Fig. 14.1
Diagram of a cross-section of the human umbilical cord showing anatomical compartments including Wharton’s jelly, a rich source of stem cells
There are different compartments within the UC that are considered as hosts for stem cell populations. These compartments are described as distinct regions (Jeschke et al. 2011) and include the amniotic compartment (outer epithelial layer and inner subamniotic mesenchymal layer), the WJ compartment, the perivascular compartment surrounding the vessels, the media and adventitia compartment of the walls of UC blood vessels, the endothelial compartment (inner lining of the vein), and the vascular compartment (blood lying within the UC blood vessels) (Bongso and Fong 2013). There has been no standardized nomenclature for these structures although terms such as “subamnion”, “cord lining (sub-amnion)”, “intervascular”, “perivascular” and “HUVEC” have been frequently used. Since there is no defined histological demarcation among these compartments, it is difficult to determine whether isolated populations from different compartments have the same identity. Also, the absence of a defined consensus on the region of interest, and differences in isolation methods and derivation protocols contribute to the difficulty in identifying cell population of interest (Bongso and Fong 2013). WJ-MSCs can be isolated from two regions: the perivascular zone and inter-vascular zone (Prasanna and Jahnavi 2011), although they can also be obtained from these three regions, namely the intervascular, subamnion, and the perivascular regions (Troyer and Weiss 2008). In vitro structural, immunohistochemical and functional analyses revealed significant differences in the number, nature and properties of cells isolated from the above-mentioned regions (Nanaev et al. 1997; Karahuseyinoglu et al. 2007). Moreover, it has been assumed that they originate from different pre-existing structures (Can and Karahuseyinoglu 2007). Human umbilical cord perivascular cells (HUCPVCs) are one of the cell populations isolated from around the umbilical cord vessels (Sarugaser et al. 2005; Baksh et al. 2007). Moreover, other groups isolated other equipotent stem-like cells from the subamnion (cord lining; CL) (Kita et al. 2010; Jeschke et al. 2011). Of note, previous studies have found that WJ-MSCs populations isolated in close proximity to the amniotic surface display higher proliferative and less differentiation properties than those isolated in proximity to umbilical vessels (Nanaev et al. 1997; Karahuseyinoglu et al. 2007).
14.4 Characteristic Properties of WJ-MSCs for Cell-Based Therapy
14.4.1 Phenotypic Characterization of WJ-MSCs
A groundwork on the characterization of WJ has been provided through an overview of the human UC (Conconi et al. 2011) . In this paper, we review the phenotypic characteristics of human UC cells derived from various parts of the UC. There has been difficultly in determining the precise identity of UC stromal cells because of lack of standardized extraction, culture, and analysis methods. However, it was demonstrated that cells from WJ carry the mesenchymal phenotypic characteristics including the expression of specific lineage cytoskeletal markers, such as smooth muscle actin (SMA) and vimentin. Moreover, ESC markers Oct-4, stage-specific embryonic antigen 4 (SSEA4), nucleostemin, SOX-2 and Nanog are expressed by some populations, although HUCPV cells do not express Oct-4 and SSEA4. Moreover, HUCPV cells do not express cell surface molecules such as CD59, putatively involved in the regulation of the complement system and in preventing cell lysis, and CD146, an endothelial specific cell molecule also expressed on MSCs (Conconi et al. 2011). It has been hypothesized that HUCPV cells are more differentiated than WJ-MSCs. In a previous study, pan-cytokeratin staining was found to be stronger in HUCPV than in WJ-MSCs (Karahuseyinoglu et al. 2007), supporting the observation on the failure of HUCPV to differentiate into neuronal cells. Recently, another group characterized the role of the ESC marker SSEA4 in preserving the multipotency of WJ-MSCs. This group found that the stemness of WJ-MSC explants and their capacity to differentiate into osteocytes and adipocytes depended on the expression of SSEA4. Non-muscle myosin II (NMII), a protein that participates in cell division, migration and differentiation, is crucial in the promotion of the proliferative activity of WJ-MSCs. Accordingly, it has been found that inhibiting NMII leads to
/
arrest of WJ-MSCs, thereby attenuating the proliferative property of WJ-MSCs. Consequentially, this leads to activation of cell cycle inhibitory genes, such as CDKN1A, CDKN2A and CDKN2B, and attenuation of the expression of genes that promote S/M phase transition (Sharma et al. 2013). A recent study compared WJ-MSCs transcriptomes after 4 and 12 passages to investigate the effect of prolonged cell culture on gene expression of WJ-MSCs (Gatta et al. 2013). Among the differentially expressed genes found after the 12th passage but not in the 4th passage are those involved in inflammatory and cell stress response, cell proliferation and maturation, and apoptosis. These findings suggest that WJ-MSCs develop characteristics common in aging cells such as decreased proliferative capacity and apoptosis resistance after multiple cycles of cell expansion (Gatta et al. 2013) .


It has also been found that the macrophage marker CD14 is expressed only by cord lining mesenchymal stem cells (CL-MSCs) and not by WJ-MSCs (Kita et al. 2010). Furthermore, soluble CD14 has been shown to downregulate T-cell activation (Rey Nores et al. 1999). In addition, WJ-MSCs express HLA-G6 isoform, an immune-modulation protein, suggesting the suitability of WJ-MSCs for cell-based therapy. It was found that cell populations with different phenotypic profiles are not only present in various parts of the UC but also inside the same UC regions, indicating that UCMSCs are a unique cell family showing varying levels of stemness. Moreover, WJ CD105(+)/CD31(−)/KDR(−) cell populations have also been observed to differentiate in vivo into myogenic lineage and assist in muscle regeneration. This was also confirmed by in vitro assays which showed myogenic differentiation potential of CD105(+) cells (Conconi et al 2006) .
Specific regions of the UC lining (sub-amnion) and WJ enriched with stem cell niches have been defined (Jeschke et al. 2011). A previous study reported MSCs isolation from sub-amnion (cord lining) of the UC and that sub-amnionic MSCs from ESCs do not show tumorigenicity in vitro (Kita et al. 2010).Moreover, isolated CL-MSCs expressed mesenchymal features in vitro but also showed several distinct features (Kita et al. 2010). As stated previously, UC-isolated multipotent cells show heterogeneity due to anatomically distinct zones in the UC from which they are obtained, and also differences in isolation techniques . However, although CL-MSCs have promising therapeutic potentials due to their multipotent and proliferative capacities, harvesting these cells is an extremely time-consuming process, and this limits their utility for cell-based therapy (Jeschke et al. 2011). Nevertheless, WJ is a good source for MSCs although WJ-MSCs show variation in cell quality. Therefore, it is important to consider both the quality and quantity of the stem cells for each specific application.
It is believed that the biological characteristics of MSCs can be affected by the perinatal environment. Studies have shown that the intrauterine metabolic disturbances occurring during pregnancy, such as those caused by maternal hyperglycemia, increase the odds of the offspring to develop diabetes and obesity (Dabelea and Pettitt 2001; Gillman et al. 2003; Clausen et al. 2008). Results from animal studies have shown that MSC commitment to pre-adipocytes and mature adipocytes begins during fetal development and the perinatal life (Tang et al. 2008). Further, it was found that obese subjects showed lower amounts of pre-adipocytes and mature adipocytes than normal subjects (Tchoukalova et al. 2007). However, more studies need to be conducted to examine the impact of the perinatal environment on differentiation of fetal MSCs, especially in unregulated gestational diabetes. The underlying mechanism causing metabolic diseases in offsprings of diabetic mothers has been investigated (Pierdomenico et al. 2001). One study found similarities in the types of markers expressed on isolated WJ-MSCs from the UC of healthy and diabetic mothers, but differences in the expression levels of these markers. This phenomenon can be explained by the differences in the functional features between the two groups (i.e., healthy and diabetic mothers). Accordingly, cells from the diabetic mother showed limited plasticity as exemplified by lower expression levels of CD90. However, these cells also showed proliferative features, attributable to high expressions of CD44, CD29, CD73, CD166, SSEA4 and telomerase reverse transcriptase (TERT) in isolated WJ-MSCs. Also, isolated WJ-MSCs from the diabetic group showed higher capacity to differentiate into adipocytes indicating a possible pre-commitment to adipocyte lineage. In summary, many studies suggest “pre-commitment” of MSCs to the adipocyte lineage due to a diabetic uterine environment. This causes higher adipocyte production and when coupled with an unhealthy diet lifestyle, will lead to diabetes and obesity .
14.4.2 Immunomodulatory Properties of WJ-MSCs
WJ-MSCs are characterized by low immunogenicity and high proliferative capacity, which make them favorable for allogenic and xenogenic transplants . In addition, WJ-MSCs possess immunosuppressive characteristics (Prasanna and Jahnavi 2011). Specifically, it was found that WJ-MSCs lack the cell surface expression of major histocompatibility complex (MHC) class II (HLA-DR) and the co-stimulatory antigens CD80 and CD86, which are involved in the activation of T and B cell responses (Rachakatla et al. 2008; Weiss et al. 2008; La Rocca et al. 2009; Prasanna and Jahnavi 2011). Moreover, MHC class I antigens (HLA-ABC) are less expressed in WJ-MSCs, which may protect them from natural killer cell-mediated lysis (Rachakatla et al. 2008; Weiss et al. 2008; La Rocca et al. 2009; Prasanna and Jahnavi 2011). Moreover, there appears to be differences in the induction of pro-inflammatory cytokines between bone marrow-derived MSCs (BM-MSCs) and WJ-MSCs, although both cell types display similarities in the expression of immune-stimulatory ligands. WJ-MSCs secrete immunosuppressive proteins including interleukin (IL)-6 and vascular endothelial growth factor (VEGF), which are key players in the immunosuppressive capability of MSCs (Djouad et al. 2007; Weiss et al. 2008) . They also express HLA-G, a protein which suppresses the immune response against the fetus, induces the expansion of regulatory T cells (Djouad et al. 2007; Selmani et al. 2008; Weiss et al. 2008; La Rocca et al. 2009; Griffin et al. 2010; Prasanna et al. 2010; Deuse et al. 2011) and plays pivotal role in the activation of the regulatory T-cells during pregnancy. Some studies showed low rejection rate associated with expression of HLA-G and other antigens (e.g. IL-10, transforming growth factor [TGF]-β) in the blood, heart and liver/kidney grafts (Zarkhin et al. 2010). When compared to BM-MSCs, WJ-MSCs were found to exhibit higher immuno-modulating responses as exemplified by higher amounts of the anti-inflammatory molecules IL-10 and TGF-β (Selmani et al. 2008; Weiss et al. 2008; La Rocca et al. 2009; Prasanna et al. 2010). Also, another study compared the responses to the pro-inflammatory cytokine interferon (IFN)-γ between these two cell populations. Due to the absence of HLA-DR in WJ-MSCs, WJ-MSCs showed negligible induction by IFN-γ while BMSCs exerted substantial responses (Prasanna et al. 2010; Deuse et al. 2011). The immuno-modulatory capabilities of WJ-MSCs in diabetic rat models have also been suggested and some studies found suppressive effect of WJ-MSCs on the proliferation of allogenic T-cell response (Wang et al. 2014). Furthermore, these studies also demonstrated the absence of CD40, CD40L, CD80 and CD86 expression by WJ-MSCs. When co-cultured with peripheral blood lymphocytes, WJ-MSCs caused a remarkable decrease in the levels of IFN-γ secreted by these cells. Additionally, WJ-MSCs also exerted therapeutic benefits in type-1 diabetic rats by reducing pancreatic cell destruction and hyperglycemia, most likely by decreasing the inflammatory response against β-cells of the pancreas (Wang et al. 2014) .
Nevertheless, UCMSCs can also elicit an immune response. Accordingly, although single injection of MHC-mismatched inactivated UCMSCs did not induce a detectable immune response, repeated injection in an inflamed region or upon stimulation with IFN-γ prior to injection unmasked immunogenic properties of UCMSCs (Cho et al. 2008). These findings indicate that caution must be exercised when injecting UCMSCs repeatedly into inflamed or damaged regions. Generally, all MSCs have immunosuppressive properties as they inhibit CD4(+), CD8(+), CD2(+) and CD3(+) subsets (Aggarwal and Pittenger 2005). However, relative to BM-MSCs, WJ-MSCs have been found to display more robust immuno-modulating activity as evidenced by more remarkable mitogen-driven alloantigens and specific antigen-driven T cell responses. Some studies also revealed that WJ-MSCs caused dose-dependent attenuation of the T-cell immune response in vitro (Di Nicola et al. 2002). Moreover, WJ-MSCs also caused significant suppression of mitogen induced CD3(+) T cell activity even when administered at low doses, compared to their bone marrow-derived counterparts (Najar et al. 2009; Prasanna et al. 2010). Another study asserted that WJ-MSCs have more potent suppressive properties compared to BM-MSCs or adipose-derived MSCs when co-cultured with allogenic-stimulated T-cells (Prasanna and Jahnavi 2011). When compared to WJ-MSCs, the immuno-modulating activity of fetal liver-derived MSCs is limited only to the attenuation of mitogen-driven lympho-proliferative activity, although they have no effect on allo-proliferative responses (Gotherstrom et al. 2003). In this regard, perinatal MSCs not only seem to attenuate lymphoproliferation more robustly that BM-MSCs similar to WJ-MSCs, but also operate in a stimuli-independent fashion, unlike fetal MSCs (Prasanna and Jahnavi 2011) . Another mechanism may be consequential to the influence of WJ-MSCs on the maturation and activation of dendritic precursors (DCs), as shown by experiments involving co-culture of WJ-MSCs with CD14(+) monocytes. Accordingly, WJ-MSCs inhibited differentiation of DCs in a contact-dependent manner when these cells were co-cultured with CD14(+) monocytes. Moreover, WJ-MSCs co-cultured monocytes appeared to be locked in an immature dendritic cell-stage and did not show upregulation of the co-stimulatory ligands essential for their maturation (Tipnis et al. 2010). Previous studies have focused on the kinetic patterns of pro-inflammatory cytokine secretion by phytohemagglutinin (PHA)-activated lymphocytes when co-cultured with WJ-MSCs and BM-MSCs (Prasanna et al. 2010). A change in the threshold and kinetics of the activating cytokine IL-2 was only observed in BM-MSC co-cultures. Moreover, results showed that activation of negative co-stimulatory ligands on peripheral blood lymphocytes was higher in WJ-MSCs co-cultures compared to BM-MSCs co-cultures (Prasanna et al. 2010). The secretion profiles of WJ-MSCs are similar to those of other MSC populations, except for the release of IL-12, IL-15 and platelet-derived growth factor (PDGF), which are secreted only by WJ-MSCs and cord blood MSCs. In summary, WJ-MSCs display robust immunomodulatory properties via several mechanisms including secretion of immunosuppressive soluble factors, upregulation of negative co-stimulatory ligands, production of memory cells, cell fusion to escape recognition, immune fetal-maternal specific immune avoidance mechanisms, attenuation of antigen-presenting cell functions, altered migration of immune cells, and T cell energy apoptosis tolerance (Prasanna and Jahnavi 2011) .
14.5 Clinical Applications of WJ-Derived Stem Cells
14.5.1 Cancer Therapy
Stem cells can also be utilized for primary and metastatic cancers. Previous studies have demonstrated the capacity of un-engineered human and rat UCMSCs to attenuate amplification of multiple cancer cells in vivo and in vitro (Ayuzawa et al. 2009; Ganta et al. 2009). Tamura et al. (2011) also reported intrinsic stem cell-dependent regulation of cancer growth, probable mechanisms underlying the observed biological function, delivery of exogenous anti-cancer agents, and potential clinical applications. By showing intrinsic ability to secrete factors that can inhibit cancer cell growth and/or apoptosis in vitro and in vivo, UCMSC may have several advantages for cell-directed cancer therapy. Two potential mechanisms have been suggested to explain the manner by which naïve UCMSCs attenuate tumor growth. First, UCMSCs may produce multiple secretory proteins that induce death of cancer cells and cell cycle arrest. Caspases are activated by the secretion of many secretory proteins that lead to cell cycle arrest and eventually cell death (Deuse et al. 2011; Ganta et al. 2009; Nakamizo et al. 2005). Some evidence reported UCMSCs may stimulate caspase activities and arrest cell cyle even in the absence of direct contact with cancer cells. Microarray analysis of rat UCMSCs also revealed over-expression of multiple tumor suppressor genes (Tamura et al. 2011). The second mechanism may involve enhancement of an immune reaction to cancer cells. Immunohistochemistry analysis revealed CD8(+) T cell infiltration of the tumor tissues which is not in line with low immunogenicity profiles of UCMSCs (Ganta et al. 2009). Therefore, the immunogenicity of UCMSC in tumor-bearing animals may be dependent on the microenvironment of UCMSC and the tumor cells.
The interaction between cytokines/growth factors and their receptors mediate the homing ability of stem cells. Accordingly, tumor cells secrete large amount of cytokines and growth factors which are detected by their corresponding receptors expressed on the surface of the UCMSC and other MSCs (Tamura et al. 2011). Nevertheless, UCMSCs show greater migration capacity towards the tumor tissue than BM-MSCs due to overexpression of IL-8 receptor and C-X-C chemokine receptor (CXCR) in UMSCs. Furthermore, UMSCs can also be engineered to express cytotoxic cytokines before being delivered to the tumor, and can be preloaded with nanoparticle payloads which could attenuate tumors after they reach the tumor site (Rachakatla et al. 2007; Matsuzuka et al. 2010). Using these manipulations, human UCMSC expressing IFN–β produced sufficient amounts of IFN–β which induced death of human breast adenocarcinoma and bronchioalveolar carcinoma cells in vitro and in vivo (Rachakatla et al. 2008; Matsuzuka et al. 2010). Altogether, these studies suggest therapeutic potential of UCMSCs for the treatment of various cancers. Furthermore, UCMSCs are also suitable for allogenic transplantation due to their abundance, low immunogenicity, lack of CD34 and CD45 expression, and less complex methods involved in harvesting these cells and during in vitro cell expansion. Importantly, the homing ability of UCMSCs to inflammatory tissues including cancer tissues, and the tumoricidal ability of these cells make them ideal stem cell-based intervention for cancer therapy .
14.5.2 Liver Disease
Based on the potentiality of UCMSCs to differentiate into an endodermal lineage including hepatocyte-like cells, WJ-MSCs can also be considered for the treatment of liver disease, furthermore, as an alternative to orthotopic liver transplantations. The in vitro and in vivo use of UCMSCs for rescuing injured hepatocytes has been described previously (Scheers et al 2011). UCMSCs have also been shown to display several hepatic markers that characterize the sequential steps of liver development. In vivo studies showed the capacity of transplanted undifferentiated UCMSCs to express human hepatic markers such as albumin and alpha- fetoprotein (AFP) in the liver of severe combined immunodeficiency (SCID) mice with partial hepatectomy at 2, 4, and 6 weeks after in vivo transplantation (Campard et al. 2008). Furthermore, there is some evidence which showed decreased rate of hepatic cell fibrosis due to compensatory effects of transplanted undifferentiated UCMSCs. This finding thus indicates supporting role of UCMSCs in enhancing functional recovery of recipient livers, probably via stimulation of the differentiation of endogenous parenchymal cells and promoting degradation of fibrous matrix, even in the absence of a transdifferentiation process (Anzalone et al. 2010). Meanwhile, the capacity of UCMSCs to differentiate into a hepatic lineage can be enhanced in vitro and in vitro via co-culture with hepatogenic factors. A previous study also found relationship between anti-inflammatory and anti-fibrotic actions of UCMSCs due to secreted metalloproteinases (Anzalone et al. 2010). Nevertheless, further research is required to clarify the ability of UCMSCs to differentiate into hepatocyte-like cells and their capacity to repopulate and rescue liver functions.
14.5.3 Cardiovascular Diseases
The therapeutic value of WJ may also be explored for cardiovascular diseases (Semenov and Breymann 2011). Due to disadvantages of surgical treatment using non-autologous valves or conduits (Mayer 1995; Schoen and Levy 1999), cardiovascular tissue engineering focused on in vitro fabrication of autologous, living tissues with the potential for regenerating heart muscles (Shinoka et al. 1996). A number of animal studies have validated WJ-MSC-based cardiovascular tissue engineering. In one study, autologous trileaflet heart valves derived from human WJ-MSCs were successfully implanted in sheep models for up to 20 weeks. The functional, biochemical and structural features of these valves were comparable with those of the native semilunar heart valves. The feasibility of obtaining stem cells from UC for cardiovascular tissue engineering in contrast with obtaining cells from other sources has also been demonstrated (Kadner et al. 2004). Another study was conducted to develop a myocardial patch to repair myocardial infarction tissue or slow down tissue damage, and enhance long-term function of the heart (Kenar et al. 2011). This study used a 3D aligned microfibrous myocardial tissue construct cultured under transient perfusion. Accordingly, 3D constructs from static and perfused cultures increased cell viability, provided uniform cell distribution and alignment due to supply of nutrients from the 3D structure.
Indeed, WJ-MSCs have great potential in cardiovascular tissue engineering. Nevertheless, we need to understand deeply the clinical limitations of these cells and establish realistic clinical protocols for their application. For instance, the use of natural, synthetic or hybrid polymers as scaffolds resulted in reduced growth and remodeling of cells, and increased risk for infection and formation of thrombo-embolus. Thus, it is necessary to develop materials that are biocompatible with cardiovascular tissue for them not to negatively affect the regenerative and immune-modulatory characteristics of WJ-MSCs in engineered scaffolds (Semenov and Breymann 2011). Furthermore, despite advanced knowledge on the characteristics of WJ-MSCs and their successful preclinical and clinical applications, standardized criteria for isolation, characterization, long-term cultivation and maintenance of human MSCs are required, as long-term survival of stem cells in the host tissue and establishment of treatment regimen are still critical issues which hamper broad clinical application of stem cells.
14.5.4 Cardiac Differentiation of Human WJ-Derived Stem Cells
Due to the capacity of undifferentiated MSCs to differentiate spontaneously into multiple lineages when transplanted in vivo, BM-MSCs may differentiate into multiple tissue types after transplantation , and its developmental fate may not be restricted by surrounding tissues after myocardial infarction. Undifferentiated cells are known to undergo maldifferentiation within the infarcted myocardium posing life-threatening consequences (Breitbach et al. 2007). Thus, cardiac differentiation must be initiated prior to transplantation of cells, which could be achieved by culturing the cells in defined culture conditions. It has been hypothesized that cardiac differentiation prior to transplantation would result in enhanced myocardial differentiation and recovery of heart functions (Bittira et al. 2002; Tomita et al. 2002). Accordingly, WJ-MSCs can be induced to differentiate into heart cells by treatment with 5-azacytidine for 3 weeks; thereafter these cells would express cardiocyte markers such as cardiac troponin I, connexin 43, and desmin, and display cardiac myocyte morphology (Wang et al. 2004). Additionally, cells may also be co-cultured with oxytocin, whose levels are higher in the fetal but not adult cardiac tissues indicating their involvement in cadiomyocyte differentiation (Fathi et al. 2009), or with embryo-like aggregates and other growth factors such as TGF-β1, PGDF and basic fibroblast growth factor (bFGF) to transform cells into myocytes (Maltsev et al. 1993; Matsuura et al. 2004; Hollweck et al. 2011). It has been observed that the treatment of UCMSC with oxytocin and 5-azacytidine caused cardiac differentiation and also the formation of “embryoid bodies” (Hollweck et al. 2011). A detailed morphological and immunocytochemical analyses showed that oxytocin is a more potent inducer of cardiac differentiation than 5-azacytindine in the formation of embryoid bodies.
The long-term therapeutic effects of MSCs derived from the BM-MSCs and WJ-MSCs were compared (Lopez et al. 2013) in a rat model of myocardial infaction (MI). Results showed significant improvement in groups which received MSCs at 25–31 weeks after treatment with MSCs. Moreover, cardiac differentiation potential of WJ-MSC was enhanced when these cells were co-cultured with fetal MSCs, compared with those co-cultured with adult MSCs. Furthermore, combination treatment with WJ-MSC and fetal MSCs induced myotube formation in 2–3 days and spontaneous contractions (beating) after 5–7 days. Collectively, the above-mentioned studies indicate that MSCs administered 24–48 h after MI exerted strong beneficial effects which lasted longer than 25 weeks after MI, and that WJCs may be useful sources of off-the-shelf cellular therapy for MI . Due to the accessibility and differentiation capability of USMCs into cardiomyocyte-like cells, UCMSC are a favorable treatment intervention for cell-based therapies and cardiac engineering. However, in order to use UCMSCs, as well WJ-derived stem cells in cardiac repair, we need to determine whether these cells possess functional properties of cardiomyocytes.
14.5.5 Cartilage Regeneration
Exposure to traumatic injury or autoimmune diseases damages the cartilage, a tissue which displays poor in vivo regeneration and self-repair capacity. Reports on the capacity of stem cells to differentiate into chondrocyte-like cells raised enthusiasm for the use of cell therapy to repair cartilage damage. In particular, WJ-MSCs have been shown to differentiate into chondrocyte-like cells in vivo and in vitro (Lo Iacono et al. 2011). Analysis of the chondrogenic potential of WJ-MSCs revealed their multipotency and chondrogenic capacity (Arufe, et al. 2011), which are essential properties for cell therapy in articular diseases (Arufe et al. 2011). Moreover, WJ-MSCs are also capable of increasing the production of hyaluronic acid, glycosaminoglycans and key genes such as SOX9, cartilage oligomeric protein (COMP), collagen type II and fibromodulin (Fong et al. 2012). The beneficial effects of seeding density of WJ-MSCs in poly-glycolic acid (PGA) scaffolds, in the presence of a chondrogenic medium, on chondrogenic potentials of WJ-MSCs have also been described (Wang et al. 2009). This study revealed the potential for chondrogenic differentiation of WJ-MSCs in three-dimensional tissue engineering; higher seeding densities promoted better biosynthesis and mechanical integrity, and that a seeding density of at least 25 million cells/mL was sufficient for fibrocartilage tissue engineering with umbilical cord mesencyhmal stromal cells (Wang et al. 2009). Culturing WJ-MSCs on nanofibrous substrates with sequential two culture medium environments also promoted chondrogenic differentiation of WJ-MSCs (Fong et al. 2012). For successful osteochondral regeneration, cell sources and tissue integration between cartilage and bone regions must be considered because osteochondral tissue consists of cartilage and bone. A supportive structure, which mimics native osteochondral tissues, has been recently developed (Wang et al. 2011). In their study, investigators showed that embedding WJ-MSCs into two poly-L-lactic-acid (PLLA) scaffolds with chondrogenic and osteogenic media for 3 weeks, followed by suturing of the chondrogenic and osteogenic constructs to form four different osteochondral assemblies resulted in enhanced integration and transition of the matrices between two layers in the composite group compared to other control composites (Wang et al. 2011). WJ-MSCs were also found to differentiate into intervertebral disc-like tissues exhibiting immature nucleus pulposus (NP) phenotype in a pseudo-three-dimensional culture system (Chon et al. 2013). Moreover, it has been shown that these NP-derived cells possess specific laminin isoforms and laminin binding receptors, which may lead to the formation of NP-like cells. As a result, WJ-MSCs are not only promising candidates in osteochondral regeneration but also in intervertebral disc repair. Also, their low immunogenicity suggests their ideal use in autoimmune disorders such as osteoarthritis and rheumatoid arthritis. In view of the high variability of cell sources, the need for scaffold and matrices, and the necessity of co-culture with growth factors , more research is required to optimize this cellular therapy approach and translate the results obtained from bench to clinic for cartilage repair.
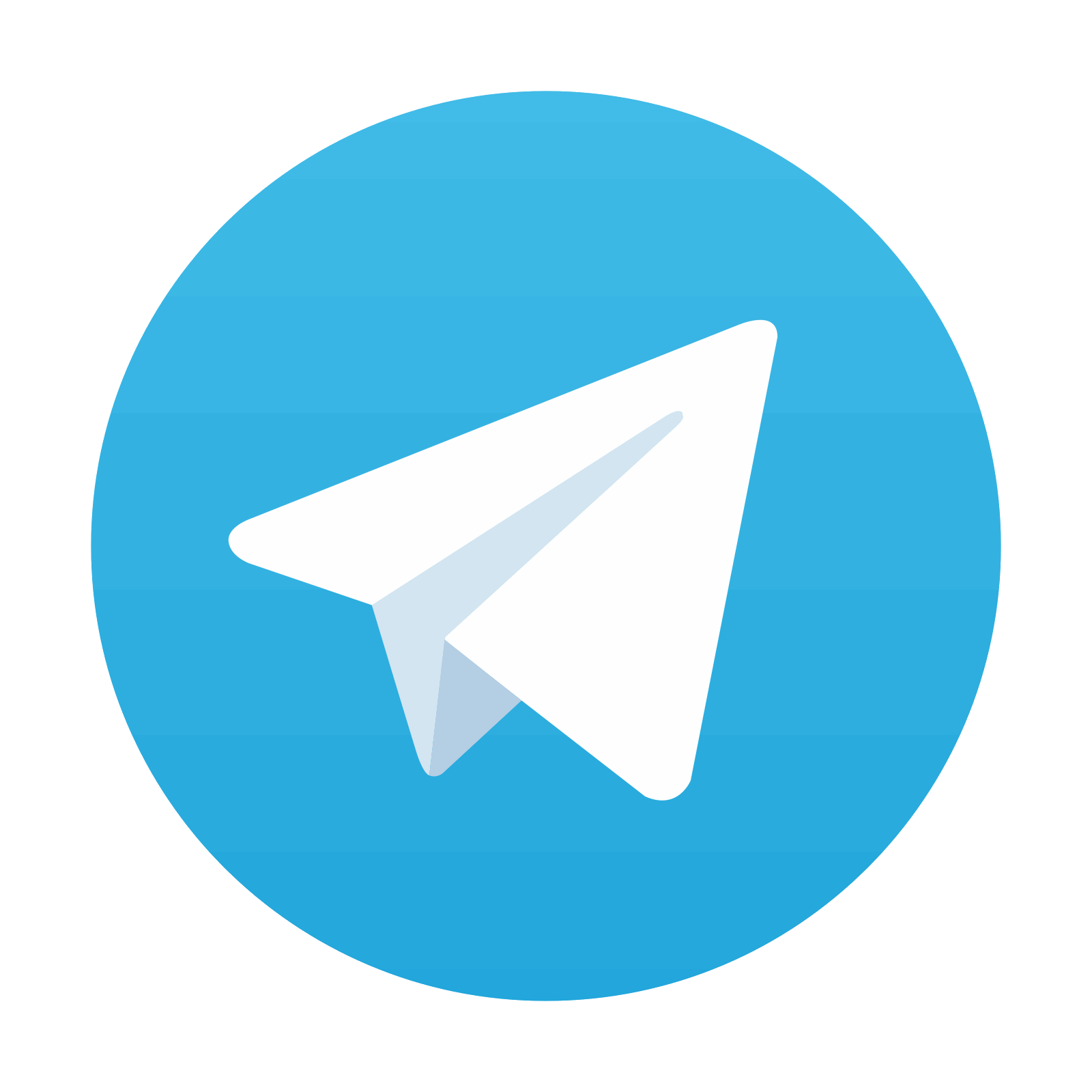
Stay updated, free articles. Join our Telegram channel

Full access? Get Clinical Tree
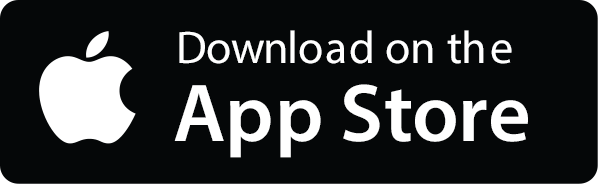
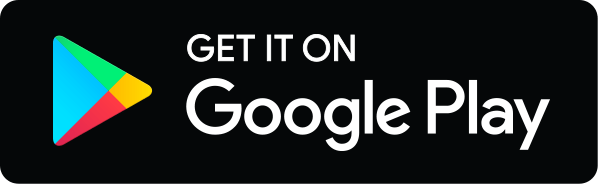