Abstract
Non-invasive brain stimulation to stimulate neuroplasticity, enhance recovery, and improve mood after stroke has made substantial technical advances in the past two decades. The most common neuromodulatory techniques are transcranial direct current stimulation (tDCS), applying a weak electrical current across the brain, and transcranial magnetic stimulation (TMS), inducing an electrical field within the brain. Currently, the only non-invasive brain stimulation technique and indication for which there is a sufficiently strong evidence base to support routine use in clinical practice is transcranial magnetic stimulation to improve mood in post-stroke depression. TMS applied to dorsolateral prefrontal cortices can substantially reduce depressive symptoms, though not increase complete remission. TMS is a reasonable second-line intervention in patients with post-stroke depressed mood who have been resistant to pharmacotherapy. For several additional indications in post-stroke patients, both TMS and tDCS have shown signals of potential benefit in randomized trials. The strongest evidence is for enhancement of recovery of upper extremity motor function and hand dexterity with TMS. In addition, there is suggestive evidence for possible benefit in improving recovery of function after stroke in walking (TMS), activities of daily living (tDCS), aphasia (both), hemispatial neglect (both), and swallowing (both). However, for these and potentially other recovery-enhancing applications, substantial additional larger trials are needed.
Investigation into the merits of non-invasive brain stimulation as a therapeutic tool in stroke has gained momentum over the past two decades. These techniques include transcranial direct current stimulation (tDCS), high-definition tDCS (HD-tDCS), transcranial alternating current stimulation (tACS), transcranial random noise stimulation (tRNS), repetitive transcranial magnetic stimulation (rTMS), and theta burst stimulation (TBS). To date, the most studied modalities used to modulate brain excitability are tDCS and rTMS.
The use of cortical stimulation to facilitate stroke recovery is based on the premise that a focal lesion produces a region of decreased neuronal output and disrupts the balance of interhemispheric inhibition. Therefore, current delivered from an external source may facilitate a shift of this imbalance to a more equal state by increasing excitability of the perilesional areas and/or decreasing inhibition arising from the contralesional hemisphere (Schlaug et al., 2008).
Transcranial Direct Current Stimulation
The Stimulator
tDCS involves applying a weak, direct current of electricity, usually 1–2 mA, to the scalp via two rubber electrodes sheathed in saline-soaked sponges. The current flows in a direction away from the cathode to the anode; therefore, the effects depend on the position and polarity of the electrodes. The tDCS-stimulating device is a 13 cm × 21 cm portable box, which is typically programmed to ramp up the current over several seconds to minimize discomfort. Subjects experience a tingling/itching sensation under the electrodes, which abates in most cases by 1 minute owing to tolerance. The portability, ease of application, and relatively low cost of the device render it a practical therapy option for stroke clinicians.
Mechanisms of Action
Transcranial direct current stimulation is said to be neuromodulatory as it delivers subthreshold stimulation that affects the neuronal firing rate by altering the balance of ions inside and outside the neuronal membrane, producing hyperpolarization in the region of the cathode and depolarization of the resting membrane potential beneath the anode (Nitsche et al., 2003; Giordano et al., 2017). Sustained application (typically 10–30 min) generates changes at the synaptic level that persist transiently following the cessation of stimulation (Nitsche and Paulus, 2000). These effects are similar to those seen in long-term potentiation where there is a lasting increase in postsynaptic potentials. This in turn is capable of inducing cortical reorganization, most likely by increasing local synaptic effectiveness and network processing (Krause et al., 2013) and may be propagated in anatomically or functionally connected distant cortical and subcortical regions (Lang et al., 2005). Computational models have been developed that have improved our understanding of the effects of tDCS on single neurones, yet the mechanisms of how tDCS affects and interacts with a network or neuronal populations, particularly inhibitory interneurones, remain obscure (Radman et al., 2009).
In addition to effecting changes in neuronal membrane polarization, there are also non-neural cells and tissues (such as blood vessels and connective tissue) within the central nervous system that may also influence the effects of tDCS. These may influence physiological mechanisms such as vascular motility, inflammation, and cell migration, which would seem relevant in stroke recovery (Brunoni et al., 2012).
Application of tDCS
Electrode position and orientation will affect the outcome produced by tDCS, as different neuronal populations with different tissue characteristics will be stimulated; therefore, the induced currents may be distorted and unpredictable, particularly in the case of stroke in which the brain tissue may not be anatomically intact.
The most commonly used protocol for tDCS stimulation in stroke is the anodal montage with the anode positioned over the area of the scalp corresponding to the lesioned motor cortex (to increase excitability of the perilesional regions) and the cathode on the contralateral forehead. Conversely, cathodal stimulation involves application of the cathode over the region of the contralesional motor cortex and the anode on the contralateral forehead to downregulate excitability, thus releasing the lesioned hemisphere from excessive transcallosal inhibition (Boggio et al., 2007). A third variation places the anode on the lesioned cortex and the cathode on the non-lesioned cortex with the intent of achieving both anodal and cathodal effects simultaneously and is termed bihemispheric tDCS. Finally, extracephalic tDCS is a montage where the cathode is placed more distally, usually over the contralesional upper arm. This has the proposed advantage of avoiding unwanted excitability changes under the cathode but there is uncertainty regarding safety as current could potentially affect the brainstem (Redfearn et al., 1964) and the increased distance between the electrodes could negatively impact current density and therefore the duration of effects (Moliadze et al., 2010b).
There is an immense range of possible dose parameters (current intensity, duration, number of sessions, etc.) which provides flexibility but also presents a challenge in determining optimal dosage for individual clients (Peterchev et al., 2012).
Safety
Routinely used tDCS protocols appear to be safe with thousands of subjects tested worldwide and very few reported cases of adverse events. Furthermore, the induced effects, both electrophysical and behavioural, appear to be totally reversible (Liebetanz et al., 2009; Bikson et al., 2016; Antal et al., 2017). The exclusion criteria are very general and include unstable medical conditions, epilepsy, acute eczema at the electrode sites, metallic implants near the electrodes (e.g. cochlear implants, aneurysm clips), pacemaker, pregnancy, and neuromodifying medications.
Current density and total charge are the most important parameters for judging safety and must exceed 24 mA/cm2 before brain tissue is at risk of harm (McCreery et al., 1990). The current density in typical protocols is approximately 0.1 mA/cm2, well within these limits. Safety considerations have been built into the tDCS unit whereby the operator can programme a predefined upper voltage limit to shut down the unit if high resistance occurs. In this scenario, the operator will add more saline solution/remoisten the electrode pads and recommence the session.
The risk of epileptic seizure is often raised in the literature due to a reported episode in one of the earliest studies (Redfearn et al., 1964), but as tDCS does not cause seizure, nor reduce the seizure threshold in animals, this does not appear to be a valid risk for human subjects with no history of seizure (Nitsche et al., 2008).
Several isolated cases of adverse reactions have been reported; these include skin irritation and breakdown following consecutive applications of tDCS, headache, and fatigue. Yet it should be stated that the safety of prolonged periods of application in stroke patients is relatively unknown; for example, it may produce occult effects, particularly on cognitive function, which have not been anticipated or measured (Fitz and Reiner, 2015). A systematic review of 23 randomized trials, allocating 371 patients to active tDCS and 293 to control, reported dropouts or adverse events in 6 of these studies, with comparable proportions of events in the active and sham conditions, risk difference (RD) 0.01, 95% confidence interval (CI): –0.02 to 0.03 (Figure 26.1) (Elsner et al., 2016a).
Figure 26.1 Forest plot showing the effects of transcranial electrical direct stimulation vs control on the composite of dropouts, adverse events, and deaths at the end of the intervention period, in post-stroke patients.
Variation in Effects
The physiological effects of tDCS on the cortex in healthy subjects are generally uniform; however, there is wide variation in the size of the effect that cannot be explained purely by stimulation parameters (Kim et al., 2014). This inconsistent effect is likely to be the result of many variables that are known to influence corticomotor plasticity, as listed in Table 26.1, and an interaction between them. The relative contribution of each variable is impossible to determine at this stage as there is a lack of studies which systematically manipulate each factor while controlling for the other parameters (Saiote et al., 2013). Inter-individual variation is even greater in the stroke population due to changes in cortical anatomy, including gliosis with corresponding increases in cerebrospinal fluid, which has a conductance 4–10 times greater than brain tissue (Wagner et al., 2007b).
Table 26.1 Factors affecting response to tDCS
Evidence
Studies in stroke patients have tested tDCS as a potential therapy for several different outcomes of interest, using varied electrode montages, in individuals with diverse stroke characteristics. The M1 hand area has been the therapeutic target used most extensively, due to its superficial location on the cortex and importance for recovery of upper extremity function, but several studies report successfully stimulating the leg area of M1, the language centres, and cerebellum.
Effects of tDCS according to Outcome
Activities of Daily Living (ADL)
A systematic review in the Cochrane Library identified 10 randomized controlled trials (RCTs) with a total of 407 stroke patients assessing the effect of tDCS on ADL functional level, as measured on the Frenchay Activities Index, the Barthel Index, or similar scales (Elsner et al., 2016a). For the outcome of absolute level of ADL function at the end of the intervention period, in nine trials, allocation to tDCS (232 patients), compared with control (164 patients), was associated with higher ADL function at the end of the intervention period: standardized mean difference (SMD) 0.24 (05% CI: 0.0 –0.44; p = 0.02). Effects were consistent across trials, I2 = 0%. One small additional trial evaluating change in ADL, rather than absolute ADL, function found a qualitatively similar effect (Figure 26.2).
Figure 26.2 Forest plot showing the effects of transcranial electrical direct stimulation vs control on activities of daily living at the end of the intervention period, in post-stroke patients.
Six of these studies, with 269 participants, assessed the sustained effects of tDCS beyond the intervention period on ADL at end of study follow-up and found evidence of a sustained beneficial effect: SMD 0.31, 95% CI: 0.01–0.62; p = 0.04. However, the confidence interval was wide and there was evidence of moderate heterogeneity across trials (I2 = 27%) (Figure 26.3).
Figure 26.3 Forest plot showing the effects of transcranial electrical direct stimulation vs control on activities of daily living at the end of follow-up, in post-stroke patients.
Upper Limb Function
A systematic review in the Cochrane Library identified 16 RCTs enrolling 484 stroke patients assessing the effect of tDCS on upper limb function, as measured on the Action Research Arm Test, the Fugl-Meyer Score, or similar assessments (Elsner et al., 2016a). For the outcome of absolute amount of improvement in upper limb function scores, in 10 trials, allocation to tDCS (243 patients), compared with control (188 patients), was not associated with a statistically significant difference in arm and hand function at the end of the intervention period: SMD 0.11 (05% CI: –0.17–0.39). Four small, additional trials evaluating change in upper limb, rather than absolute upper limb, function found qualitatively similar effects (Figure 26.4).
Figure 26.4 Forest plot showing the effects of transcranial electrical direct stimulation vs control on upper limb function at the end of the intervention period, in post-stroke patients.
Lower Limb Function
Studies investigating the effects of tDCS on lower limb function are fewer, in part because the somatotopy of the legs is located deep in the central sulcus and technically difficult to isolate. However, there is consistent evidence that this area can be reached and is a feasible target for tDCS stimulation (Chang et al., 2015). Individual RCTs have provided signals of potential benefit from the use of anodal tDCS to improve postural stability (Sohn et al., 2013; Koyama et al., 2015), lower limb muscle strength (Tanaka et al., 2009; Tanaka et al., 2011), and gait function (Tahtis et al., 2014). Conversely, individual RCTs did not show strong signals of beneficial effects on gait parameters, balance control (Chang et al., 2015), and lower limb biomechanics (Tahtis et al., 2014). These isolated reports from small studies require further validation.
Spasticity
A systematic review identified three RCTs enrolling 206 patients evaluating tDCS compared with control for post-stroke spasticity, as assessed with the Modified Ashworth Scale (Elsner et al., 2016b). Overall, tDS did not significantly alter the absolute amount of improvement in spasticity scores: standardized mean difference –0.61, 95% CI: –1.51–0.30; p = 0.19.
Aphasia
A systematic review identified 12 RCTs assessing tDCS for post-stroke aphasia, among which 6 trials, enrolling 66 patients, could be pooled for the outcome of accuracy of picture naming. Allocation to tDCS, compared with control, was not associated with a statistically significant difference in correct picture naming at the end of the intervention period (SMD 0.37, 95% CI: –0.18–0.92; p = 0.19) (Elsner et al., 2015) (Figure 26.5). No studies were identified that used a standardized measure of functional communication, that is, an objective measure of real-life communication, rather than a more narrow endpoint like picture naming. Subsequent, modestly larger RCTs have continued to provide mixed positive (Meinzer et al., 2016) and neutral (Spielmann et al., 2018) signals.
Figure 26.5 Forest plot showing the effects of transcranial electrical direct stimulation vs control on accuracy of picture naming at the end of the intervention period, in post-stroke patients.
Hemispatial Neglect
A systematic review identified two studies, providing three randomized comparisons of 32 patients, assessing tDCS for hemispatial neglect after stroke, as measured by the Line Bisection Test. Allocation to tDCS, compared with control, was associated with a statistically significant greater reduction in hemispatial inattention: SMD –1.07, 95% CI: –1.76 to –0.37; p = 0.003 (Salazar et al., 2018). However, two of the comparisons used a shared control group, warranting interpretive caution.
Additional Cognitive Functions
Studies in healthy adults have suggested tDCS to be capable of improving additional cognitive domains, including attention, memory, and executive functions (Smith and Clithero, 2009). Small RCTs have explored effects of tDCS in elements of these domains in stroke patients, with mixed results (Kang et al., 2009; Yun et al., 2015; Kazuta et al., 2017; Shaker et al., 2018). Due to the prevalence of cognitive impairment post-stroke and its association with poor rehabilitation outcomes, further research in this field is warranted.
Mood
Transcranial direct current stimulation has induced mood improvements in several neuropsychiatric conditions but has been assessed in one modest-sized, controlled trial for post-stroke depression, enrolling 48 patients (Valiengo et al., 2017). Stimulation intensity was 2 mA for 30 minutes daily for 10–12 sessions, centred on left dorsolateral prefrontal cortex. On the primary endpoint, score on the Hamilton Depression Rating Scale, tDCS was associated with less depressed scores at the final 6-week visit (mean difference [MD] 4.7, 95% CI: 2.1–7.3; p < 0.001), but not at the 2-week or 4-week visit. This encouraging initial trial result supports the merit of further investigations.
Dysphagia
A systemic review identified four sham-controlled RCTs, enrolling 109 patients, assessing tDCS for post-stroke swallowing dysfunction (Chiang et al., 2018). Sites of stimulation were ipsilateral or contralateral swallowing or pharyngeal motor and sensory cortices. Allocation to tDCS, compared with sham, was associated with a greater improvement in swallowing scale scores: SMD 0.61 (95% CI: 0.14–1.08). There was substantial variation across trials in stimulation intensity (1 mA and 2 mA), duration of treatment (between 4 days and 10 days), and time from stroke onset to treatment start (between <7 days and >1 month), but the net positive signal supports further studies to confirm benefit and identify optimal treatment parameters.
Effects of tDCS according to Type and Hemisphere of Stimulation
Three broad variations in stimulation paradigm have been evaluated in tDCS studies: (1) anodal tDCS over the lesioned hemisphere; (2) cathodal tDCS over the lesioned hemisphere; and (3) bihemispheric stimulation with anodal tDCS over the lesioned and cathodal tDCS over the non-lesioned hemisphere. For outcomes of improvement in ADL and improvement in motor function, the pattern of trial results is suggestive, but not definitive, that cathodal-ipsilesional and bihemispheric stimulation paradigms are more effective than anodal-ipsilesional.
In the systematic review in the Cochrane Library of tDCS to improve ADL in post-stroke patients, study stimulations were: (1) anodal tDCS over the lesioned hemisphere (5 trials, 164 patients); (2) cathodal tDCS over the lesioned hemisphere (6 trials, 301 patients); and (3) bihemispheric stimulation with anodal tDCS over the lesioned and cathodal tDCS over the non-lesioned hemisphere (2 trials, 33 patients). There was no statistically significant evidence of heterogeneity in the overall positive treatment effect across the three stimulation paradigms (subgroup heterogeneity p = 0.16). However, within-subgroup point estimates were positive for cathodal-lesional (SMD 0.33) and bihemispheric (SMD 0.30) paradigms, compared with neutral for anodal (SMD -0.04) (Elsner et al., 2016a) (Figure 26.6). Similar findings were noted in a systematic review of tDCS for motor performance in stroke patients. Among the 19 RCTs identified, stimulation types studied were: anodal tDCS in 9 trials, cathodal tDCS in 7 trials, and bihemispheric tDCS in 3 trials. None of the stimulation paradigms reached a statistically significant within-subgroup treatment effect, but point estimates were positive for cathodal (SMD 0.39) and bihemispheric (SMD 0.24) paradigms, compared with neutral for anodal (SMD 0.05) (Marquez et al., 2015).
Figure 26.6 Forest plot showing the effects of transcranial electrical direct stimulation vs control on activities of daily living at the end of the intervention period, in post-stroke patients, according to the type and hemisphere of stimulation.
Effects of tDCS according to Stroke Characteristics
Time since Stroke
Preliminary data have provided conflicting signals regarding the effect of time since stroke upon responsiveness to tDCS, and modification may vary by the functional networks being stimulated. In the systematic review in the Cochrane Library of tDCS to improve ADL in post-stroke patients, stimulation time periods analysed were: (1) acute/subacute (first 1–4 weeks post-stroke: 4 trials, 213 patients); (2) late subacute (1–6 months post-stroke: 2 trials, 140 patients); and (3) chronic (>6 months post-stroke: 4 trials, 93 patients) (Elsner et al., 2016a). Although point estimates directionally favoured earlier treatment periods, there was no statistically significant evidence of variation in treatment effects across the timing subgroups (subgroup heterogeneity p = 0.59) (Figure 26.7). In a systematic review of tDCS for motor performance in stroke patients, among 11 RCTs with clear chronic stroke populations, stimulation time periods analysed were acute/subacute (3 trials) and chronic (8 trials) (Marquez et al., 2015). Confidence intervals for treatment effects overlapped across the two time intervals, suggesting subgroup differences did not reach statistical significance. However, point estimates directionally favoured the later treatment period, in which benefit reached statistical significance: SMD 0.45 (95% CI: 0.09–0.80) (Figure 26.8).
Figure 26.7 Forest plot showing the effects of transcranial electrical direct stimulation vs control on activities of daily living at the end of the intervention period, according to time since stroke until treatment period.
Figure 26.8 Forest plots showing the effects of transcranial electrical direct stimulation vs control on motor performance at the end of the intervention period, according to time since stroke until treatment period. (a) Chronic period after stroke. (b) Subacute period after stroke.
Stroke Severity
In the systematic review of tDCS for motor performance in stroke patients, among 13 RCTs with clear severity populations, effects were analysed in individuals with mild/moderate impairments (9 trials) and moderate/severe impairments (4 trials) (Marquez et al., 2015). Confidence intervals for treatment effects overlapped across the two time-deficit severity subgroups, suggesting subgroup differences did not reach statistical significance. However, point estimates directionally favoured the mild/moderate severity group, in which benefit reached statistical significance: SMD 0.37 (95% CI: 0.05–0.70) (see Figure 26.8).
Implications for Practice and Research
Currently, there is suggestive, but inconsistent and imprecise, evidence available indicating that tDCS may improve ADL performance, motor function, swallowing, mood, and language and other cognitive functions after stroke. There is insufficient evidence to clarify the optimal type, targeting, intensity, and duration of tDCS for different functional domains. Clearly, not all stroke patients respond in the same way or benefit from tDCS to the same magnitude, and future research needs to establish well-defined patient selection criteria. If tDCS does work by enhancing neuroplasticity and capacity for reorganization, it is likely that its effects will be maximized if given in tandem with concomitant intensified behavioural rehabilitation therapy in the functional domain targeted for improvement. This expectation, however, requires validation in clinical trials.
There are currently numerous ongoing registered, large-scale RCTs, and this further evidence is necessary to substantiate whether tDCS has merit as a therapeutic adjunct in stroke rehabilitation.
Transcranial Magnetic Stimulation
The Stimulator
Transcranial magnetic stimulation is delivered to the brain by passing a strong, brief electrical current through an insulated wire coil placed on the skull. Several coils are available, with the two most common being circular and figure-8, whereby the latter produces a more focused electric field peak under the centre of the coil (Peterchev et al., 2012). The subject’s head is partially immobilized in a padded head- or chinrest to prevent movement, and the coil is generally held in place by the researcher for the duration of the stimulation. In contrast to tDCS, TMS has high focality, and accurate positioning is required and is usually achieved with neuronavigational equipment (Cabrera et al., 2014).
Transcranial magnetic stimulation can be used in several different modes: single-pulse TMS is used to investigate the excitability of the corticospinal system, paired-pulse TMS can be used to assess connectivity within and between cortical regions, and long trains of stimuli can be used to induce excitability changes desired for stroke rehabilitation (rTMS) (Paulus et al., 2013).
Mechanism of Action
Repetitive transcranial magnetic stimulation acts as a neurostimulator. The electric current produced by the coil generates a transient magnetic field which passes into the brain, where it induces a secondary current in a parallel plane to the plane of the coil, which depolarizes neurones (Bolognini et al., 2009). The induced electric field causes the shift of ions in the brain without the need for current to flow across the skull; therefore, it avoids the skull impedance issues encountered with tDCS. When delivered over the primary motor cortex, it induces efferent volleys along the corticospinal tracts (Barker et al., 1985). Depending on the stimulation duration and frequency, output pulse shape, coil geometry, positioning, and strength of the magnetic field, rTMS can be used to either excite or inhibit areas of the cortex with great temporal precision (Priori et al., 2009).
Application of TMS
Conventional rTMS protocols use trains of evenly spaced pulses usually consisting of stimuli applied at either low frequency (1–2 Hz) to decrease cortical excitability, or high frequency rTMS (>1 Hz) to increase it. More recently, new rTMS paradigms have been developed in which the train duration and temporal spacing of the bursts are altered. Theta burst stimulation (TBS) uses repeated high-frequency (50 Hz) bursts of pulses applied at a theta frequency (5 Hz) that may produce more enduring changes in excitability at a lower dosage than conventional rTMS (Paulus et al., 2013). Another technique, known as paired associative stimulation (PAS), combines rTMS with peripheral nerve stimulation at fixed time intervals. Excitation or inhibition can be selectively produced depending on the interval between the stimulation (Wolters et al., 2003).
Safety
Protocol guidelines and safety parameters have been well defined for the use of TMS, and the safety of TMS continues to be supported by the literature (Machii et al., 2006). The only absolute contraindication to TMS is the presence of metal in close contact to the coil. Conditions of increased risk are related to the use of novel paradigms and patient characteristics such as a history of epilepsy, severe cardiac disease, and drugs including alcohol and neuromodifying agents.
The occurrence of seizure is the most serious TMS-related, acute adverse effect but reports of this are extremely rare, with occurrences typically reported when the procedure exceeds the guidelines, or when patients are receiving treatment with medications which may lower the seizure threshold (Rossi et al., 2009). Other safety concerns which have been raised include transient hearing changes (a broadband acoustic artefact is produced by the mechanical deformation of the stimulating coil), localized pain/discomfort, headache, and cognitive changes. A systematic review in the Cochrane Library reported that, of the included 327 stroke patients, adverse events were: transient or mild headache in 2.4%, syncope in 0.6%, anxiety in 0.3%, and insomnia exacerbation in 0.3% (Hao et al., 2013). These effects are considered to be mild and transient and do not typically affect patient compliance.
The potential risk of long-term magnetic field exposure for rTMS operators due to daily exposure over prolonged periods of time is an issue that may need to be addressed in the future (Rossi et al., 2009).
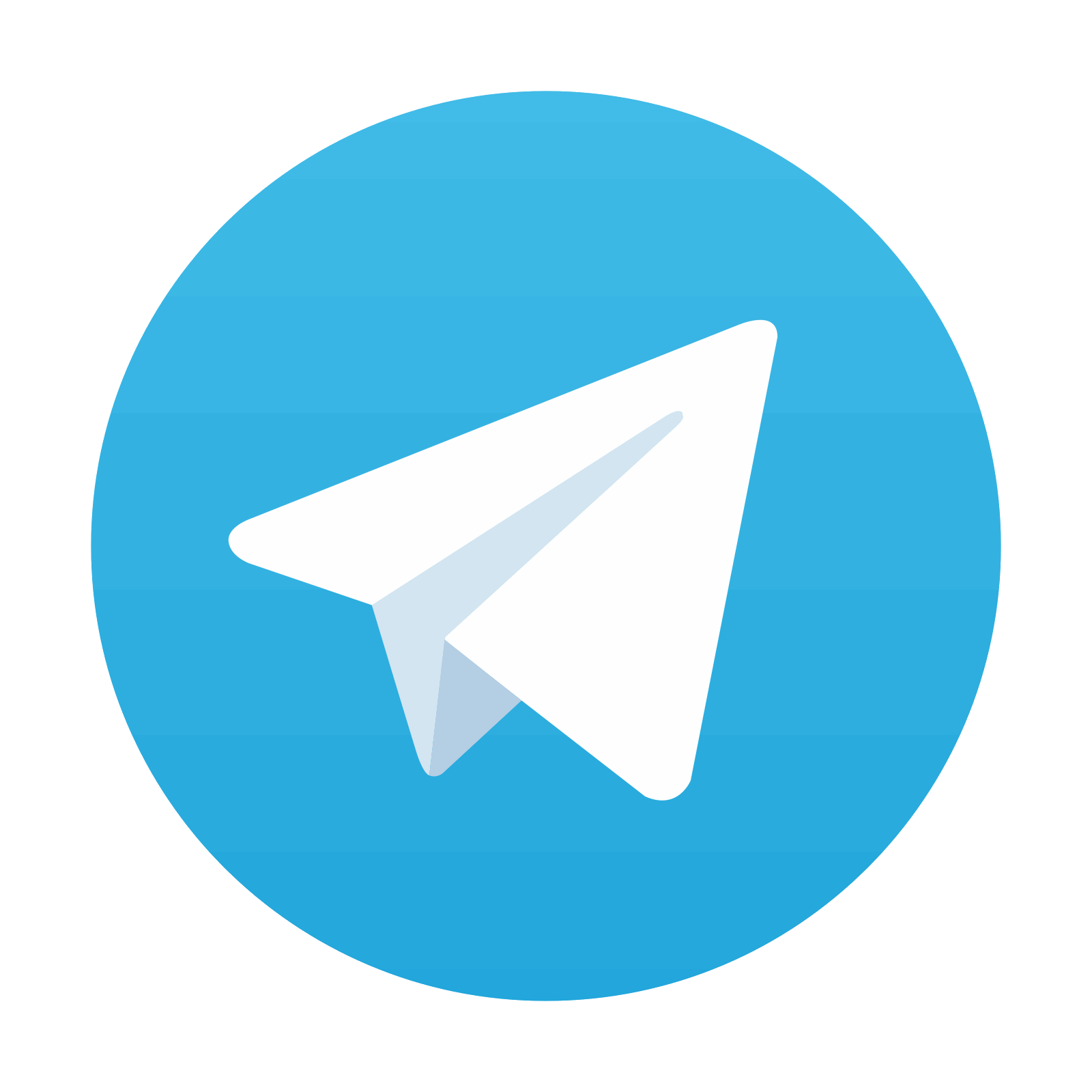
Stay updated, free articles. Join our Telegram channel

Full access? Get Clinical Tree
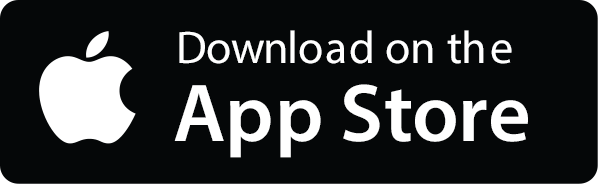
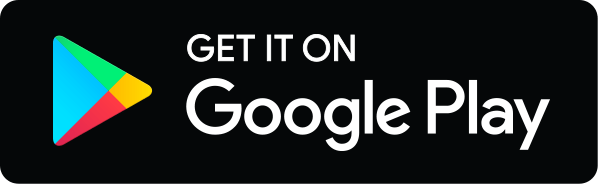
