Abstract
The incidence of traumatic brain injury (TBI) in children is estimated to be high, being an important cause of mortality and morbidity. Post traumatic seizures (PTS), post-traumatic epilepsy (PTE), and neuropsychiatric sequelae after TBI contribute to the medical health burden in children. PTE has been recognized as one of the most common forms of acquired epilepsies in young people, and it is associated with poor functional and psychosocial outcome. In this chapter, clinical presentation, diagnosis and management of PTE, and the role of antiepileptic drugs in children are discussed. In addition, the poorly understood pathophysiology of PTE is discussed with reference to genetic and neurophysiologic biomarkers and potential risk factors that increase the risk of developing PTE.
Introduction
In 2014, about 2.87 million traumatic brain injury (TBI)-related emergency department (ED) visits, hospitalizations and deaths occurred in the United States. This number has increased by 53% from 2006 (approximately 1.88 million) to 2014. It has been estimated that in 2014, over 837,000 TBI-related ED visits, hospitalizations and deaths occurred among children.1
In addition to being an important cause of death, TBI is a common source of morbidity in children. Post-traumatic seizures (PTS), post-traumatic epilepsy (PTE) and neuropsychiatric sequelae contribute to the medical health burden that children frequently have after TBI. PTS are commonly defined by many researchers as immediate seizures (occurring less than 24 hours after TBI), early PTS (occur less than 1 week after injury) and late PTS (occur more than 1 week after TBI). PTE is usually defined as the patient having had two or more unprovoked seizures (some authors require one unprovoked seizure) following TBI.2, 3 This chapter will be devoted to the discussion of PTE.
Epidemiology and Risk Factors
The incidence and prevalence of PTE in children is difficult to ascertain due to differences in definitions used, severity of injury and follow-up duration. Different studies show that 10–20% of children have PTE following severe TBI.4–9 The prevalence of PTE in children ranges from 0.2% to 11%.2, 7, 8, 10, 11 Distinct risk factors have been demonstrated to be associated with higher incidence of PTS or PTE. These risk factors are detailed in Table 5.1. Risk factors for developing PTE are: early PTS, higher severity of TBI (i.e., worse Glasgow Coma Scale (GCS)), young age, abnormal neuroimaging (subdural hematomas, uncal herniation, brain contusion, skull fractures) and/or prolonged loss of consciousness. Note, however, that some of the population studies included both children and adults without distinction between these two groups. Additionally, younger age, nonaccidental head trauma and presence of intra-axial blood were clinical predictors of subclinical seizures or status epilepticus in a prospective study that included patients who were admitted to the pediatric intensive care unit (PICU).5 Furthermore, it is important to mention that PTE may develop 10 years after a severe TBI.10, 11
Table 5.1 Relationship of Risk Factors and the Development of PTS or PTE in Subjects with TBIa
Study (design) | No. of subjects (age) | Incidence rate | Risk factors for developing immediate or early PTS | Risk factors for developing PTE |
---|---|---|---|---|
Pediatric cohort | ||||
Lewis et al. (1993), USA (retrospective hospital chart review)35 | 194 children (3 months–15 years) | Immediate PTS: 7% (14/194) | GCS on admission ≤ 8. | NA |
Hahn et al. (1988), USA (retrospective hospital chart review and prospective follow-up between 7 months and 6 years)8 | 937 children (birth–16 years) | Immediate PTS: 9% (87/937); Early PTS: 0.3% (3/937); PTE: 0.2% (2/937) | GCS on admission ≤ 8; Diffuse cerebral edema; Acute subdural hematoma. | |
Appleton and Demellweek (2002), U.K. (retrospective hospital chart review and prospective follow up of children admitted in a rehabilitation unit)9 | 102 children (1.3–15.2 years) | PTE: 9% (9/102) | NA | Early PTS; GCS on admission < 8 (marginal significance). |
Emanuelson and Uvebrant (2009), Sweden (Population-based retrospective follow-up of 10 years)7 | 109 children with TBI (0–17 years) | Immediate PTS: 7% (8/109); PTE: 11% (12/109). | NA | Immediate PTS. |
Arango et al. (2012), USA (retrospective hospital chart review)6 | 130 children with severe TBI (0–17 years) | Early PTS (clinical or subclinical): 19% (25/130); PTE: 17% (22/130) | Young age (<1 year); Nonaccidental trauma; Epidural hematomas. | Presence of immediate or early PTS; Young age (<3 years); Subdural hematomas and uncal herniation. |
Arndt et al. (2013), USA (Prospective in-hospital, cEEG in PICU)5 | 87 children with TBI (1 month–18 years) | Immediate or early PTS (clinical and subclinical): 44% (37/87); Status epilepticus: 18% (16/87) | Young age (< 1 year); Nonaccidental trauma; Presence of fracture on computerized tomography. | NA |
Park and Chugani (2015), USA (retrospective hospital chart review)4 | 321 children with severe TBI (0–15 years) | PTE: 15% (47/321) | NA | Severe TBI. |
O’Neill et al. (2015), USA (Prospective in-hospital, cEEG)32 | 144 children with TBI (0–17 years) | Immediate or early PTS (clinical and subclinical): 30% (43/144) Status epilepticus: 16% | Young age (< 2.4 years); Nonaccidental trauma. | NA |
Adult and pediatric cohort | ||||
Annegers et al. (1998 and 2000), USA (retrospective hospital chart review)2 | 4541 children and adults (age not specified) | Immediate and early PTS: 2.6% (117/4541); PTE: 2.1% (97/4541) | NA | Brain contusion; Subdural hematoma; Severe head injuries; Skull fractures; Prolonged loss of consciousness; Early PTS. |
Aiskainen et al. (1999),46 Finland (patients referred to a rehabilitation and employment program after TBI, retrospective review hospital chart and prospective follow-up ≥ 5 years) | 490 children and adult (0.8–71 years): 241 subjects ≤ 16 years of age | Subjects ≤ 16 years of age: Early PTS: 24% (59/241); PTE: 30% (74/241) | Young age (< 7 years) | Early PTS; Depressed skull fracture; Young age. |
Thapa et al. (2010), India (Prospective observation hospital study)41 | 520 adults and children (14 days–89 years): 93 subjects ≤10 years of age | Subjects ≤10 years of age: Immediate PTS: 11% (10/93) Early PTS: 4% (4/93) PTE: 3% (3/93) | Both children and adult:: TBI due to fall from height Associated medical problems (hypertension, diabetes mellitus, chronic airway disorders, liver diseases, cerebrovascular disease and renal failure at the time of injury); Severity of TBI. | |
Zhao et al. (2012),47 China (retrospective hospital follow-up) | 2826 adults and children (4–79 years of age): 288 subjects ≤ 19 years of age | Subjects ≤ 19 years of age: PTE: 4% (12/288) | NA | Both children and adult: Older patients (particularly > 50 years of age); Severity of TBI; Higher number of surgery interventions; Contusions; Intraparenchymal brain hemorrhages; Early PTS. |
Wang et al. (2013),48 China (retrospective hospital chart review) | 3093 adults and children (1 month–95 years old): 320 subjects ≤14 years of age | Subjects ≤14 years of age: PTS 11% (34/320) (not specified timing of PTS) | Both children and adult: Frontal-temporal lobar contusion; Linear fracture; Severity of TBI. |
a It was not possible to specify the risk factors for immediate/early PTS vs. PTE for some studies because the authors did not make that distinction.
cEEG, continuous electroencephalography; GCS, Glasgow Coma Scale; NA, Not available; PICU, Pediatric intensive care unit; PTE, post-traumatic epilepsy; PTS, post-traumatic seizures; TBI, Traumatic brain injury
Pathophysiology
The pathophysiology of a TBI is poorly understood and it seems to differ significantly between a pediatric and an adult patient. It is well known that the immature brain has a higher capacity for plasticity, essential in synaptic formation. This can be an advantage as an injury in the developing brain may activate undefined mechanisms responsible for architectural and functional modifications of neuronal networks, maximizing the functional outcome.12 However, plasticity in a maturing brain can also be a source of vulnerability due to early imbalances in excitatory/inhibitory transmission. The proposed mechanisms to support this finding are postsynaptic decrease in GABAA receptor sensitivity and/or expression, changes in the ratio between postsynaptic expression of GABAA/GABAB receptors and decrease in presynaptic inhibitory interneuron activity.12 Additionally, mechanisms involved in epileptogenesis may include alterations in GABA-receptor-mediated postsynaptic inhibition, with the post-TBI-induced seizures occurring in part due to the reduction of GABAA-mediated inhibition.12 Other possible contributing factors associated with age-dependent differences include: lower expression of the glutamate type 1 receptor (with a slower clearance from the synaptic cleft) and N-methyl-d-aspartate (NMDA) receptors that are more permeable to Ca2+ with slower desensitization, thereby increasing excitability in the developing brain.13
Neuroinflammation may also play a role in the development of PTE. After a TBI, there is a cerebral inflammatory response which includes blood–brain barrier (BBB) dysfunction, edema, microglial, astrocytic activation and migration, and the release of inflammatory factors. The inflammatory factors include cytokines (including tumor necrosis factor (TNF-α), transforming growth factor-β (TGF-β) and interleukin-1β (IL-1β), -6 and -10, with downstream activation of intracellular signaling cascades involving nuclear factor Kappa light-chain-enhancer of activated B cells (NF-κB) and the resultant recruitment of blood-derived leukocytes into brain parenchyma. Although important in promoting tissue repair, microglia and astrocytes promote inflammation with production of cytotoxic factors and cytokines. All of the above contribute to edema, oxidative stress, the production of more cytokines and neurotoxic proteases, propagating the inflammatory cascade.13
Additionally, increasing evidence suggests that neuroinflammation (with activation of microglia and production of proinflammatory cytokines) may be a common consequence of epileptic seizure activity.14 Analysis of cerebrospinal fluid (CSF) from newly diagnosed adult patients with tonic-clonic seizures detected an upregulation of IL-6 and IL-1 receptors (IL-1Rs). Matched serum samples revealed higher levels of IL-6 in the CSF, suggesting that these cytokines likely originated in the brain. High levels of cytokines including IL-1β and high-mobility group box protein-1 (HMGB1) have also been identified in neurons and glia of surgically resected epileptic tissue.15–17 Conversely, the demonstration that glial cell activation and recruitment, and the synthesis of inflammatory factors may precede and/or occur concurrently with epileptogenic events support the hypothesis that neuroinflammation may also contribute to epileptogenicity after TBI. Interactions between neurons, glia and inflammatory mediators (HMGB1 interacting with toll-like receptors, IL-1β interacting with its receptors and TGF-β signaling from extravascular albumin in an immature brain) have all been implicated in promoting seizure susceptibility.13
Lastly, age-dependent differences in seizure susceptibility and neuroinflammation may contribute to a heightened vulnerability to epileptogenesis in young patients with TBI in comparison to adult patients. There is a higher upregulation of chemokines, higher levels of cytokines (IL-1α, IL-6, IL-12 and TNF-α) in CSF and serum, and differences in the activation of microglia, macrophages and neutrophil infiltration.13 Although these findings have been seen in multiple studies with different models, the mechanisms underlying the relationship between the inflammatory environment, age-dependent differences and epileptogenesis, particularly in the context of brain injury, remain poorly understood.
Furthermore, different biomarkers seem to be associated with PTE. Genetic association studies have suggested various predisposing genetic traits contributing to the development of PTE: single nucleotide polymorphism (SNP) rs1143634 of interleukin-1β (IL-1β) gene, SNPs rs3791878 and rs769391 of the glutamic acid decarboxylase 1 (GAD1) gene, SNPs rs3766553 and rs10920573 of the adenosine A1 receptor (A1AR) gene and the functional variant C677T of the methylenetetrahydrofolate reductase (MTHFR) enzyme.18 The most noteworthy biomarkers identified were IL-1β rs1143634 and A1AR rs10920573. Both had heterogenous at-risk genotypes (Cytosine-Thymine (CT)). Those with IL-1β rs1143634 CT genotype developed PTE in 47.7% of cases (p = 0.008) and this CT genotype was also associated with lower IL-1β serum levels and a higher CSF/serum IL-1β ratio. The IL-1β rs1143634 TT genotype was suggested to be protective.19 Those with A1AR rs10920573 CT genotype developed PTE in 19.2% of cases (p = 0.022).20 However, limitations of these genetic association studies included small sample sizes and some heterogeneity regarding the definition of PTS versus PTE.
Additionally, brain-derived peripheral biomarkers have been studied after TBI, particularly the glial protein S100B. TBI is associated with a rapid loss of BBB integrity followed (or in the absence) by brain injury. The S100B is a candidate peripheral biomarker of BBB permeability, reflecting the presence of BBB disruption and may have a role in predicting or ruling out brain injury. Due to the fact that serious intracranial events are associated with an increased risk for seizures, S100B may also prove useful in the future in detecting individuals at low risk versus those who will likely develop posttraumatic sequelae. Nonetheless, the correct interpretation of blood biomarkers related to the central nervous system (CNS) can be challenging, as most of these biomarkers are expressed to varying degrees outside the CNS.21, 22
Finally, neurophysiologic biomarkers can also be considered. Angeleri and collaborators followed prospectively 137 patients aged between 15 and 65 years for 12 months after TBI. They demonstrated that the presence of focal electroencephalography (EEG) abnormalities (focal slow and/or epileptiform activity) detected at 1 month after TBI was associated with a 3.49 fold increased risk of PTE 95% confidence interval, CI: 1.10–11.05, adjusting for low GCS scores, early seizures and single focal lesions on brain computerized tomography.23 A more recent study by Kim and collaborators performed a retrospective case–control study of risk factors for PTE, in which 25 consecutive patients who developed epilepsy within 1 year of a TBI were matched by injury severity and age to 25 controls who did not develop epilepsy within 1 year of the trauma, for patients aged ≥ 18 years. Epileptiform abnormalities in EEG (i.e., seizures, sporadic epileptiform discharges, lateralized or generalized periodic discharges and lateralized rhythmic delta activity) were associated with PTE (odds ratio, OR: 3.5 95% CI: 0.99–11.68, p=0.042). When evaluating individual subtypes of EEG epileptiform abnormalities, only sporadic epileptiform discharges were associated with PTE (OR: 4.57 95% CI: 1.60–21, p=0.007). These findings remained significant even after controlling for subdural hematoma (another independent factor that was shown to contribute to increased risk for PTE in this study). Differences in sporadic epileptiform discharges between cases and controls were already apparent within 5 days of the head trauma. The authors suggested that early EEG could be a useful diagnostic tool to assess TBI patients for PTE risk. Interestingly, EEG focal polymorphic slowing was also found to be associated with PTE on univariate analysis (OR: 2.67 95% CI: 0.97–10.1, p=0.04).24
Clinical Presentation
TBI in the pediatric population is associated with a high mortality rate and long-term morbidities, including motor, cognitive, behavioral and language impairments. As previously mentioned, another important consequence is the development of PTS and PTE.
Although the initial seizure type may be difficult to lateralize (due to TBI severity involving multifocal regions in the brain), some studies in pediatric patients have shown that focal seizure activity is the most common seizure type followed by generalized tonic-clonic activity.25 Infants can have focal seizures presenting with generalized seizure semiology, as in symmetric/asymmetric epileptic spasms or generalized tonic seizures.25 In a retrospective study of pediatric patients diagnosed with PTE, about 19% of patients (6 out of 31) had epileptic spasms.4 Furthermore, a higher severity of TBI is associated with multiple seizure types.4, 26 PTE can occur as late as 10 years after TBI. However, in the majority of patients (75%), the onset is within the first year after TBI.7, 10
After a TBI, there is an increased risk of psychiatric diagnosis, including depression, anxiety, posttraumatic stress disorder, obsessive-compulsive disorder, panic disorder and attention deficit hyperactivity disorder. Various episodic nonepileptic phenomena, mainly of psychopathologic nature or misinterpreted behavior, are characterized by paroxysmal movements, unusual sensations and staring. The presence of these nonepileptic events in a patient make the diagnosis of seizures more challenging.27
Assessment and Diagnosis
Head computerized tomography is the recommended neuroimaging in the acute setting after a severe TBI. Various abnormalities can be found, including fracture, hemorrhage, contusion, pneumocephalus, edema, herniation and compressed basal cisterns.28, 29 In the management of pediatric severe TBI, routinely obtaining a repeat computerized tomography scan >24 hours after the admission and initial follow-up are not suggested for making decisions about neurosurgical intervention, unless there is either evidence of neurologic deterioration or increasing ICP.30
Brain magnetic resonance imaging (MRI) is the study of choice, particularly for nonacute evaluations of PTE, providing the highest sensitivity to detect structural brain changes. Fluid attenuated inversion recovery (FLAIR) is helpful in detecting contusion, diffuse axonal injury, blood, edema or encephalomalacia. Other sequences are also helpful in detecting microhemorrhages, such as gradient echo (GRE) or susceptibility-weighted imaging (SWI). However, it is important to mention that some features can be transiently found in FLAIR or diffusion-weighted imaging (DWI) sequences after repeated and prolonged seizures.31
The utility of continuous electroencephalography (cEEG) has been studied in pediatric patients admitted to the hospital due to an acute mild to severe TBI. These studies showed that 7% to 16% of patients with TBI had only subclinical seizures and status epilepticus was identified in 11% to 18%. The incidence of status epilepticus in mild TBI was 12%, moderate TBI was 11% and severe TBI was 31%. Between 30% and 40% of patients had clinical or subclinical seizures.5, 32 Although there is no clear evidence that the routine use of cEEG can change the outcome, some potential benefits can be described including assistance in establishing the correct differential diagnosis and adequate treatment, avoidance of treatment of nonepileptic events and eventually some assistance with prognosis.33 These advantages propose a role for EEG monitoring to detect seizures in children with TBI in the acute setting, particularly in severe TBI.32, 34 In a patient presenting with a possible first epileptic seizure months or years after the TBI, a 10-20 system EEG can be helpful in the differential diagnosis. An interictal EEG showing epileptiform discharges has a specificity of >97% for epilepsy, despite a low sensitivity of 30–50%. A video-EEG monitoring also has a role in clarifying differential diagnosis and localizing the suspected epileptogenic zone.31
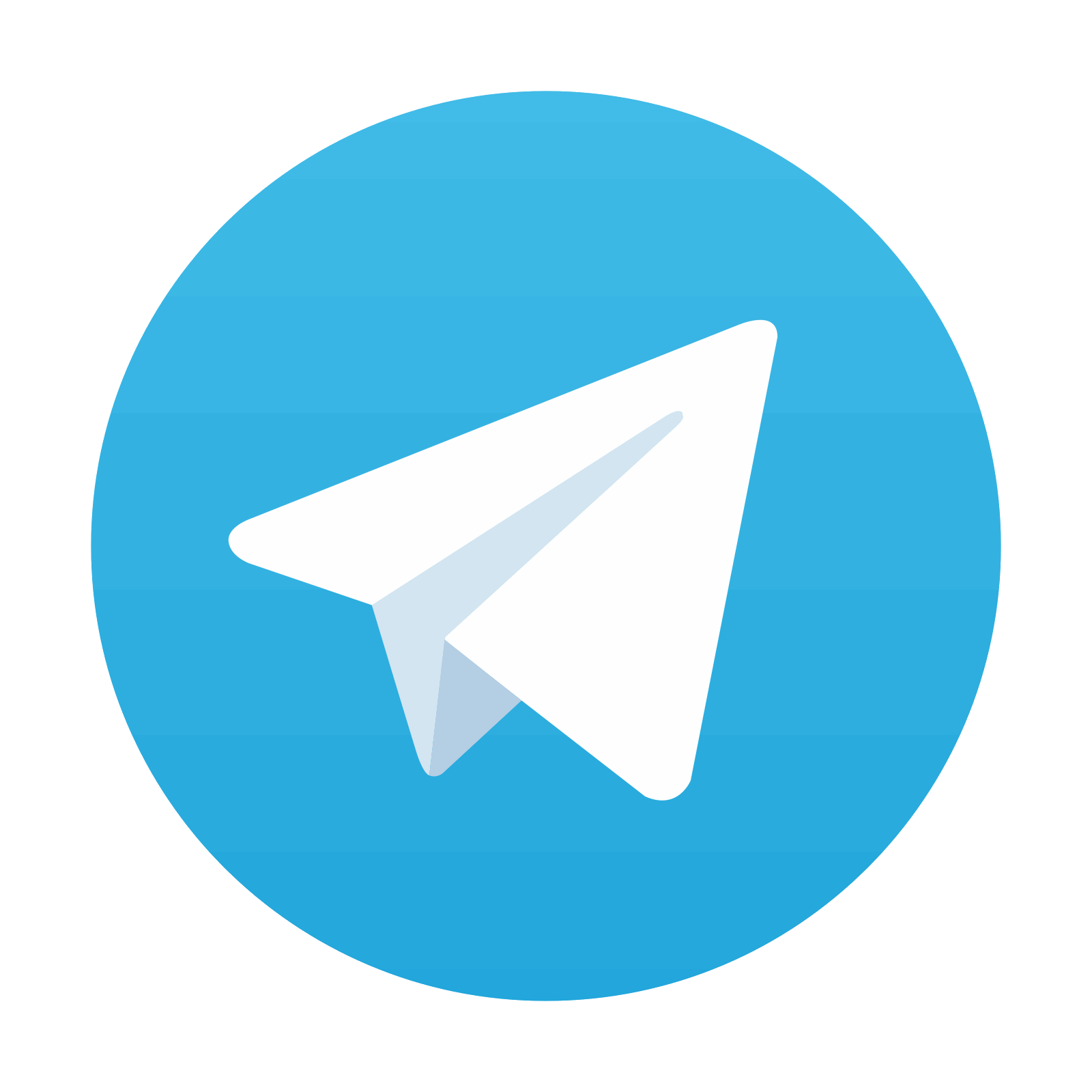
Stay updated, free articles. Join our Telegram channel

Full access? Get Clinical Tree
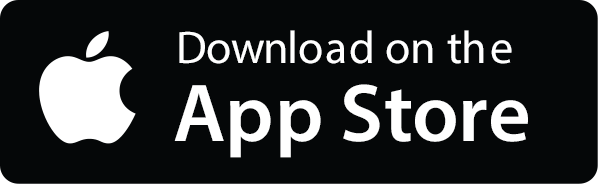
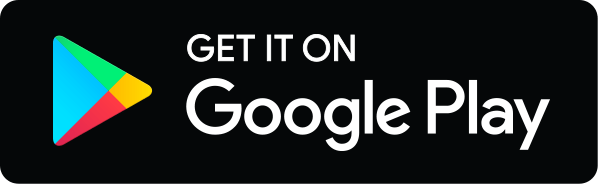