© Springer Japan KK 2017
Kenji Kosaka (ed.)Dementia with Lewy Bodies10.1007/978-4-431-55948-1_33. Cholinergic Pathology in Dementia with Lewy Bodies
(1)
Institute of Neuroscience, Newcastle University, Newcastle upon Tyne, NE4 5PL, UK
Abstract
Cholinergic dysfunction is a major feature of dementia with Lewy bodies (DLB) and makes a significant contribution to the cognitive impairment and other challenging symptoms seen in this condition. Despite this, or indeed, because of this, manipulation of the cholinergic system can offer significant therapeutic opportunities for treating DLB, and this is reflected in the fact that cholinesterase inhibitors remain one of our best treatments in this condition. In this chapter, we will explore the pathology of cholinergic dysfunction in DLB, review findings from both post-mortem and imaging studies and place this within a clinical framework.
Keywords
CholinergicDementia with Lewy bodiesAcetylcholine3.1 Introduction
To date, cholinesterase inhibitors have been our most successful therapeutic intervention in dementia with Lewy bodies (DLB) supporting the concept that cholinergic dysfunction is central to understanding DLB.
In this chapter, we will examine the salience of the cholinergic system to DLB by considering a number of perspectives including pathological evidence of dysfunction of this system, in vivo imaging data and, throughout, provide a clinical perspective which provides a useful subtext. Given the overlap between DLB and Parkinson’s disease with dementia (PDD), we also make reference to this as well as make relevant comparisons with the pathology and symptoms of Parkinson’s disease (PD) and Alzheimer’s disease (AD) as part of our exegesis.
It is important to acknowledge that other neurotransmitter systems (e.g. dopaminergic, noradrenergic and serotonergic) are likely to be relevant in the aetiology of DLB and are emerging as potential targets for therapeutic manipulation. For example, there are case reports advocating the use of atomoxetine (norepinephrine) for fatigue and emerging double blind clinical trial data supporting the use of pimavanserin (serotonin 2A antagonist) in psychosis in PD [1, 2]. Aside from dopamine, however, their role is only partially understood. Thus, for the purposes of the present chapter, we will focus mainly on the cholinergic system and justify this given the clear therapeutic implications of targeting this system.
3.2 Pathology
Studies in post-mortem tissue of the presynaptic cholinergic enzyme detected biochemically in the cerebral cortex, and of neuronal density in the basal forebrain, identified cholinergic deficits in PD and DLB over 25 years ago [3, 4]. Studies since then have extended to consider other cholinergic nuclei and receptors.
3.2.1 Nucleus Basalis and Cerebral Cortex
Ninety percent of neurons in the nucleus basalis of Meynert (nbM) are cholinergic and send widespread cortipedal projections to the entire cortical mantle, and thus this nucleus is a key driver of cholinergic drive in the brain. It is also notable that from a Lewy body disease perspective that Friedrich Lewy first observed inclusion bodies, not in midbrain dopaminergic neurons, but in the nbM [5].
In DLB and PDD, cell loss in the nbM and cortical cholinergic deficits are at least as extensive as in AD and correlate with cognitive decline. In a recent stereological analysis of the nbM, Hall et al. (2014) [6] assessed α-synuclein pathology in demented and non-demented patients with PD, together with choline acetyltransferase activity in the hippocampus and frontal cortex. Despite variable cell loss in the region of Ch4, a major nbM subgroup supplying cholinergic input to the cortex and amygdala, cortical but not hippocampal cholinergic activity was consistently reduced in PD without dementia. This relative structural preservation of Ch4 nbM despite cortical cholinergic loss suggests that prior to neurodegenerative change, there are marked functional impairments in cholinergic activity, and this has been attributed to α-synuclein pathology reducing neurotransmitter production. Once dementia becomes manifest in PD, however, there is an increase in the severity of α-synuclein pathology in the basal forebrain and hippocampus and a further reduction in hippocampal cholinergic activity, suggesting that hippocampal cholinergic dysfunction may be a major contributor to dementia in PDD.
Lui et al. (2015) [7] in a recent review suggested that there is a caudo-rostral pattern of neuronal loss within the nbM in AD on the basis of accumulated data across a number of studies. In contrast, the pattern of neuronal loss in the nbM is not as clear in Lewy body (LB) disease, although the distinctively different neuropsychiatric and cognitive deficits seen in DLB and PDD are likely to reflect a differential pattern of nbM neuronal loss in LB disease to that seen in AD. Certainly up to 80 % depletion in Ch4 occurs in PD, and this is comparable to, or more extensive than, that seen in AD [3, 8, 9]. This is accentuated in PD patients with dementia and appears greater in the intermediate nbM region.
Interestingly, a recent study by Alexandris et al. (2015) [10] demonstrated galanin upregulation within the basal forebrain cholinergic system in PD and PDD patients. Galanin has been mooted to be an inducible neuroprotective factor, and thus increased activity of this neuropeptide may reflect a protective tissue response which is attempting to improve the survivability of residual cholinergic neurons, a phenomenon which has been observed in AD [11].
The aetiology of neuronal dysfunction in Lewy body diseases is also different to that of AD; AD is characterised by amyloid plaques and abundant neurofibrillary tangles, and whilst these pathologies can occur in DLB, LB disease is more typically characterised by α-synuclein pathology [12] which localises to presynaptic elements and reduces the efficiency of synaptic communication. Quite how these presynaptic changes impact upon cholinergic function in Lewy body diseases remains to be clarified, however. From a cortical perspective, pathological Lewy body load and reductions in choline acetyltransferase activity can be observed in PD patients with cognitive impairment, with the number of Lewy bodies negatively correlating with choline acetyltransferase activity, suggesting a potential link between the two [13].
In addition to this presynaptic pathology, postsynaptic cholinergic receptors are also affected in LB disorders. Based on radioreceptor binding in autopsy tissue, Piggott et al. (2003) [14] reported that muscarinic M1, M2 and M4 binding in the striatum correlated positively with increased Alzheimer-type pathology, with M2 cortical binding correlating with LB pathology. Loss of nicotine binding (a broad-spectrum ligand with binding to a range of nicotinic receptor subtypes) was reported in early studies of cortical and subcortical areas in DLB and PD [15, 16]. With the evolution of subtype-specific nicotinic ligands, a complex topographic pattern of nicotinic receptor alterations in relation to cognitive dysfunction and neuropsychiatric symptoms is now apparent (below).
3.2.2 Nucleus Basalis and Limbic Structures
Efferent cholinergic projections from the nbM are concentrated in limbic areas such as the hippocampus and amygdala. Dementia in PD is associated with reductions in hippocampal cholinergic activity and Ch4 cholinergic neurons [6]. Sahin et al. (2006) [17] found that the distribution pattern of pathology and cholinergic deficits in the amygdaloid complex in AD and DLB differ; cholinergic deficits, as measured by choline acetyltransferase activity, were more prominent in the amygdaloid subnuclei of basolateral and corticomedial groups in DLB, probably because of the combined involvement of both nbM and brainstem cholinergic nuclei in DLB.
3.2.3 Striatum
Broadly, pathological changes in the striatum are evident in Lewy body diseases; in particular, striatal amyloid beta has been implicated in the development of dementia in PD and DLB, independent of comorbid AD pathologies [18].
In regard to the cholinergic system, there is direct evidence that striatal nicotinic receptors are affected in DLB and PD, with nicotine binding reduced in both conditions [19]. This reduction appears to be accentuated by a history of neuroleptic medication. Muscarinic striatal receptors (M1) correlate negatively with cortical LB pathology and are reduced in DLB but not in AD [14]. Low M1 and dopamine 2 (D2) receptors in DLB imply altered regulation of the striatal projection neurons which express these receptors. Pimlott et al. (2004) [20] also identified loss of striatal nicotinic alpha4beta2 activity in DLB, which paralleled reductions in nigrostriatal dopaminergic markers. Reduced striatal binding of nicotinic alpha4beta2, comparable to that in PD, has been postulated as a potential index of early degeneration in nigrostriatal inputs in DLB. Ray et al. (2004) [21] reported parallel reductions in striatal alpha6/alpha3 nicotinic receptor and dopamine uptake sites in DLB, and Bohr et al. (2005) [22] observed reduced alpha6 nicotinic binding in the putamen and caudate in PD and DLB. Gotti et al. (2006) [23] used nicotinic subtype-specific immunochemistry to demonstrate extensive striatal deficits in alpha6 and beta3, compared to alpha4 and beta2, in PD and DLB.
In summation, therefore, there appears to be marked disturbances in striatal cholinergic function both in terms of intrinsic striatal cholinergic interneuron function and nicotinic/muscarinic receptor change. This is likely not only to have an impact on voluntary movement but also on cognitive function in Lewy body diseases [24], although this is not fully understood.
3.2.4 Thalamus
The thalamus has been posited to have a central role in the symptoms of DLB, including cognitive fluctuations and visual hallucinations, symptoms which also show a positive response to cholinergic therapies [25, 26].
The thalamus receives dual cholinergic innervation from both the nbM and pedunculopontine nucleus, and this innervation has been implicated in the mediation of cortical activation and attention. Thalamic presynaptic cholinergic deficits occur in Lewy body diseases, particularly after significant cortical and subcortical neurodegeneration when the dementia manifested after prolonged parkinsonism [27].
Topographically, it appears that, in PDD, mediodorsal thalamic choline acetyltransferase activity is reduced compared with PD and centromedian activity is lower in DLB with, as opposed to without, concomitant parkinsonism.
With respect to nicotinic receptors, Ray et al. (2004) [21] noted extensive reductions in the alpha6/alpha3 subunits in DLB in the centromedian, ventral lateral and ventroposterior medial thalamic nuclei. Thalamic nicotinic alpha6 was further compared in DLB with, and without, extrapyramidal symptoms [22]. Whilst reductions in the centromedian and parafascicular nuclei were apparent in both groups, decreased binding in the ventral lateral nucleus only occurred in those with symptoms. Reviewing evidence of thalamic cholinergic denervation in LB disorders and AD, Kotagal et al. (2012) [28] concluded that this occurs in PD, PDD and DLB but not in AD and contributes to the motor and cognitive abnormalities of LB disorders.
3.2.5 Brainstem
Seidel et al. (2014) [29], analysing α-synuclein-immunoreactive inclusion pathologies in brainstem nuclei and fibre tracts in PD and DLB, found Lewy bodies and Lewy neurites to be prevalent in the pedunculopontine nucleus in both conditions.
Schmeichel et al. (2008) [30] observed loss of cholinergic pedunculopontine tegmental nuclei/laterodorsal tegmental nuclei neurons in DLB that was not related to REM sleep behaviour disorder, and this was reinforced by the observations of Dugger et al. (2012) [31]; in the latter study, choline acetyltransferase immunohistochemistry was used to label these neurones. Neuronal losses in this region occurred in DLB, but not AD, and again, these losses were independent of REM sleep behaviour disorder. Therefore, whilst cholinergic losses within the pedunculopontine nucleus are severe in the Lewy body dementias, they do not appear to be aetiologically implicated in REM sleep behaviour disorder; rather losses in this region might associate more with gait dysfunction [32] or neuropsychiatric symptoms [33] (see below).
3.2.6 White Matter
White matter intensities in cholinergic pathways have previously been related to cognitive dysfunction in AD and PDD. In a structural imaging study using a visual rating scale, Park and colleagues (2015) [34] demonstrated that white matter hyperintensities were a feature of AD, DLB and PDD and related to the severity of cognitive impairment, although the degree of pathology was similar between the conditions. Whether this reflects specific cholinergic loss or reflects more general disease severity is not clear.
3.3 Neuroimaging
Monitoring the cholinergic system in the living human brain has followed autopsy tissue studies and has confirmed that there is extensive and clinically significant pathology in DLB and PDD. The first PET imaging study used a presynaptic marker of the vesicular transporter to detect extensive cortical loss in PDD [35]. Since then, PET imaging advances in LB disorders have been based on monitoring the catabolic cholinergic enzyme, acetylcholinesterase (AChE). This molecule was not, paradoxically, the subject of previous tissue studies, presumably reflecting its postsynaptic as well as presynaptic location and also co-localisation with non-cholinergic as well as cholinergic neurons.
3.3.1 Acetylcholinesterase Imaging
Acetylcholinesterase (AChE) imaging indicates that basal forebrain cholinergic system degeneration appears early in PD and progresses with the appearance of dementia. Hilker et al. (2005) [36] detected lower activity in frontal, parietal and temporal cortex in PDD compared to PD without dementia. Shimada et al. (2009) [37] reported cholinergic dysfunction in PD and DLB in the cerebral cortex, especially in the medial occipital cortex with deficits evident in early PD, was more widespread and profound in both PDD and DLB.
Both AChE and fluorodeoxyglucose PET imaging demonstrate severe reductions in the neocortex in DLB and PDD, with increasing signal diminution from frontal to occipital regions [38].
Marcone et al. (2012) [39], using AChE PET, observed comparable AChE reductions in AD and MCI (mild cognitive impairment) converters, with a more variable degree of cholinergic dysfunction in early DLB. Such imaging analyses are in agreement with the autopsy studies described above, in that thalamic cholinergic denervation occurs in DLB and PDD (but not AD) and that degeneration of thalamic cholinergic afferent projections is likely to contribute to LB disease-specific motor and cognitive abnormalities [28].
3.3.2 Nicotinic Receptor Imaging
According to O’Brien et al. (2008) [40], alterations in nicotinic alpha4beta2 receptor SPECT binding in DLB are distinct from perfusion deficits, with increases in nicotinic receptor binding in occipital lobe. Colloby et al. (2010) [41] followed this observation with the finding that the same nicotinic marker is a predictor of cognitive progression DLB as well as AD. Isaias et al. (2014) [42] concluded from SPECT nicotinic imaging in PD that upregulated cholinergic activity in striatal, motor and limbic cortical regions is evident at an early stage of disease, prior to cognitive decline.
3.3.3 Muscarinic Receptor Imaging
Using a ligand that primarily detects the M1 subtype, Colloby et al. (2006) [43] observed elevated muscarinic binding in the right occipital lobe in DLB and in both occipital lobes in PDD. Reduced binding was evident in PDD in frontal regions and temporal lobes bilaterally but not DLB. These patterns were independent of regional blood flow changes, suggesting that structural loss or perfusion deficits do not explain the deficits in binding. In a follow-up study, Colloby et al. (2016) [44] applied a spatial covariance approach to their imaging data to examine relative M1/M4 binding in PDD patients and demonstrated a pattern of relative decreased binding in basal forebrain, temporal, striatum, insula and anterior cingulate which was independent of perfusion changes. They posited that these reflected disturbances in cholinergic innervation of specific limbic-paralimbic and salience brain networks.
3.3.4 Volumetric, Perfusion and Metabolic Imaging
Using MRI, Kim et al. (2011) [45] reported that the volume of the substantia innominata (SI, which incorporates the cholinergic nbM) is equally reduced in PDD and DLB and more so than in AD. However, two recent studies [46, 47], whilst demonstrating grey matter loss in the SI/basal forebrain in DLB, found that this was not significantly greater than that seen in AD. In relation to disease progression, PD through to PD-MCI and PDD are associated with grey matter loss in the SI [45], although given the relatively small size of the SI and structural heterogeneity (as it contains many different nuclei), structural imaging studies can only provide inferential insights.
3.4 Functional Implications
Cholinergic pathologies, detected with imaging during life or in post-mortem tissue, have been implicated in a broad range of clinical symptoms – cognitive, behavioural and motor – in DLB, as well as in PD or PDD.
3.4.1 Attention and Cognition
Nucleus basalis lesions in animals block electrocortical activation and impair learning and memory, providing a clear mechanistic basis for the role of cholinergic system in cognition. In particular, an emerging consensus supports the notion that automatic orienting of attention is mediated by ‘bottom-up’, stimulus-driven signals from cholinergic nuclei, particularly the nbM. Increases in acetylcholine in cortical targets also appear to boost signal-to-noise ratios for salient stimuli, so amplifying their detection and ensuring their attentional significance [50, 51]. Pathophysiologically, the fact that cholinergic deficits are profound in DLB and PDD compared to PD and that the prototypical cognitive profile in these dementias aligns with clinical observations of major deficits in attention in DLB [52], together with the observation that cholinergic therapies such as cholinesterase inhibitors can ameliorate these deficits, strongly supports the notion that the cholinergic system is central to cognitive and attentional impairment in the Lewy body dementias.
However, are there further data and cognitive correlates to support this conjecture? Findings from structural imaging studies are variable. Hanyu et al. (2002) [53] observed atrophy of the SI, and whilst they obtained significant correlation between SI thickness and cognition (MMSE) in AD, they did not see this in PDD. In contrast, Kim et al. (2011) [45] noted in relation to PD-related cognitive impairment (PD-MCI, PDD or DLB) but not AD a significant positive correlation between the SI volume and MMSE. Choi et al. (2012) [54] also noted that SI volume correlates with cognitive performance in PD. SI grey matter atrophy in DLB patients in another study by Colloby et al. (2016) was associated with global cognitive impairment and the severity of cognitive fluctuations. However, a similar study by Grothe et al. (2014) [46] found basal forebrain atrophy in AD but not DLB was associated with lower MMSE scores although poorer visuoperceptual function was associated with SI volume in DLB. Some of this heterogeneity in associations between basal forebrain atrophy and cognition may reflect challenges with imaging such small structures and also variability in cholinergic loss between individuals with LB disease even with the same degree of cognitive impairment.
From a functional imaging perspective, Bohnen et al. (2006) [55] reported significant correlations between the degree of in vivo cortical AChE activity and tests of attentional and executive functions in PD and PDD, but no correlation between cortical AChE activity and duration of motor disease or severity of parkinsonian motor symptoms. Lorenz et al. (2014) [56] observed that nicotinic alpha4beta2 SPECT imaging in parietal and thalamic regions correlated with impairments in memory-related and perceptual cognitive tasks in PD.
Overall, it is likely that cognitive deficits do not reflect loss of cholinergic drive to one area; rather functional imaging and electroencephalography (EEG) studies suggest that the degree and pattern of cognitive loss depends upon the interrelationship of cholinergic-related pathology across multiple regions rather than within discrete brain areas [44, 57–60].
3.4.2 Neuropsychiatric Symptoms: Hallucinations, Delusions and Behavioural Disturbances
Neuropsychiatric symptoms are core features of Lewy body disorders [61] although they commonly coexist and have complex and hard to disentangle interrelationships [62].
Early retrospective clinical-pathological analyses in post-mortem tissue identified differential cortical cholinergic enzyme and receptor activities in DLB with and without visual hallucinations [63]. Court et al. (2001) [64] noted, for example, that visual hallucinations are associated with lower levels of cortical nicotinic alpha7 subtype in DLB. Increased cingulate M2 and M4 receptors in DLB are also associated with visual hallucinations in DLB [65]. Delusions appear to be associated with different cholinergic dysfunctions; Ballard et al. (2000) [63] observed that delusions are associated with elevated cortical M1 muscarinic receptors, whilst visual hallucinations were associated with lower choline acetyltransferase. Teaktong et al. (2005) [65] associated delusions in DLB with increased cingulate M2 binding.
Correlating behavioural symptoms with the deficit of global cholinergic activity, as measured in vivo by short-latency afferent inhibition (a neurophysiological technique combining peripheral stimulation with transcranial magnetic stimulation which is sensitive to cholinergic function), Marra et al. (2012) [66] found that loss of short-latency inhibition (an indirect marker of cholinergic loss) was associated with hallucinations in DLB patients. From an imaging perspective, O’Brien et al. (2008) [67] reported that nicotinic alpha4beta2 SPECT was associated in occipital cortex with visual hallucinations.
The pedunculopontine nucleus may be particularly relevant in PD for hallucinosis. Whilst there is a primary role of pedunculopontine cholinergic drive in locomotion [32], neuropathologically, cholinergic cell loss in this nucleus has been shown in hallucinating PD although not DLB patients (Hepp et al. [68]). This is supported by imaging data using voxel-based morphometry which has demonstrated correlations between visual hallucinations in PD patients and volumetric reductions in the pedunculopontine nucleus [33].
The relationships between these multiple cholinergic changes and those seen in other neurotransmitter networks, particularly dopaminergic, remain obscure (e.g. [69]). Most current theories see a core role for the cholinergic innervation of distributed perceptual and cognitive networks in accounting for neuropsychiatric symptoms, especially complex visual hallucinations [70, 71]. For example, theories for the genesis of hallucinations see a complex interplay between attentional and perceptual impairment and subsequent compensation across the ventral visual stream, thalamus and frontal cortex, all areas with significant cholinergic loss in Lewy body disorders. Modelling of these networks is in an early stage, but accumulating evidence suggests that symptoms are associated with widely distributed pathology across a number of cortical areas and pathways (e.g. [58, 59]). This complexity may illuminate why these problems commonly co-occur and the lack of specific responses seen in treatment trials (e.g. [72]). Relating particular patterns of modulation across these networks with specific symptoms remains a challenge.
3.4.3 Fluctuations in Consciousness
Disturbances of consciousness are common in DLB. Fluctuations in attention and awareness have been associated with variations in epibatidine binding to the high-affinity nicotinic receptor in autopsy temporal cortex in DLB, being higher in those more prone to fluctuations [73]. In DLB, Ray et al. (2004) [21] detected lower nicotinic alpha6/alpha3 receptors, and Pimlott et al. (2006) [74] higher alpha4beta2 in the thalamus, in subgroups of patients affected by disturbed consciousness. Concentrated in the thalamus compared with other human brain areas, nicotinic modulation of thalamo-cortical circuitry may be a key factor in control of conscious awareness in DLB. This overlaps with associations between clinically observed cognitive fluctuations and electrocortical disturbances in the low alpha (so-called pre-alpha) and theta range in DLB and PDD patients, which are known to be contingent upon cortico-thalamic activations [75].
The mechanism underlying these fluctuations, which can occur across a number of timescales [76, 77], is not well understood, though comparisons with delirium, which is also characterised by variable alertness, suggest that cholinergic hypofunction is a common factor [78]. The role of feedforward and feedback loops between the cortex, thalamus, subcortical areas and the cerebellum in maintaining stability within attentional networks is likely to be central to understanding cognitive fluctuations within Lewy body disorders, and it is likely that cholinergic (dys)function is a key player in these processes [26, 79].
3.4.4 Motor Symptoms: Gait Disturbances
The concept that cholinergic pathology impacts on motor function in LB disorders is relatively recent. Based on AChE PET studies, Bohnen et al. (2009) [80] originally suggested that in contrast to nigrostriatal dopaminergic denervation, cholinergic hypofunction in the cortex and thalamus is associated with a propensity to falls in PD. Thalamic cholinergic activity in part represents cholinergic output of the pedunculopontine nucleus, which, as noted previously, is a key centre for gait control. Subcortical cholinergic denervation, due to degeneration of brainstem pedunculopontine nucleus neurons, may thus relate to the presence of dopamine nonresponsive gait and balance impairments including falls, in PD.
Analysing cholinergic correlates of gait speed in PD, Bohnen and colleagues (2013) [80] further tested the hypothesis that gait dysfunction results from multisystem degeneration with acetylcholinesterase PET imaging indicating slower gait speed occurred in a low cholinergic PD subgroup, compared to the normal-range cholinergic PD subgroup. Comorbid cortical cholinergic denervation was proposed to be a more robust marker of slowing of gait than nigrostriatal denervation alone. Sarter et al. (2014) [81] reviewed PET studies in PD ‘fallers’ and argued that falls result from interactions between loss of basal forebrain cholinergic projections to the cortex and striatal dopamine loss. Bohnen et al. (2009) [82] obtained in vivo imaging evidence that cholinergic pathology in the PPN is associated with frequent falling in PD. From the perspective of activities of daily living, there is evidence to suggest that risky driving is linked to thalamic cholinergic denervation in PD [83].
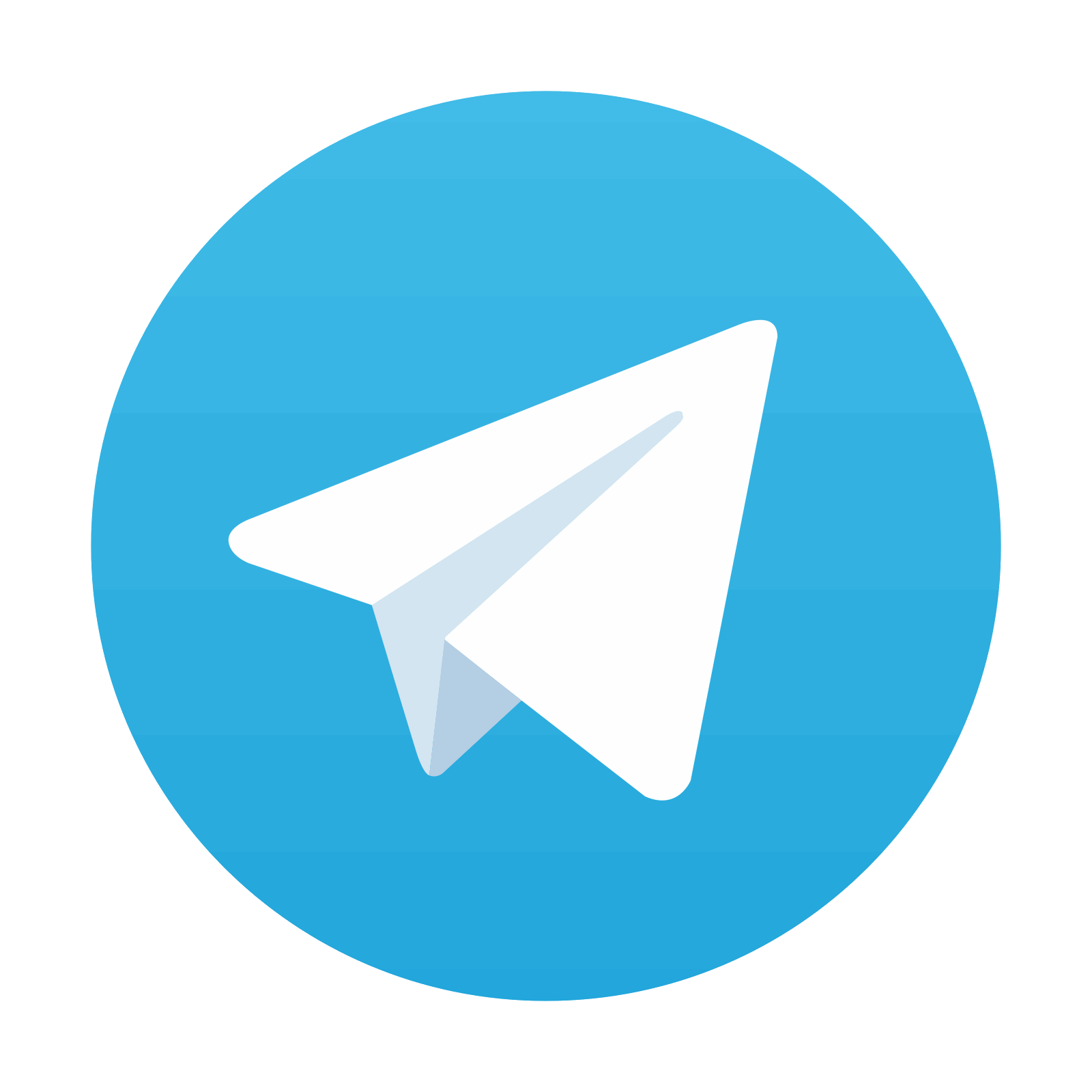
Stay updated, free articles. Join our Telegram channel

Full access? Get Clinical Tree
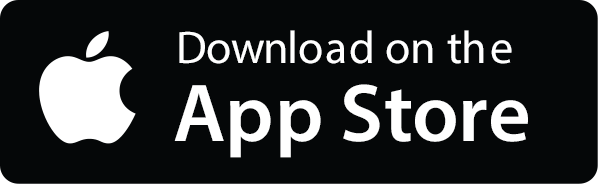
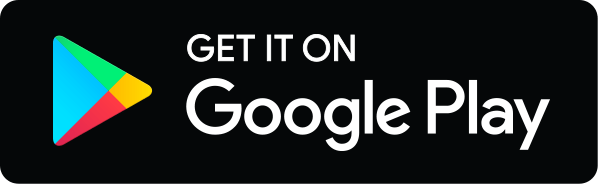