Abstract
In recent years, there has been tremendous support for working toward the RDoC goals of identifying the neurobiological mechanisms that cut across or are common to multiple psychiatric disorders. Identifying the pathophysiological mechanisms underlying transdiagnostic symptoms will improve the validity of disease classifications by grouping individuals based on multiple dimensions of behavior and biology. This could potentially account for heterogeneity and comorbidity observed among DSM diagnostic categories.
5.1 Introduction
In recent years, there has been tremendous support for working toward the RDoC goals of identifying the neurobiological mechanisms that cut across or are common to multiple psychiatric disorders. Identifying the pathophysiological mechanisms underlying transdiagnostic symptoms will improve the validity of disease classifications by grouping individuals based on multiple dimensions of behavior and biology. This could potentially account for heterogeneity and comorbidity observed among DSM diagnostic categories. One of the constructs that provides an excellent opportunity for transdiagnostic research is reward processing, as reward-related dysfunction is reported across several psychiatric disorders including schizophrenia and addiction, but especially mood disorders. Current research suggests that abnormal reward sensitivity is involved across the entire mood disorder spectrum, with blunted reward-related functioning observed as a risk for major depression, whereas abnormally elevated reward-related functioning reported as a risk for bipolar disorders (BDs). However, the results are mixed and there is considerable debate regarding the degree to which shared or distinct profiles of reward system dysfunction contribute to the motivational deficits observed across unipolar and bipolar mood disorders. This is due to the fact that reward processing is not a unitary construct, but multifaceted, and comprises subcomponents with distinct and overlapping neurobiological underpinnings. For example, different components of reward processing are suggested to include reward anticipation, reward consumption, and reward learning. In addition, both unipolar depression and BDs are highly heterogeneous. Consequently, considerable research has focused on understanding if these discrete components of reward processing could explain the heterogeneity observed across mood disorders and lead to finer parcellation of symptom clusters. This will further lead to identification of homogeneous subgroups based on symptomatology, a prerequisite process to the development of targeted prevention and treatment strategies.
The primary focus of this chapter is to review the neural mechanisms underlying different reward processing components, including reward anticipation, reward consumption, and reward learning, in major depressive disorder (MDD) and BD. The chapter will also review evidence for these neural mechanisms acting as state-like versus trait-like markers of mood pathology. In addition, we will comment on future analytical approaches that are needed to understand the complex reward-related disruptions observed across mood disorders.
5.2 Neurobiology of Reward Processing and Its Components
The components of reward processing mentioned here – reward anticipation, reward consumption, and learning map onto the core reward components (reward responsiveness, reward valuation, and reward learning) of reward processing outlined in the Positive Valence Systems Matrix of the National Institute of Mental Health’s (NIMH’s) Research Domain Criteria (1–3). Reward responsiveness (or anticipation) is associated with the ability to anticipate or represent future incentives. In contrast, reward valuation refers to the processes by which the value of a reinforcer is computed as a function of its magnitude, predictability, time to expected delivery, and the effort required to obtain it. Finally, reward learning incorporates the process by which an organism acquires information about reward-predictive cues and by which novel reward outcomes subsequently shape behavior.
Evidence suggests that these processes map onto partially distinct neural circuitry (for a review, see 4). Briefly, the reward system has been linked to the frontal–striatal circuit that responds to stimuli involving the anticipation and receipt of rewards (e.g., 5). The ventral striatum is thought to play a central role in reward anticipation and is involved in processing both primary (e.g., food) and secondary (e.g., monetary) rewards. In contrast, studies examining reward consumption implicate “hedonic hotspots” in the ventral pallidum and nucleus accumbens that are the site of endogenous opioid receptors (6), as well as the encoding of reward value in the orbitofrontal cortex (OFC; 7). In the past decade there has been a burgeoning interest in applying computational algorithms to dissect reward learning in healthy and psychiatric populations. Using these models, individual differences can be estimated by measuring trial-by-trial variability in learning. Learning occurs when there is a deviation between the expected and actual outcome, quantified as a prediction error which is then used to update value estimates that support better prediction of future rewards. Nonhuman primate findings have shown that phasic firing of dopamine (DA) neurons in the ventral tegmental area (VTA) encodes reward prediction error (RPE). These midbrain DA RPE signals are then transmitted to the striatum and cortex and used to update stimulus-action values and guide goal-directed behavior (8, 9). Consistent with this, human fMRI studies have described RPE signals in cortico-striatal circuits including the striatum, midbrain, and prefrontal cortex (10, 11), and these signals have been found to be disrupted under conditions that affect phasic DA signaling (e.g., DA antagonists) (12–14).
5.3 Major Depressive Disorder
5.3.1 Neural Correlates of Reward Anticipation
Several fMRI studies have found that compared to psychiatrically healthy individuals, individuals with MDD show evidence of blunted striatal activation during anticipation of monetary rewards. For example, a recent meta-analysis of thirty-eight fMRI studies found that when both whole-brain effects as well as effects in targeted regions of interest were examined, individuals with MDD exhibited reduced activation in the ventral striatum during anticipation of reward (15). Furthermore, evidence suggests that this neural abnormality may be a trait-like marker of MDD that precedes depression onset. For example, reduced striatal activation during reward anticipation has been observed in adolescents who are at increased familial risk for MDD relative to their low-risk peers (16–18). Reduced anticipation-related ventral striatum activation also appeared to predict clinical course, with one study showing that blunted anticipation-related activation in this region predicted transition to subthreshold or clinical depression in healthy adolescents, and also predicted concurrent anhedonia in adolescents with depression at a two-year follow-up (19). In the context of treatment, changes in reward anticipation-related ventral striatum activation observed following treatment with a selective serotonin reuptake inhibitor (SSRI) were found to track changes in depressive symptom severity (20). Taken together, these findings indicate that disturbances in anticipation-related striatal function may underlie to the pathogenesis of MDD and may have important implications for the development of new interventions for the disorder.
In addition to disturbances in striatal function, several studies have found evidence for abnormal activation in cortical regions during reward anticipation in individuals with MDD, particularly in parts of the anterior cingulate cortex (ACC) and insula. For example, one study found evidence of increased activation in the ACC during anticipation of gains, along with reduced discrimination of gain versus non-gain outcomes, in unmedicated individuals with MDD (21). Additionally, relative to healthy controls, adolescents at increased familial risk for MDD exhibited both reduced and increased reward anticipation-related activation in the left and right insula, respectively (22). Furthermore, when comparing adolescents at increased familial risk for MDD who did and did not experience a depressive episode, Fischer and colleagues (16) found that although both groups showed evidence of blunted striatal activation during reward anticipation relative to adolescents who were not at familial risk for MDD, those who did not experience depression (i.e., those who appeared to be resilient despite having increased familial risk) showed greater activation in the middle frontal gyrus during reward anticipation relative to those who did go on to develop depression. These findings are again consistent with the hypothesis that attenuation of reward anticipation-related neural responses may be a trait-like marker of risk for depression. However, they also suggest that resilient individuals show changes in neural activation in cortical regions that may compensate for these deficits and thereby confer protective effects on mood and motivation.
5.3.2 Neural Correlates of Reward Consumption
In addition to disturbances in anticipation-related neural activation, MDD has been associated with abnormal patterns of neural activation during the receipt or “consumption” of rewards. For example, in the context of fMRI studies, the same meta-analysis that showed significantly reduced anticipation-related activation in the ventral striatum in MDD also found evidence for consistent reductions in striatal activation during reward feedback (15). Specifically, studies examining patterns of whole-brain activation during reward consumption found decreased activation in the left and right caudate in individuals with MDD relative to controls, whereas studies employing a region-of-interest approach found significantly reduced consumption-related activation in the caudate as well as the putamen and globus pallidus (15).
Evidence for disrupted consumption-related neural activation in MDD is not solely restricted to fMRI studies. For example, several event-related potential (ERP) studies have shown that individuals with MDD show a more blunted reward positivity (RewP) amplitude in response to reward feedback relative to healthy controls (for a review, see 23). The RewP is a fronto-central positive-going deflection in the scalp-recorded ERP that occurs approximately 250–350 ms following receipt of reward feedback (note that this component is often referred to as the feedback-related negativity [FRN] in cases where neural responses to reward and loss feedback are directly contrasted; 24). Although the precise origin of the RewP signal remains a topic of debate, it is thought to reflect the indirect effect of reward-related striatal activation on other regions, particularly the ACC (25). A meta-analysis of twelve ERP studies found that individuals with MDD had significantly more blunted RewP amplitudes in response to reward feedback relative to healthy controls, with the magnitude of this effect being largest for studies using adolescent MDD samples (15). Several studies have also highlighted links between blunted RewP amplitudes and increased risk for MDD. For example, a blunted RewP/FRN amplitude has been found to predict the onset of a first depressive episode longitudinally (26), and has been found to moderate the association between maternal depression and future depressive symptoms in offspring (27). Blunted RewP amplitudes have also been observed in individuals who are in remission from a depressive episode (28, 29). Taken together, findings from the fMRI and ERP literature suggest that disruption in reward consumption-related neural activation may also be a trait-like risk marker for MDD.
5.3.3 Neural Correlates of Reward Learning
In addition to anticipation and consumption, another domain of reward processing that is reported to be impaired in MDD is reward learning. Prior fMRI studies have highlighted blunted RPE signals in the striatum (30–33) but intact RPE signal in the VTA (which are the hypothesized source of striatal RPE signals) during learning in individuals with MDD. This suggests that, in MDD, RPE signals might be accurately encoded in the VTA but are not appropriately transmitted to the striatum potentially due to abnormal connectivity between these two regions, thereby causing reduced downstream RPE signaling and impaired reward learning. Supporting this interpretation, a recent study observed intact VTA RPE signals but reduced functional connectivity between the VTA and striatum during reward feedback in individuals with MDD. (33) This weakened VTA–striatal connectivity in the MDD group points to a downstream DA signaling deficit (as observed by reduced RPE signals in the striatum), which then leads to impaired reward learning (34).
Another study reported that the striatal RPE signals correlated with self-reported depression severity suggesting that higher depression severity scores exhibit lower reward learning. Further, supporting the importance of these learning signals in a healthy sample, individuals with a higher genetic risk exhibited higher stress-induced reduction in RPE in the striatum. These studies together suggest these RPE signals may be a trait-marker of MDD, but future studies investigating learning in at-risk individuals are warranted.
5.4 Is Reward Dysfunction a Potential Endophenotype of MDD?
According to Gottesman and Gould (35), endophenotypes (or intermediate phenotypes) are measurable components (e.g., neurophysiological, biochemical, neuroanatomical, cognitive, or neuropsychological) that exist between the behavioral symptoms of a disease and genes. To be considered an endophenotype, a reward dysfunction should meet the following criteria (35): (a) specificity (i.e., reward dysfunction is strongly associated to a given condition); (b) heritability; (c) state independent (i.e., reward dysfunction is stable over time and independent from clinical state and treatment); (d) cosegregation (i.e., reward dysfunction occurs more frequently in affected compared to non-affected relatives of an ill individual); (e) familial association (i.e., reward dysfunction is more frequent in relatives of ill individuals than the general population); and (f) biological and clinical plausibility (36). Because endophenotypes are presumed to be more proximal to genes than clinical diagnoses, they may help us identify genetic variants and associated genes with small samples. Identification of genetic variants and associated genes using small samples. Endophenotypes are thought to be to be disorder-specific. Blunted striatal response to both anticipation and consumption has been suggested as an endophenotype of MDD, as blunted striatal response to rewards has been reported in individuals with current depression, remitted MDD, and non-affected family members of individuals with MDD. Next, endophenotypes must be heritable and although no studies have investigated the heritability of striatal reward responses, behavioral response to rewards and reward-related ERPs is reported to be heritable. In addition, blunted striatal response to reward response has been observed within never-depressed offspring of depressed versus healthy parents (37, 38). This blunted striatal response to both anticipation (39) and consumption (40, 41) prospectively predicted worsening of depressive symptoms over two years among adolescents, even after controlling for baseline symptoms. Furthermore, reduced FRN amplitude – a deflection in the ERP thought to originate from RPE activity in the dorsal ACC and striatum, predicted first onset of MDD in a two-year follow-up among never-depressed adolescent girls (26). Finally, the reliability of striatal response to rewards has been poor to moderate (17). Nevertheless, taken together, these studies suggest that neural markers of altered reward processing are a promising endophenotype for MDD risk. However, future studies will need to replicate these findings over a broad developmental range and to investigate the specificity and reliability of such findings.
5.5 Bipolar Disorder
5.5.1 Neural Correlates of Reward Anticipation
One prominent theory of BD proposes that the hyper-hedonic symptoms observed in (hypo)mania (e.g., sensation-seeking, spending sprees, hypersexuality) may arise due to hypersensitivity in the fronto-striatal circuits that support reward processing (e.g., see 42). A number of studies have yielded findings consistent with this theory. For example, Nusslock and colleagues (43) used a card-guessing paradigm to examine reward-related neural activation in euthymic BD versus healthy control participants. They found that relative to controls, the BD group displayed greater ventral striatal, medial OFC, and left lateral OFC activation during anticipation, but not during receipt, of monetary reward. This finding appeared to be specific to reward, as no group differences in neural activation were observed in response to anticipation or receipt of monetary loss. Increased left lateral OFC (particularly left ventrolateral prefrontal cortex; vlPFC) activation during reward anticipation appears to be one of the most consistent findings in fMRI studies of BD. Increased anticipation-related left lateral OFC/vlPFC activation has been observed across all mood states, including mania (44), depression (45), and euthymia (43), and has been observed in samples with BD type I (43–45) and BD type II (46). Similar findings have also been observed in unaffected first-degree relatives of individuals with BD (47). These findings suggest that abnormal left lateral OFC activation during reward anticipation may be a risk marker for BD.
However, not all studies have yielded findings consistent with the reward hypersensitivity theory of BD (hypo)mania. For example, using the monetary incentive delay task, Schreiter and colleagues (48) found that euthymic individuals with BD had decreased, rather than increased, activation in the bilateral ventral striatum during reward anticipation relative to controls. Other studies have found evidence for decreased striatal activation during reward anticipation in samples that include individuals with BD not otherwise specified (49). Furthermore, although Caseras and colleagues (46) found greater anticipation-related activation in the ventral striatum in individuals with BD type II relative to controls, no abnormalities in anticipation-related striatal activation were observed for individuals with BD type I, who are at risk for more severe (hypo)manic symptoms than are individuals with BD type II. These findings have raised the question of whether BD can be characterized by increased or decreased activity in reward-related regions. In an attempt to reconcile these conflicting findings, Mason and colleagues (50) proposed a model wherein mood strongly biases reward perception in individuals with BD, such that patients might exhibit hypersensitivity to rewards when mood is high and hyposensitivity to rewards when mood is low. Future studies examining the association between changes in mood state and changes in neural responses to reward in individuals with BD are needed to fully test this hypothesis.
5.5.2 Neural Correlates of Reward Consumption
Consistent with the reward sensitivity hypothesis of BD, several studies have reported increased activation in the ventral striatum and medial OFC in response to reward outcome in individuals with subthreshold hypomania or euthymic BD (51, 52). These abnormal patterns of neural activation have been observed in response to a range of reward types. For example, using monetary and social incentive delay tasks, euthymic individuals with BD type I have been found to show increased striatal activation during receipt of both monetary and social rewards, suggesting that hypersensitivity to reward consumption may be evident across a variety of contexts in individuals with BD (53). Several ERP studies examining RewP/FRN amplitudes in response to reward feedback have also found abnormalities in reward consumption-related neural activation in individuals at risk for BD. For example, individuals with elevated scores on the hypomanic personality scale were found to show elevated FRN amplitudes in response to reward feedback relative to individuals with moderate or low scores on this measure (54). Similar findings have also been observed in individuals meeting full criteria for BD (55).
Although many of these findings appear to align with the reward hypersensitivity model of BD, not all studies have yielded consistent findings. For example, one study found that individuals with BD mania had reduced, rather than increased, striatal activation during receipt of monetary rewards relative to controls (56). Furthermore, another study found that reduced ventral striatal activation during receipt of social rewards correlated with the severity of depressive symptoms in depressed individuals with BD, despite there being no differences in overall neural activation between the BD group and controls (57). Compared to the growing literature on the neural correlates of reward processing in MDD, the relative paucity of studies in BD samples makes it difficult to determine the precise role that abnormal reward circuit activation plays in BD. Future studies are needed to determine the direction and extent of reward processing abnormalities that are evident across different BD subtypes and across different BD mood states.
5.6 Heritability of Reward Dysfunction in BD
BD is a highly heritable disorder, with an estimated heritability of 60–80% (47). The offspring of patients are reported to have a tenfold risk of developing BD relative to the general population. Therefore, identifying endophenotypes (or intermediate phenotypes) based on biological constructs that are associated with BD and reported in individuals at risk of BD could elucidate the pathophysiology of BD. Consistent with this, abnormalities in reward-related neural responses have been reported in healthy offspring of parents with BD. For example, similar to BD patients, healthy offspring of parents with BD also had elevated vlPFC activation during reward consumption (47, 58). Similarly, they also show elevated vlPFC–striatal functional connectivity during reward processing that was specific to offspring of parents with BD and not observed in healthy controls or offspring of parents with non-BD psychopathology (59), underscoring the specificity of these findings to BD and a potential “intermediate phenotype” for risk of BD.
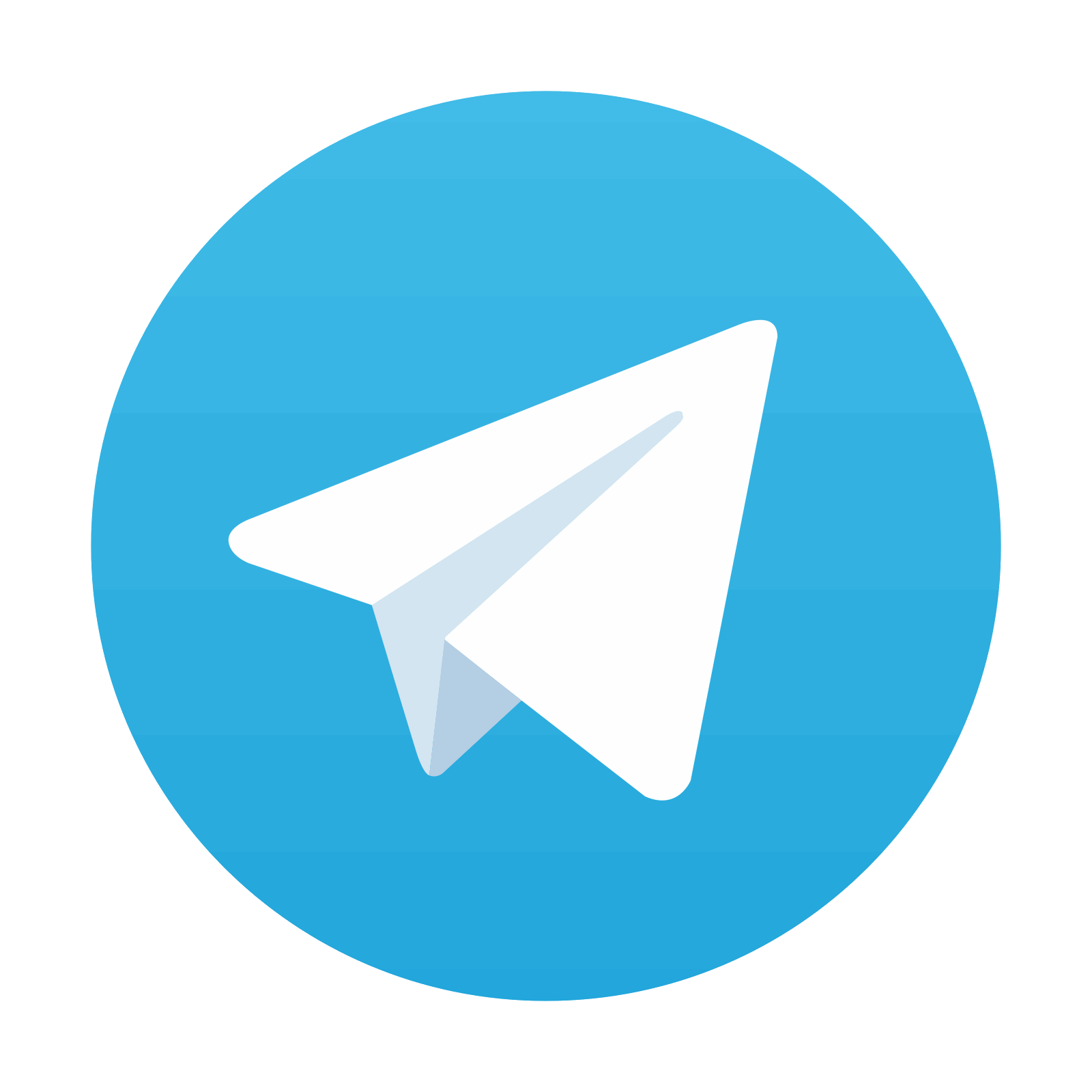
Stay updated, free articles. Join our Telegram channel

Full access? Get Clinical Tree
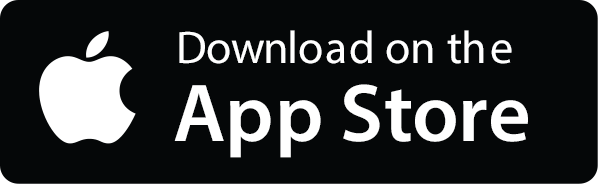
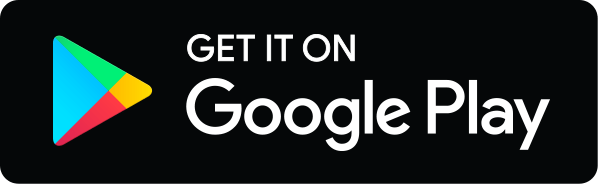