Fig. 8.1
Treatment planning study for a 3 year old child with a glioma. (a) Shows a VMAT photon plan and (b) a two field proton plan for a treatment with 54 Gy. (The threshold dose of the dose colorwash was set to 7 Gy). The low dose area L is spread out over a much larger volume with the VMAT plan, the high dose area H is nearly identical for the two techniques and highly conformal to the target. The right hippocampus h can be better spared by the proton plan
As stated above proton treatment can be especially attractive in paediatric radiotherapy as long time survivors are not at risk for developing functional impairments and secondary cancers. However, there is a lack of statistical evidence to demonstrate the clinical superiority of proton treatment. Some studies have shown that local control is comparable to photon treatment, but there are as yet no published studies on late morbidity after proton therapy (Brada et al. 2009). Some caution is also advisable, since the risk of secondary cancer might be higher than theoretically estimated for proton treatment because of neutron contamination with some proton delivery systems. There are no randomized controlled trials comparing the therapies in children and it is controversial whether conduction of such trials would be possible or desirable. Right now there are not enough proton facilities worldwide to meet the needs for treatment of paediatric patients therefore it is not realistic to expect that all children needing radiotherapy will be treated with protons. Accordingly, a case selection is necessary. It must be emphasized that protons are mainly superior to photons when patients are treated for cure. In case of palliative radiotherapy there is no or very little advantage of protons.
As large randomized controlled face-to-face trials comparing modern photon and proton radiotherapy are not expected to be conducted, it is important that all information on children treated on routine basis are collected in databases and evaluated. It is therefore desirable that all children treated with modern treatment modalities such as photon IMRT or proton radiotherapy are included in large multi-institutional databases for registration of local control, survival and late morbidity outcomes.
The following review will focus mainly on childhood brain tumours with a good prognosis, when proton therapy is expected to have an important impact on the long term outcome compared with modern photon treatment.
Photon Radiotherapy for Common Childhood Brain Tumours
Medulloblastoma
Primitive neuroectodermal tumours (PNET) arise from the neural crest. PNET tumours of the posterior fossa of the brain are referred to as medulloblastoma.
Due to their location adjacent to the fourth ventricle they often cause increased intracranial pressure at the time of diagnosis. They are considered as grade IV tumours in the WHO classification and have an aggressive growth not only locally but also by their potential to disseminate in the whole subarachnoid space.
Radiation therapy to the complete cerebrospinal axis combined with systemic therapy has dramatically improved survival and today medulloblastoma patients have a 5 year survival rate of 60–80 % depending on their risk profile.
Treatment for medulloblastoma includes surgical resection, radiotherapy and chemotherapy. The surgery needs to be as radical as possible. Standard risk patients have no or only residuals following surgery and no dissemination to the cerebrospinal fluid (CSF) or along the surbarachnoidal space. High risk patients have macroscopic residual tumour following surgery or spread of tumour cells to the CSF. Radiotherapy consists of irradiation of the complete cerebrospinal axis and a boost in the posterior fossa. Chemotherapy has allowed reduction of the dose to the craniospinal axis in standard risk patients and the delay of radiotherapy in small infants. A routine prescription for standard risk patients is 23.4 Gy to the craniospinal axis combined with a boost in the posterior fossa to a total dose of 54–55 Gy. High risk patients need a radiation dose to the craniospinal axis of 36 Gy followed by a boost in the posterior fossa to a total dose of 55 Gy. Concomitant single agent chemotherapy is administered along with the radiotherapy and multidrug chemotherapy is continued for several cycles after completion of radiotherapy for all medulloblastoma patients. Newer protocols and studies are aiming to confine the boost volume to the tumour bed with a small margin instead of irradiating the whole posterior fossa.
Traditional irradiation of the craniospinal axis is conducted with photons. The technique consists of two lateral fields to include the whole brain and the upper cervical spine combined with posterior fields to cover the rest of the subarachnoidal space until the sacral roots. Often two to three posterior fields have to be applied depending on the length of the spinal cord. All the fields have to be perfectly matched and it has to be assured that field junctions between the fields are neither under- or overdosed. Although only the spinal cord is the desired target to treat, the beam will exit through the organs anterior to the spinal cord, thus resulting in a considerable dose to the vertebral bodies, the thyroid gland, the heart, the gastrointestinal tract, the lungs, the kidneys and the female reproductive tract, resulting in possible side effects to multiple organs from irradiation. With modern photon based IMRT techniques doses to organs anterior to the craniospinal axis like the mediastinum, the thyroid gland or the heart as well as structures anterior to the posterior fossa like the cochleae or the pituitary gland can be spared to some degree.
Electron irradiation of the spinal axis has been proposed and is practised in some institutions. It has the advantage that with the rapid fall off of dose at depth, tissues anterior to the spinal cord can be better spared. But matching with cranial photon fields and resulting dosimetric uncertainties make electrons difficult to use. Because of insufficient range of the electrons this technique may lead to underdosage of deeply located parts of the spinal subarachnoid space.
Nowadays prospective clinical studies often stratify patients according to immunohistochemical and histological risk factors, and patients may be allocated to treatments designed according to the risk factors, but the principle of irradiating the whole subarachnoid space and boosting the tumour bed remains for the moment unchanged. Pattern of failure studies have shown that cerebral recurrences often occur in the posterior fossa, the subventricular area and the frontal area and it has been speculated whether the whole brain irradiation could be replaced with subtotal irradiation of the brain which includes these areas (Miralbell et al. 1997a, b).
Radiation remains an integral part of the therapy of medulloblastoma, but the risk for late morbidity for these patients is high because of the large volumes needing irradiation.
Low Grade Glioma
Low grade glioma originate from the glial cells. These tumours present a variety of different histologies and localisations in the brain. They often grow along the midline structures of the brain like the optical pathways, the hypothalamus and the brainstem and can also be found in the supratentorial hemispheres and the cerebellum. Long term survival is favourable in patients treated with macroradical resection. Radical resection can often be achieved in tumours of the supratentorial hemispheres, but more rarely in the cerebellum and in the midline regions where even a biopsy carries a risk of severe morbidity.
Radiotherapy is used for patients with progressing tumours where radical surgery is not possible. The timing of radiotherapy is controversial. Immediate postoperative radiotherapy in the case of residual disease has shown to increase the progression free survival, but not the overall survival. Therefore, radiotherapy is in general deferred in case of asymptomatic patients with slowly progressing low grade glioma. Radiotherapy is advised in the case of symptoms or progressing tumour at the time of recurrence. Trials are investigating the use of chemotherapy at progression of low grade glioma with the primary purpose of deferring radiotherapy as long as possible Radiotherapy is usually applied with conformal techniques with localized irradiation of the lesion and an appropriate margin for microscopic disease. The dose given is usually 45–54 Gy in 25–30 fractions depending on the site of the lesion. Small lesions have been successfully treated by some centers with stereotactic radiosurgery or fractionated stereotactic radiotherapy with favourable local control and morbidity (Kortmann et al. 2003). The overall survival of children with low grade glioma treated with radiotherapy is in the range of 80 % at 10 and 20 years after treatment.
Ependymoma
Ependymoma arise from the neuroepithelial linings of the ventricles. They are graded into grade II ependymoma and grade III anaplastic ependymoma. Two thirds of the tumours are located in the posterior fossa and one third in the hemispheres. It is mainly a neoplasm of children and adolescents, but one third of the patients are small infants (MacDonald and Yock 2010).
The standard treatment for cerebral ependymoma constists in gross tumour resection followed by radiation therapy. Radical surgery is an important prognostic factor and improves survival. If the surgery was not radical, a second look operation is often recommended. Postoperative chemotherapy may be considered in case of residual tumour (MacDonald and Yock 2010; Massimino et al. 2006).
Postoperative radiotherapy consists of invol-ved field irradiation of the tumour bed with an appropriate margin to a total dose of 54 Gy. Radiotherapy yields a local control of up to 80 % if the initial surgery was radical (MacDonald and Yock 2010).
Less than 10 % of cerebral ependymoma disseminate into the subarachnoid space or the CSF. The pattern of failure is mainly locally, and postoperative craniospinal radiotherapy has been abandoned as a standard treatment for non disseminated tumours. In cases with CSF seeding the postoperative radiotherapy should include radiotherapy to the whole craniospinal axis with 36 Gy and a local boost to the spinal metastases and the initial tumour site.
Postoperative chemotherapy may defer radiotherapy in infants younger than 3 years old. Studies on young infants have conflicting results. There are reports on long term survival in infants treated by chemotherapy without radiotherapy. On the other hand several studies have shown that the progression free survival is impaired if radiotherapy is delayed to more than 1 year after surgery (MacDonald and Yock 2010; Koshy et al. 2011).
Craniopharyngioma
Craniopharyngioma are benign tumours arising from embryonic tissue in the pituitary region and often affect the functions of the optic chiasm, the hypothalmus and the pituitary gland. They often contain a mixture of cystic components and solid components.
The primary therapy is surgery, but as the lesions are situated in the area described, in close proximity to important risk organs, radical resection is often not possible. Postoperative radiotherapy is not needed in case of total tumour resection, but salvage radiotherapy is often used in case of progression after primary surgery. The overall survival is good, in most series approximately 80 % at 20 years after diagnosis.
Radiotherapy needs to be applied with a dose of at least 50 Gy (Fitzek et al. 2006). Although this tumour is often curable using combined treatment modalities, the patients often suffer from side effects related to therapy.
Intracranial Germinoma
Intracranial germ cell tumours are rare tumours that arise in the region of the third ventricle. Histologically, they are divided – like germ cell tumours in other locations – into pure germinoma and non-germinomateous germ cell tumours. In this section intracranial pure germ cell tumours will be described. These germ cell tumours may occur in children and in adolescents and have an excellent prognosis. Surgery may be omitted unless a histological confirmation of the diagnosis in addition to serum markers and MRI-scans is needed. These tumours may be cured with radiotherapy alone. Previously, radiotherapy of pure intracranial germ cell tumours included the whole craniospinal axis with a boost in the tumour region. Nowadays, the therapy consists of combined chemo- and radiotherapy where the later is restricted to the periventricular volume and a boost to the tumour volume.
Morbidity After Radiotherapy for Childhood Brain Tumours
In radiation therapy it is important to distinguish between acute, temporary toxicities like headache, nausea or fatigue starting during the course of radiotherapy and late complications. Acute side effects are often due to edema and are usually treated with steroids and disappear in less than 3 months after the treatment. Late toxicity is defined as toxicity appearing later than 3 months after the treatment, these side effects usually do not resolve and may become permanent. Neurocognitive impairments, neuroendocrine deficits, hearing loss, impaired vision, increased risk for vascular insult and secondary cancer are examples of late reactions caused by irradiation of the brain. Impaired thyroid gland-, cardiac- and pulmonary function, growth retardation and infertility may be late manifestations of irradiation of the cranio-spinal axis. The development of these morbidities is dose and often also volume dependent.
Neurocognitive Problems After Radiotherapy
Varying degrees of neurocognitive changes after cerebral irradiation unfortunately are a frequent occurrence and often result in reduced quality of life. Survivors have a lower chance of getting employed and of getting married (Fossati et al. 2009). The brain tumour itself and the surgical procedure may lead to intellectual deficits. This may be further worsened however by structural changes caused by radiation therapy. Endocrine disturbances and ototoxicity may attribute to the neurocognitive problems as well. Neurocognitive decline is most pronounced after whole brain irradiation. The mechanism involved is mostly white matter loss, but vascular factors and glial cell atrophy may be responsible as well (Fossati et al. 2009).
Neurocognitive disturbances are more pronounced after irradiation of children than in adolescents or adults. Neurocognitive impairment is dose dependent and reduction of the whole brain irradiation dose from 36 to 23.4 Gy in standard risk medulloblastoma patients has resulted in less neurocognitive decline (Fossati et al. 2009).
The neurocognitive function has been correlated to the dose delivered to the whole brain, supratentoriel brain, and the temporal lobes. Regarding the dose to the supratentorial brain it was shown in a study of childhood ependymoma that the risk of neurocognitive loss was related not only to the irradiation of the high dose volume (> 40 Gy), but also the low dose volume (< 20 Gy) and even the very low dose volume (< 5 Gy). This has to be considered when treating these patients with IMRT because the very low dose and low dose volume will be increased with IMRT (Merchant et al. 2005). Unfortunately the supratentorial brain and temporal lobes, as well as the subventricular and hippocampal areas may often not be spared in the therapy of midline and supratentorial tumours. However, the dose to the brain outside the target should be kept at a minimum.
Neuroendocrine Problems After Radiotherapy
Endocrine effects occur as a consequence of irradiation of the hypothalamic-pituitary axis. Growth hormone deficiency may be caused by irradiation of the hypothalamic region and has an impact not only on growth, but also on metabolism, cardiovascular function and neurocognitive function. The threshold dose for development of neuroendocrine dysfunction is low; when doses > 16 Gy are given to the hypothalamus the patient may develop growth hormone deficiency. The higher the dose to the hypothalamus, the higher is the risk for growth hormone deficiency and the earlier it may appear (Merchant et al. 2011). This condition can be treated by growth hormone substitution. Accordingly it is important that children who have received radiotherapy to the hypothalamic region are screened regularly (Fossati et al. 2009).
Irradiation of the pituitary gland will often result in dysfunction of the gonadotropine axis. This may result in precocious puberty which together with growth hormone deficiency and irradiation of the vertebral bodies during craniospinal irradiation may lead to growth retardation. Patients should also be screened for adrenal cortical hormone deficiency and thyroid hormone deficiency due to a hypopituitarism.
Vascular Problems After Radiotherapy
It has been demonstrated that children treated for brain tumours have a higher risk of vasculopathy like Moyamoya disease and strokes and also for developing vascular malformations and aneurysms. In the childhood cancer survivor study cohort it has been shown that the relative risk of developing stroke after irradiation for childhood brain cancer was 7 % higher for patients than for their siblings, 25 years after radiotherapy. There was proposed a dose effect, with the risk for stroke increasing at doses above 30 Gy and the risk being highest at doses above 50 Gy. Endocrine changes can also attribute to this. Vascular changes after radiotherapy are more often seen in children with neurofibromatosis NF1. Venoocclusive disease and Moyamoya vasculopathy may already develop in the first years after radiotherapy. Mineralizing microangiopathia can already be seen at doses as low as 15 Gy. Some adult survivors are also at increased risk of developing migraine attacks several years after treatment (Morris et al. 2009).
Auditive Problems After Radiotherapy
Ototoxicity results from irradiation of the cochlear region and is a sensorineuronal hearing loss. It is dose dependent and the threshold dose has been estimated at 35 Gy (Moeller et al. 2011). Hearing loss therefore often occurs if tumours are located in the posterior fossa, as it is the case for medulloblastoma or ependymoma. The risk is increased by addition of chemotherapy such as cisplatinum which is often given with radiotherapy in the treatment of medulloblastoma. New generations of protocols allowing restriction of the irradiated volume to the tumor bed instead of the whole posterior fossa will most likely have an impact on the risk of hearing loss. With modern photon based IMRT techniques the inner ears can often be spared.
Visual Problems After Radiotherapy
Reduced vision due to development of cataract may occur after irradiation of the lens at low doses of 7 Gy. In young children the frontal sinus is not well developed and therefore the cribriform plate is often located at the same level as the lens and the lens may receive some dose during radiation treatment of medulloblastoma. In pattern of failure studies, recurrences appeared at the cribriform plate because of shielding of the lens (Miralbell et al. 1997a, b). However, as cataract is relatively easily treatable, the dose coverage to the cribriform plate should not be compromised. Vision loss due to irradiation of the chiasm and the optical nerves is rare, as the dose of 54 Gy customarily employed for irradiation of childhood brain tumours is generally tolerated by the optic apparatus.
Secondary Cancer After Radiotherapy
Cancer secondary to irradiation is a concept founded mainly on radiobiological models as well as the experience of following the Japanese atomic bomb survivors. The field is filled with uncertainties and conflicting results.
Carcinogenesis is derived partly from the primary irradiation of the radiation field. This risk will probably be reduced in proton treatment as less radiation is necessary to achieve the same coverage of the target. But carcinogenesis is also induced by secondary radiation. In photon treatment secondary radiation is due to leakage from the accelerator head and multileaf-collimator. This stray irradiation is difficult to estimate and is generally not reported by treatment planning systems. With conformal radiotherapy techniques the tumour is irradiated through few portals with a homogenous dose and secondary cancers are expected to arise in the high dose volumes along with the treatment fields. In modern IMRT the high dose volume is reduced at the expense of increased medium and low dose volumes. It is speculated if IMRT techniques increase the risk of secondary cancer induction (Hall 2006).
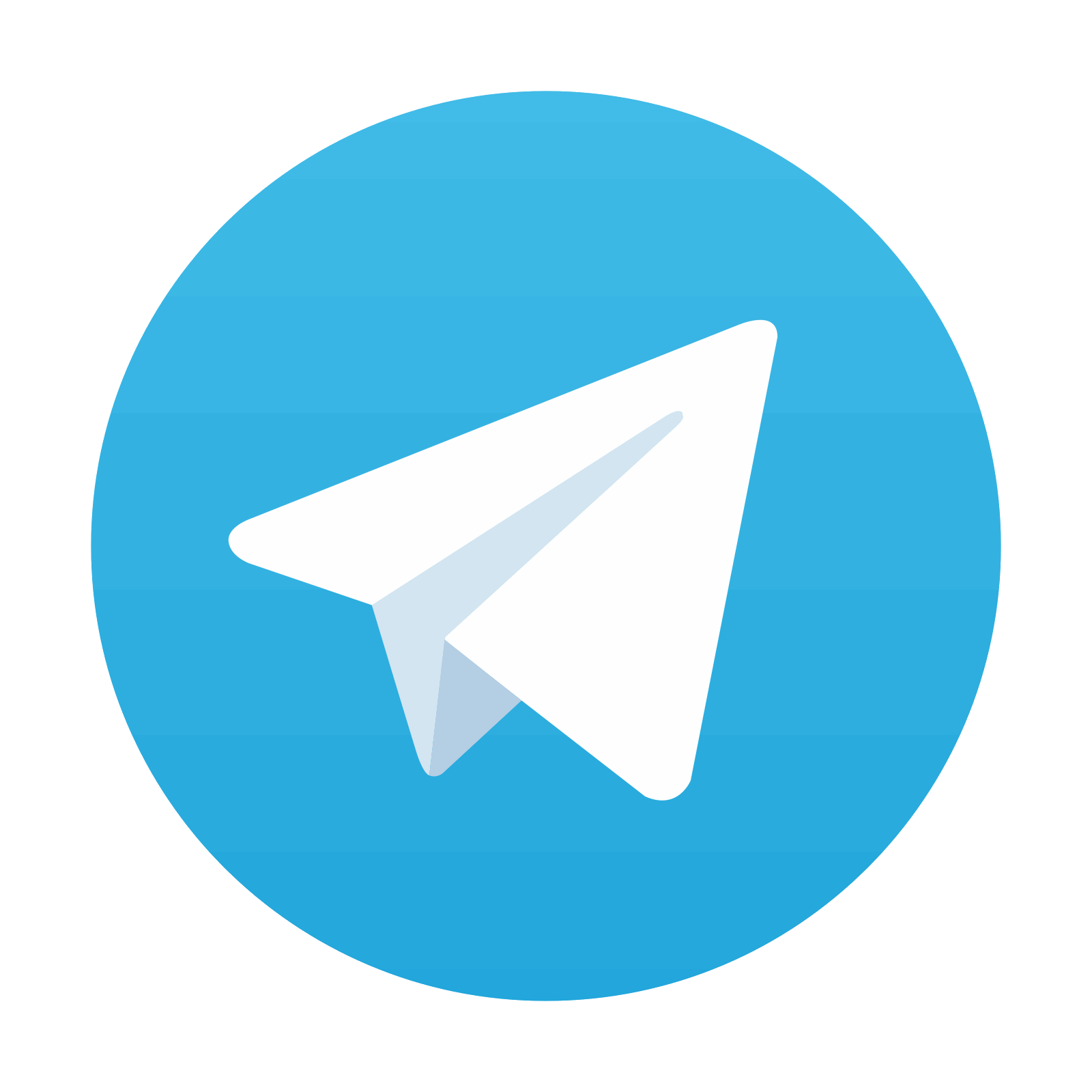
Stay updated, free articles. Join our Telegram channel

Full access? Get Clinical Tree
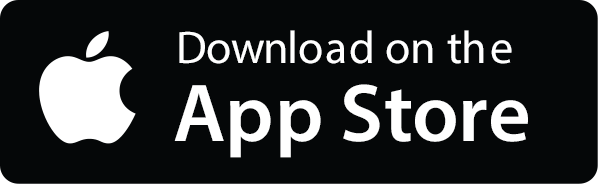
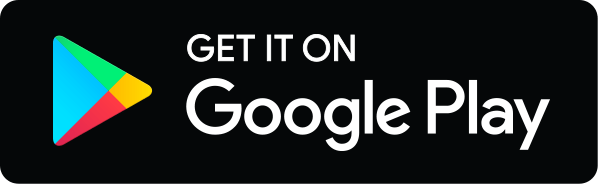