterity and timed performance with everyday arm activities in people with mild paresis [81, 84, 85], while ABT improved the capability for selective arm movements in people with severe paresis [84, 86]. Furthermore, a recent systematic review concluded that there is moderate- to high-quality evidence indicating that Bobath therapy might be inferior compared to AAT for treating upper limb motor impairment and disabilities in acute, subacute, and chronic stroke [87].
Mirror Therapy
As an alternative treatment approach, especially for the more severely affected patients, mirror therapy has been proposed as potentially beneficial [88, 89]. In contrast to other interventions mirror therapy is based on visual stimulation, as during the therapy, a mirror is placed in the patient’s midsagittal plane, thus reflecting the non-paretic side as if it were the affected side [90]. Now the movements of the non-paretic limb create for the patient the illusion of normal movements of the paretic limb. Recent clinical trials reported effects of mirror therapy on motor function, ADL, and pain reduction in arm amputees or CRPS-type I [90–93].
New developments in this field describe “mirror-like” video or computer graphic set-ups, where a video or computer graphic image of the moving limb is presented as if it were the opposite one [93].
Although much work was done on how this type of therapy might work, the exact mechanisms of the effect of mirror therapy in patients, e.g. after stroke, remain unclear [93]. The visual mirror illusion of the paretic limb might be perceived by the patient’s own moving limb, and might prevent or even reverse a learned non-use of the paretic limb. Mirror therapy might directly stimulate motor recovery, but is also seen by some as a variant of motor imagery training [93].
A recent updated Cochrane review about mirror therapy found 62 relevant studies, involving a total of 1 982 participants with a mean age of 59 years (45–73 years) in subacute and chronic stages after stroke. Mirror therapy was provided three to seven times per week, between 15 and 60 minutes per session for 2–8 weeks (mean five times per week, 30 minutes per session for 4 weeks) [93]. As a key result, it was shown that mirror therapy moderately improved movement of the affected upper and lower limb and the ability to carry out daily activities for patients within and also after 6 months post-stroke [93]. Mirror therapy reduced pain after stroke, but mainly in patients with a complex regional pain syndrome (CRPS) [93]. No clear effect could be found regarding visuospatial neglect. The beneficial effects on movement were maintained in some studies for 6 months and adverse effects were not reported [93].
Electrical Stimulation
Therapeutic electrical stimulation after stroke applied as sensory electrical stimulation or direct muscle (or functional motor) electrical stimulation can be applied in rehabilitation [87].
A recent systematic review including seven RCTs (n = 347) found moderate-quality evidence that high-frequency TENS (100 Hz) in combination with (in addition to) rehabilitation treatment is superior to the rehabilitation treatment alone to improve upper extremity impairments and disabilities [87]. Furthermore, they found moderate-quality evidence that electro-acupuncture (2–3 Hz) in combination with (in addition to) rehabilitation treatment is superior to the rehabilitation treatment alone.
Another well-known therapy approach is neuromuscular electrical stimulation (NMES) over a muscle (neuromuscular endplate), which induces muscle contractions at frequencies of 10–50 Hz. NMES can be passive or triggered by voluntary muscle contraction. According to a systematic review NMES appears to be valuable and could be integrated as an adjuvant (i.e. additional) therapy into stroke rehabilitation to improve upper limb motor impairments [87]. There are, according to the same review, insufficient arguments for integrating low-frequency TENS, EMG-NMES, or positional feedback NMES as an adjuvant therapy into stroke rehabilitation [87].
Electromechanical-Assisted Arm Training
Electromechanical and robot-assisted arm training uses specialized machines to assist rehabilitation in supporting shoulder, elbow, or hand movements. Examples of electromechanical and robot-assisted arm training devices are as follows: the Mirror Image Motion Enabler (MIME) [94]; the InMotion robot (Massachusetts Institute of Technology [MIT-Manus]) [95]; the Assisted Rehabilitation and Measurement (ARM) Guide [96]; the Robotic Rehabilitation System for upper limb motion therapy for the disabled (REHAROB) [97] and the Neuro-Rehabilitation-Robot (NeReBot) [97]; the Bi-Manu-Track (Figure 25.3) [73]; the Robot-mediated therapy system (GENTLE/s) [98]; the Arm robot (ARMin) [99]; and the Amadeo [100]. Most of these devices provide passive movement of the person’s arm, but some devices assist arm movements or provide resistance from reaching-like movements [94]. The progression of practice with the device is possible by, for example, varying the force, decreasing assistance, increasing resistance, and expanding the movement amplitude. Moreover, some devices, such as the Bi-Manu-Track and the MIME, may be used to provide bimanual exercise: the device simultaneously moves (mirrors) the affected limb passively, steered by the non-paretic limb. Very broadly reflected, most robotic systems incorporate more than one modality into a single device.
Figure 25.3 The Bi-Manu-Track robotic-arm trainer providing distal and bilateral training, as an example for electromechanical-assisted arm rehabilitation.
The main advantage of these electromechanical and robot-assisted arm training devices lies in an increase of motivation to train and also, importantly, more repetitive practice and independent exercise [101–103]. Therefore, electromechanical-assisted training devices allow a therapy paradigm that is intensive, frequent, and repetitive, and adheres to the principles of motor learning.
In the latest updated Cochrane review the authors were able to include 45 RCTs (involving 1 619 participants) [103]. The mean age of participants in the included studies ranged from 21 to 80 years. The duration of the study interventions was heterogeneous, ranging from 2 and 3 to 12 weeks. The included studies described and used in sum 19 different electromechanical devices; however, the most often used devices were the Bi-Manu-Track, the InMotion, and the MIT-Manus. For most trials the frequency of treatment was five times per week. The duration of treatment ranged from 20 and 30 to 90 minutes each working day, or even 90–105 minutes each day. As a main result, electromechanical and robot-assisted arm training improved ADL scores (standardized mean difference [SMD] 0.31, 95% CI 0.09–0.52, p <0.001), arm function (SMD 0.32, 95% CI 0.18–0.46, p <0.001), and arm muscle strength (SMD 0.46, 95% CI 0.16–0.77, p = 0.003). Electromechanical and robot-assisted arm training did not increase the risk of participant drop-out, and adverse events were rare. A somewhat surprising finding was that devices using a proximal tactic compared with devices using a distal method did not differ in the main results. This means that hand-finger robots did not improve only hand-finger function, and shoulder and elbow robots did not improve only shoulder function. A test for subgroup differences between a subgroup of participants who received mainly training for the distal arm and the hand (finger, hand, and radio-ulnar joints) and a subgroup of participants who received training mainly of the proximal arm (shoulder and elbow joints) revealed no significant difference in ADL (p = 0.64). The authors conclude that people after stroke who receive electromechanical and robot-assisted arm and hand training might improve their ADL, arm and hand function, and arm and hand muscle strength. The results should be, however, interpreted with caution because the quality of the evidence was low to very low. Furthermore, there were variations between the trials in the intensity, duration, and amount of training, but also the type of treatment and participant characteristics. As adverse events such as injuries and pain were rarely described, these devices can be applied as a rehabilitation tool.
In practice, electromechanical or robot-assisted arm training could increase the intensity of arm therapy. Perhaps more repetitions in the same time of therapy can be achieved if electromechanical and robot-assisted therapy is given. One should keep in mind that electromechanical and robot-assisted therapy uses devices simply as “vehicles” to apply an increased intensity of practice in terms of many repetitions of arm training [101, 104]. It seems unlikely that motor therapy provided by robots will lead to better results than motor therapy provided by humans under the premise that intensity, amount, and frequency of therapy are exactly comparable [103]. Electromechanical devices could, therefore, be used as an adjunct to conventional therapies. Additionally, electromechanical and robot-assisted training could create additional costs of rehabilitation after stroke. The general applicability of robot therapy might, therefore, be limited simply due to lack of access to devices, e.g. in many low-income countries, and there also appear to be fewer opportunities for therapists and patients to access robots in outpatient than in inpatient settings. All of these points taken together might limit the applicability of this type of therapy in day-to-day clinical routine.
Our patient is not prescribed any robot-assisted arm training (although available in inpatient center) because his affected arm has recovered very well and he is focused during therapies on his dexterity in daily life situations and on improving appropriate speed of movement of reaching and grasping objects.
The many existing interventions for improving upper limb function include constraint-induced movement therapy (CIMT), impairment-oriented therapy, mirror therapy, electrical stimulation (TENS, NMES), and robot-assisted arm training.
Role of Somatosensory Rehabilitation
How do somatosensory deficits in the arm and hand relate to upper limb impairment, activity, and participation problems after stroke? A recent review attempted to answer this question and included six articles about the possible relationship between somatosensory deficits and activities after stroke [105]. The authors found that two-point discrimination was shown to be predictive for upper limb dexterity, and somatosensory-evoked potentials were shown to have predictive value in upper limb motor recovery. Proprioception was significantly correlated with the perceived level of physical activity and social isolation and had some predictive value in functional movements of the upper limb. Finally, the combination of light touch and proprioception impairment was shown to be significantly related to upper limb motor recovery, as well as disability situations during ADL [105].
Although a large variation in results was found due to heterogeneity of the studies, somatosensory deficits were shown to have a role in upper limb motor and functional performance after stroke [105].
Sensory deficits might play a role in recovery, but what are the best up-to-date interventions to improve sensory capacity after stroke? There are not many RCTs about this topic. There is, however, one remarkable randomized double-blind study which compared the effects of a perceptual-learning-based sensory discrimination program with a non-specific exposure to sensory stimuli via passive movements and grasping of common objects [106]. The authors included 50 people after stroke with impaired texture discrimination, limb position sense, and/or tactile object recognition (>6 weeks, median 48 weeks post-stroke) and randomized them to somatosensory discrimination training (n = 25) or to repeated exposure to sensory stimuli (n = 25) in 60-minute sessions for a total of 10 hours. The primary outcome in this study was the change in a composite standardized somatosensory deficit (SSD) index following intervention. Follow-up was at 6 weeks and 6 months post-training. At the end of the study the authors found a significantly greater improvement in sensory capacity following sensory discrimination training and improvement was maintained at 6 weeks and 6 months [106].
Physical Fitness Training for People after Stroke
According to a recent updated Cochrane review there is very good (1a – high level) evidence [107] that cardiorespiratory training reduces disability during or after usual stroke care.
The authors included 58 trials, involving 2 797 participants, which comprised cardiorespiratory interventions (28 trials, 1 408 participants) [107]. Global indices of disability show moderate improvement after cardiorespiratory training. Cardiorespiratory training involving walking improved maximum walking speed, preferred gait speed, and walking capacity [107]. The effects of this type of training on death and dependence after stroke are, according to the authors, still unclear and these outcomes are seldom observed in physical fitness training trials [107].
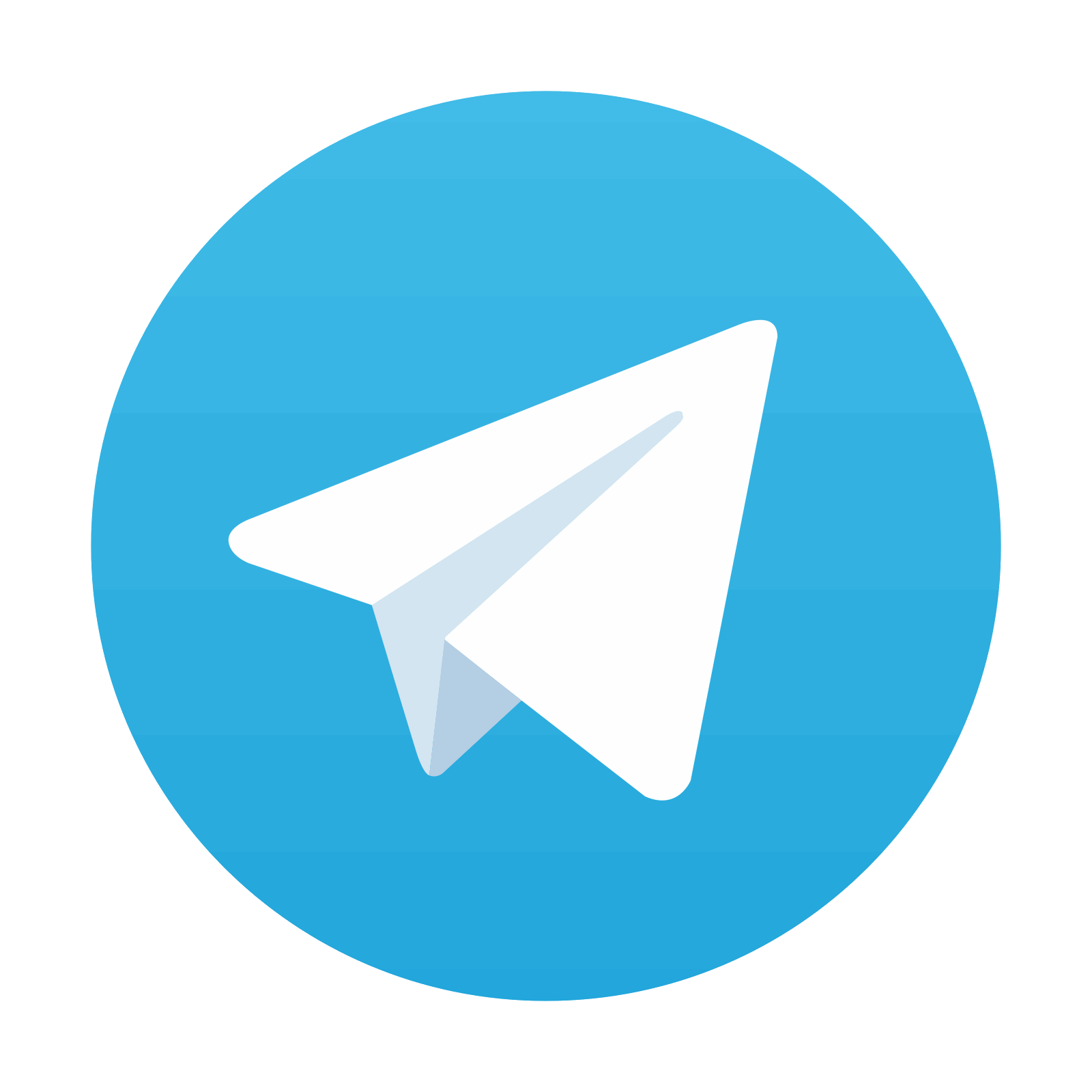
Stay updated, free articles. Join our Telegram channel

Full access? Get Clinical Tree
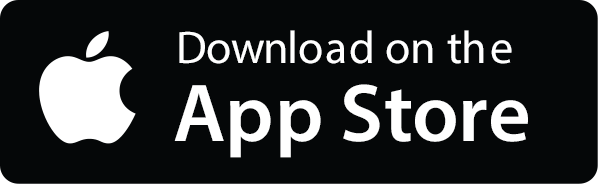
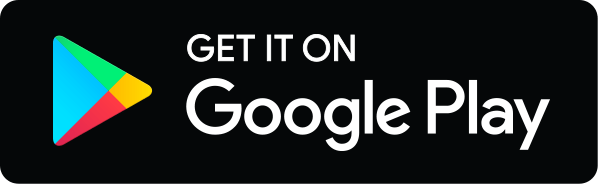
