Abbreviations
cABC I
chondroitinase ABC I
CNS
central nervous system
CS
chondroitin sulfate
CSPG
chondroitin sulfate proteoglycan
CSPGs
chondroitin sulfate proteoglycans
DS
dermatan sulfate
DSPG
dermatan sulfate proteoglycan
ECM
extracellular matrix
GAGs
glycosaminoglycans
GBM
glioblastoma
HA
hyaluronic acid
Hep
heparin
HepS
heparan sulfate
HS
heparin sulfate
KS
keratan sulfate
PEG
polyethylene glycol (PEG)
SCI
spinal cord injury
Molecular perspectives
Glycosaminoglycans and proteoglycans
Extracellular matrices (ECMs) and cell surfaces of the mammalian tissues contain repeating disaccharide units known as glycosaminoglycans (GAGs). These linear polysaccharides can be sulfated in various positions to obtain a negatively charged nature that is essential for molecular recognition and binding. The corresponding reactions are catalyzed by the enzymes of the sulfotransferase family. Based on the type of disaccharide units, GAGs are categorized into several groups, including hyaluronic acid (HA), chondroitin sulfate/dermatan sulfate (CS/DS), heparin/heparin sulfate (Hep/HS), and keratan sulfate (KS) ( ).
A typical chondroitin sulfate disaccharide unit in human, chondroitin-6-sulfate (C6S) with the primary configuration containing a uronic acid (Glucuronic acid) and amino sugar (Galactosamine) is shown in Fig. 1 A . As shown in Fig. 1 B; GAG chains can covalently bind at their reducing ends to a serine or asparagine residue of a target protein, forming a proteoglycan ( ). On a higher level, a biopolymer such as hyaluronic acid acts as a backbone structure to assemble similar or various types of proteoglycans ( Fig. 1 C).

These macromolecules are synthesized in all mammalian cells and based on the type and function; they may be secreted into the ECM, localized into the plasma membrane, or stored in secretory vesicles ( ; ; ; ). Due to the wide variety of functions, any defects in the synthesis and processing of proteoglycans have important clinical manifestations ( ). Regarding the protein binding affinity and biocompatibility of GAGs, they are proposed as a tool for localized drug-delivery, using enzymes as drug ( ).
Distribution and functions of GAGs and proteoglycans
The GAGs play essential roles in fundamental biological processes, and wide distribution of these biopolymers in animals demonstrates that they have conserved functions in organisms ( ). In the normal state, individual tissues of the human body contain a defined combination of GAGs, some of which are tissue-specific, and others may be temporally observe during growth, development, and pathogenic conditions.
The ability to absorb water molecules and their spatial structure enables GAGs and proteoglycans to provide a suitable scaffold that is essential to maintain the shape, cohesive properties, and mechanical features of tissues ( ). Accordingly, in tissue-based diseases, damage to the structure of proteoglycan is a central event ( ). Some of the GAGs can bind into several specific proteins such as cytokines, chemokines, growth factors, morphogens, etc., to form biologically effective types of macromolecules ( ). These interactions are crucial in regulating of some critical biological processes, including cell migration ( ), regulation of blood coagulation and homeostasis ( ; ), growth factor control ( ), cell adhesion, and signaling ( ), inflammation ( ), and pathogens attachment ( ). They are involved in multicellular processes and have critical roles in development.
The GAGs are also involved in the inhibition of smooth muscle cell proliferation ( ). It has been reported that proteoglycans may have essential roles in normal glomerular cell function and progressive renal disease ( ). They are considered as the first defensive line at the bladder’s luminal surface against urinary tract infections, that created by pathogenic Escherichia coli among postmenopausal women ( ). It has postulated that the accumulation of specific GAGs may form a physical barrier to the passage of immunocompetent cells from the mother to the fetus ( ). The changes in the composition and molecular structure of placental GAGs during pregnancy could alter the molecular transport rate through placental connective tissue and affect the rate of fetal growth ( ). It has been reported that GAGs are involved in tumor development, angiogenesis and metastasis in certain types of cancers ( ).
CSPGs in the nervous system
It has revealed that CSPGs and DSPGs are dominant proteoglycans in CNS-ECM ( ). Chondroitin sulfate proteoglycans (CSPGs) are those CS-GAGs that are covalently linked to a core protein ( ). The GAG side chains in CSPGs are of different lengths, and the disaccharide units of CSPGs have either N -acetylglucosamine or N -acetylgalactosamine as well as uronic acid (see Fig. 1 ). Hence, depending on the length and the type of disaccharide unit and the position of sulfate moiety (positions 4 or 6), different types of CSPGs have identified, some of them have important tasks in CNS ( ). In CNS-ECM, various types of CSPGs bind to linear non-sulfated polysaccharides such as hyaluronan to produce a specific combination of proteoglycans, which is collectively known as lectican family proteoglycans. They include Neurocan, Brevican, Aggrecan, Perlecan, Testican, Versican, and Biglycan ( ; ). Some of the GAGs are attached to the transmembrane proteins and form the transmembrane family of proteoglycans. A schematic representation of various proteoglycans in the cell surface and ECM of nervous tissues is provided in Fig. 2 ( ). Structurally, the CNS-ECM proteoglycans provide the viscoelastic properties, maintain ions and water content and normal osmotic pressure, and dictate proper tissue organization. It has shown that the development of the nervous system in mammals involves coordinated cellular interactions, such as patterning and routing of migrating neural cells, axon pathfinding, and synapse formation, that is due to the proper organization of the nervous ECM ( ; ). Hence, the time-dependent concentration of CSPGs relative to other adhesive matrix molecules at the various developmental stage, define the normal state of the CNS-ECM ( ).

Implication of CSPGs in pathogen conditions
It has been reported that CSPGs have implications in the processes of tumor metastasis by affecting endothelial migration and adhesion ( ). In the case of brain cancer, changing the pattern of interaction of CS with ligands, growth factor receptors, extracellular matrix components, and structural proteins is accompanied by the progress of Glioblastoma (GBM) ( ). It has also revealed that neurodegenerative disorders are related to defects in the synthesis/degradation of proteoglycans that affect the structural integrity of nervous tissues ( ). Genome-wide association studies have shown that mental disorders such as bipolar character and depression are related to unbalance in CNS-ECM constituents ( ; ).
One of the important clinical manifestations after SCI includes the rapid increasing of various types of CSPGs in ECM. It is believed that this event is a defensive mechanism that seals off the injury to prevent bacterial penetration. The corresponding injury response in damaged CNS is called reactive gliosis or glial scarring ( ). The glial scar also has other positive effects such as reducing the inflammation area, modulating immune activity, and helping to restore the blood–brain barrier ( ; ; ). However, despite these positive impacts, the glial scar as a physical barrier reveals a negative role in axonal regrowth, sprouting, and synaptic reorganization following SCI ( ; ). Production of CSPGs occurs after the cute phase in approximately 48 h following SCI ( ). It also prevents oligodendrocyte maturation and remyelination ( ). Accordingly, medical intervention to control the concentration of CSPGs and limit the scar size in CNS-ECM has become central to the treatment of spinal cord injuries, and management of the CSPGs concentration during this period can result in the successful treatment of SCI in subsequent phases. There are numerous reports that carbohydrate and proteoglycan degrading enzymes can be used as a therapeutic drug to prevent CSPGs chains from accumulating in the site of SCI ( ; ; ; ).
Glycosaminoglycan degrading enzymes: A brief description
Regarding the various functions of GAG-proteoglycans, it is evident that creating a delicate balance between the biosynthesis and degradation of heteropolysaccharides is crucial in maintaining the normal health conditions of tissues. As mentioned in Implication of CSPGs in pathogen conditions section, the formation of proteoglycan-rich glial scar around traumatic injury of SCI inhibits long-term axonal recovery predominantly by CSPGs moieties, and degrading enzymes is considered as therapeutic drugs to overcome this medicinal challenge. Two types of enzymes can depolymerize GAG chains including lyases (EC 4.2.2.-) and hydrolases (EC 3-2-1.-). The lyases degrade GAGs by eliminative cleavage, while hydrolases-based degradation is carried out by hydrolytic cleavage. Based on the position of the cutting site, the pattern of enzymatic action is exolytic or endolytic mode ( ) ( ). In vivo degradation of GAGs is carried out by corresponding hydrolases such as keratinase, hyaluronic acid hydrolase, and heparin hydrolase. The GAG degrading lyases have mainly bacterial sources, and are categorized into three main groups, including heparinases, chondroitinases, and hyaluronidases.
Structural features of chondroitinase ABC I
According to CAZy (Carbohydrate-Active enZYmes Database), the tertiary structure of cABC I include (α/α) 6 toroid + anti-parallel β-sheet. As shown in Fig. 3 , it is composed of three distinctive domains, including N- and C-terminals, that are symmetrically attached to the central (catalytic) domain ( ). The catalytic residues are located in the central domain, and include His-501, Tyr-508, Arg-560, and Glu-653 ( ). The peripheral domains are mainly made up of well-defined organized β-sheets, and the central domain has mainly helix conformation. The movement of N- and C-terminal domains around the catalytic region leads to the creation of open and closed conformation that is essential for the catalytic activity of the enzyme. The enzyme cleaves its substrates at the glycosidic bond via β-elimination of 1,4-hexosaminidic bonds to produce Δ4,5-unsaturated disaccharides, and a reducing end polysaccharide. Spectroscopically, product formation can be monitored as an increase in absorbance at 232 nm as a function of time.

Chondroitinase ABC I and spinal cord injury
By convention, A, B, and C in chondroitinase ABC (cABC) nomenclature, refers to chondroitin 4-sulfate, dermatan sulfate, and chondroitin 6-sulfate, respectively. According to BRENDA—The Comprehensive Enzyme Information database ( www.brenda-enzymes.org ), cABC I from Proteus vulgaris (EC 4.2.2.20) is a broad specific endolytic enzyme that degrades a variety of glycosaminoglycans of the chondroitin-sulfate- and dermatan-sulfate type. It can also acts on hyaluronan at a much lower rate. Fortunately, these substrates are the main constituents of CNS GAGs that are formed by activated astrocytes following SCI. Hence, the broad specificity of cABC I toward a combination of GAGs can be considered as a positive feature to degrade the GAG chains and can restore the right condition for nerve regeneration following SCI.
In vivo delivery of cABC I to reduce the size of glial scar and removing the inhibitory environment for the treatment of SCI was first reported by Lemons et al. in 1999 ( ). Four years later, research groups from the University of Cambridge and the University of London delivered cABC to the lesioned dorsal columns of adult rats and observed a marked functional recovery of injured CNS ( ). To analyze the effects of cABC on axon regeneration, Lin and co-workers made unilateral nigrostriatal lesions in adult rats and found that injection of cABC can digest hyaluronan and reduce neurocan in a limited area around the injection region. At the same time, the majority of GAGs are inaccessible to cABC digestion. They suggest that a single injection of cABC can produce an environment conducive to CNS repair for a long-term over 10 days ( ). In 2011, Fawcett et al. showed that using cABC combined with rehabilitation is effective in functional recovery of forelimb function in rats with chronic SCI. They used cABC I 4 weeks after SCI ( ). These studies, together with other investigations, have confirmed the ability of cABC I to help axon regeneration following SCI ( ; ).
Chondroitinase ABC I for enzyme therapy: Challenges and perspectives
Proteus Vulgaris as the bacterial source of cABC I presents in soil and intestinal tract of the human body. It uses acidic polysaccharides along with epithelial cells in the intestinal lumen as its nutrient source. Accordingly, its optimum temperature for the activity is in accordance with the normal temperature of the human body. However, the time-dependent concentration of cABC I in P. vulgaris is low, which is essential for its solubility. In other words, protein aggregation is an entropy-dependent process, and increasing the concentration of the enzyme molecules for practical uses, particularly at 37 °C, leads to protein aggregation due to the higher probability of effective molecular collisions ( ). Additionally, the enzyme adaptation has been occurred not only on the temperature but also on the other environmental factors of P. vulgaris such as pH and ionic strengths that are different from those found in the new therapeutic environment. Accordingly, changing the environmental conditions for the enzyme is accompanied by decreasing its half-life and lower catalytic efficiency due to its low conformational stability. So, more concentration of the enzyme is needed to have efficient catalysis. Nonetheless, as mentioned above, there is a critical concentration of the enzyme above that it undergoes molecular aggregation. Moreover, using a high concentration of the recombinant enzyme per injection or increasing the number of enzyme injections at the site of SCI for more efficient catalysis may have immunogenicity responses ( ). Considering the instability of cABC I due to protein aggregation and decreased half-life as well as medical challenges following repeatedly administer of the recombinant enzyme, various enzyme stabilization and enzyme delivery methods, should be developed to efficient use of the enzyme for SCI treatment. Furthermore, the formulation of the enzyme appropriate for long-term storage permits the clinicians to access the enzyme in the shortest time and a usable form.
Protein engineering strategies toward functional drug delivery
The enzyme can be synthesized, extracted, and purified with the enzyme technology methods. The classical procedures for enhancing the enzyme stability involve setting up right environmental conditions using suitable solvents containing various types of osmolytes and additives as stabilizers. It was shown that chemical modification of the recombinant enzymes with Polyethylene glycol (PEG) could increase the protein half-life and reduces the possibility of immunological side effects due to its bacterial origin ( ). Additionally, site-directed mutagenesis has intensively used to logically replace critical amino acids on the structure of the protein with new residues to improve the functional and structural features of the enzyme. For example, it was revealed that increasing the repulsive electrostatic interactions between enzyme molecules via changing of surface-exposed residues by site-directed mutagenesis can reduce aggregation ( ). Shoichet et al. used PEG-chemical modification procedure and site-directed mutagenesis strategy to produce improved engineered enzyme for SCI treatment. They demonstrated that representative PEGylated mutants of cABC could be locally delivered at the site of SCI in a more stabilized form ( ). Drug nano-carriers such as nano-structured lipid carriers (NLCs) are also potential candidates for the efficiently deliver of the enzyme toward its target. Enzyme immobilization using biocompatible nano-structures can protect the enzyme molecules from biodegradation and leads to the gradual release of the enzyme ( ; ). These are only examples of ongoing research studies on the humanization and methodological setting for using cABC I as a drug. Details of such studies have been reviewed elsewhere ( ; ).
Combinatorial therapy with chondroitinase ABC I
It is worth mentioning that SCI is accompanied by several events including proliferation of astrocytes, accumulation of macrophages and microglia, production of growth-inhibitory matrix around SCI region, abnormality in signal transduction, etc. These phenomena result in multiple clinical consequences. Therefore, SCI should be simultaneously targeted with various medical interventions ( ). In other words, cABC I should be used besides other therapeutic agents to achieve a satisfactory outcome. Efficient delivery of cABC as a drug requires the determination of the therapeutic window of enzyme utilization during treatment time. As well as timing, the threshold of enzyme concentration influences the efficacy of enzyme therapy. It was shown that the expression of corresponding proteins increases following SCI within the first 24 h, and the maximum level of CSPGs is generally observed approximately 2 weeks after SCI. However, the gradual increase of CSPGs over time is occurred with different rates according to the type of proteoglycan ( ). Finally, locomotor recovery depends on optimized drug-based treatments combined with rehabilitation.
Applications to other areas of neuroscience
Regarding the diverse roles of CSPGs in the physiological and pathological conditions of the CNS-ECM, cABC I can be used to control the right composition of the CSPGs according to the abnormal states of the nervous tissue. It had demonstrated that the efficiency of nerve recovery through peripheral nerve graft (PNG) increased in combined with cABC I and acidic fibroblast growth factor usage ( ). The enzyme can also be used to eliminate the inhibitory function of CSPGs on the remyelination, which is essential in the treatment of multiple sclerosis ( ). Investigating the experimental models has revealed that cABC I offers the opportunity to improve the outcome of Alzheimer’s disease treatment via restoration of extracellular matrix plasticity in the synaptic region of hippocampal ( ). It would be beneficial in the case of Parkinson’s disease and for patients that are involved in phobias and anxiety ( ; ). There are pieces of evidence that cABC I is helpful in the treatment of glioblastoma through sensitizing the glioblastoma cells to cancer drugs and enhancing the spread of oncolytic viruses as well as changing the pattern of the interaction of CSPGs with specific proteins ( ; ; ).
Mini-dictionary of terms
Glycosaminoglycan: Linear polysaccharide made up of repeating disaccharide units. Based on the type of sugar building blocks, various glycosaminoglycans are found in extracellular matrices of tissues.
Proteoglycan: A highly glycosylated protein produced by attachment of a given glycosaminoglycan to a given target protein. A combination of various types of proteoglycans is found in extracellular matrices. The time-dependent concentration of proteoglycans is changed due to the developmental stage or pathogenic conditions of the cells and tissues.
Chondroitin sulfate proteoglycans: Specific types of proteoglycans that are sulfated in representative positions of their sugar moiety. They are one of the main constituents of the extracellular matrix of the nervous system.
CAZy: stands for “Carbohydrate-Active enZYmes Database.” It is the specialized database that describes the families of structurally-related enzymes and carbohydrate-binding modules that are involved in the breakdown, biosynthesis, or modification of carbohydrates and glycoconjugates. It can be found at http://www.cazy.org/
BRENDA: It is a bioinformatics database containing the functional and structural information of the known enzymes. It can be found at www.brenda-enzymes.org
Glioblastoma: A type of cancer originated from the proliferation of astrocytes in the brain or spinal cord.
Conformational stability: In statistical thermodynamics, the conformational stability of a given enzyme refers to the ratio of the population of the native structure to denature states of the enzyme molecules at equilibrium condition. When a single molecule is considered, it is the structural integrity of an enzyme molecule that is the difference energy of the unfolded and folded states of an enzyme molecule. In both paradigms, increasing conformational stability leads to the activity improvement of the enzyme.
Catalytic efficiency: The minimum concentration of the substrate molecule required for an enzyme to reach its maximum catalysis capacity.
Half-life: The time required for an enzyme to lose 50% of its original activity. Increasing the conformational stability leads to increasing the half-life of an enzyme.
Protein Aggregation: is a process in which protein molecules accumulate and form molecular aggregates. Upon aggregation, the protein loses its activity. It occurred by attractive intermolecular interactions, mainly the hydrophobic one, between surface-exposed hydrophobic patches. Based on the identity of hydrophobic interaction, protein aggregation depends on the molecular collisions, which enhance by increasing the temperature and the concentration of the protein molecule. Proteins with surfaced-exposed hydrophobic patches are more prone to aggregation.
Domain: In protein science, domain is defined as distinct structural unite in the three dimensional structure of proteins. In the simplest form, the whole structure of a small and compact protein is considered as a domain. Some proteins have two or more domains. Domains are responsible for the structural integrity and stability of proteins. They are also involved in the functional features of the proteins.
Recombinant DNA technology: Recombinant DNA technology includes insertion of a given DNA sequence from an organism to the other one. In protein engineering studies, the host organism may be an engineered bacterium that is used for the expression of a recombinant protein which is of value to biotechnology.
Key facts of chondroitinase ABC I
Chondroitinase ABC I is a non-hydrolytic degrading enzyme that cleaves the glycosidic bonds of polysaccharides.
- •
It has three distinctive domains, where the peripheral ones are symmetrically arranged around the central catalytic domain.
- •
Naturally, this enzyme is expressed in Proteus Vulgaris . This bacterium is found in soil and intestinal tract of the human body.
- •
The enzyme and its mutated variants can be produced by recombinant DNA technology, and using engineered bacteria as protein express systems.
- •
Chondroitinase ABC I as a polysaccharide lyase has broad substrate specificity.
- •
Its substrates constitute the main glycosaminoglycans of extracellular matrix of the central nervous system.
Key facts of the therapeutic potential of chondroitinase ABC I
- •
The concentration of corresponding substrates of chondroitinase ABC I increases in the extracellular matrix upon spinal cord injury, which acts as inhibitors against axon regeneration.
- •
The enzyme can be used to degrade the glycosaminoglycans around the traumatic region of the spinal cord.
- •
Its conformational stability and half-life reduce in the environmental conditions around the region of SCI.
- •
To use as a drug, the conformational stability, and biocompatibility of the enzyme should be increased via protein engineering strategies.
- •
Appropriate drug delivery methods should be developed to efficient use of chondroitinase ABC I at the site of spinal cord damage.
Summary points
- •
The extracellular matrix of the central nervous system contains various types of glycosaminoglycans in the form of proteoglycans.
- •
A fine balance between the concentrations of different proteoglycans is established in normal physiological conditions.
- •
Glial scar is proposed as the main inhibitor for axon regeneration following spinal cord injury.
- •
Glial scar is composed of various types of concentrated glycosaminoglycans in the region of spinal cord damage.
- •
Degradation of glycosaminoglycans at the site of spinal cord damage combined with other therapeutic strategies can help axons to recover.
- •
The broad substrate specificity of cABC I in accord with the composition of glial scar makes the enzyme a suitable therapeutic drug to restore tissue plasticity and axon regeneration.
- •
Using a high concentration of the enzyme per injection leads to molecular aggregation.
- •
Increasing the number of injection of the enzyme may results in immunological side effects.
- •
Protein engineering and drug delivery procedures are needed to produce, store, and therapeutically use of chondroitinase ABC I.
- •
Enzyme therapy should be used in predefined periods of time.
- •
Combinatorial approaches and other medical interventions along with intensive rehabilitation are being developed for locomotor recovery.
References
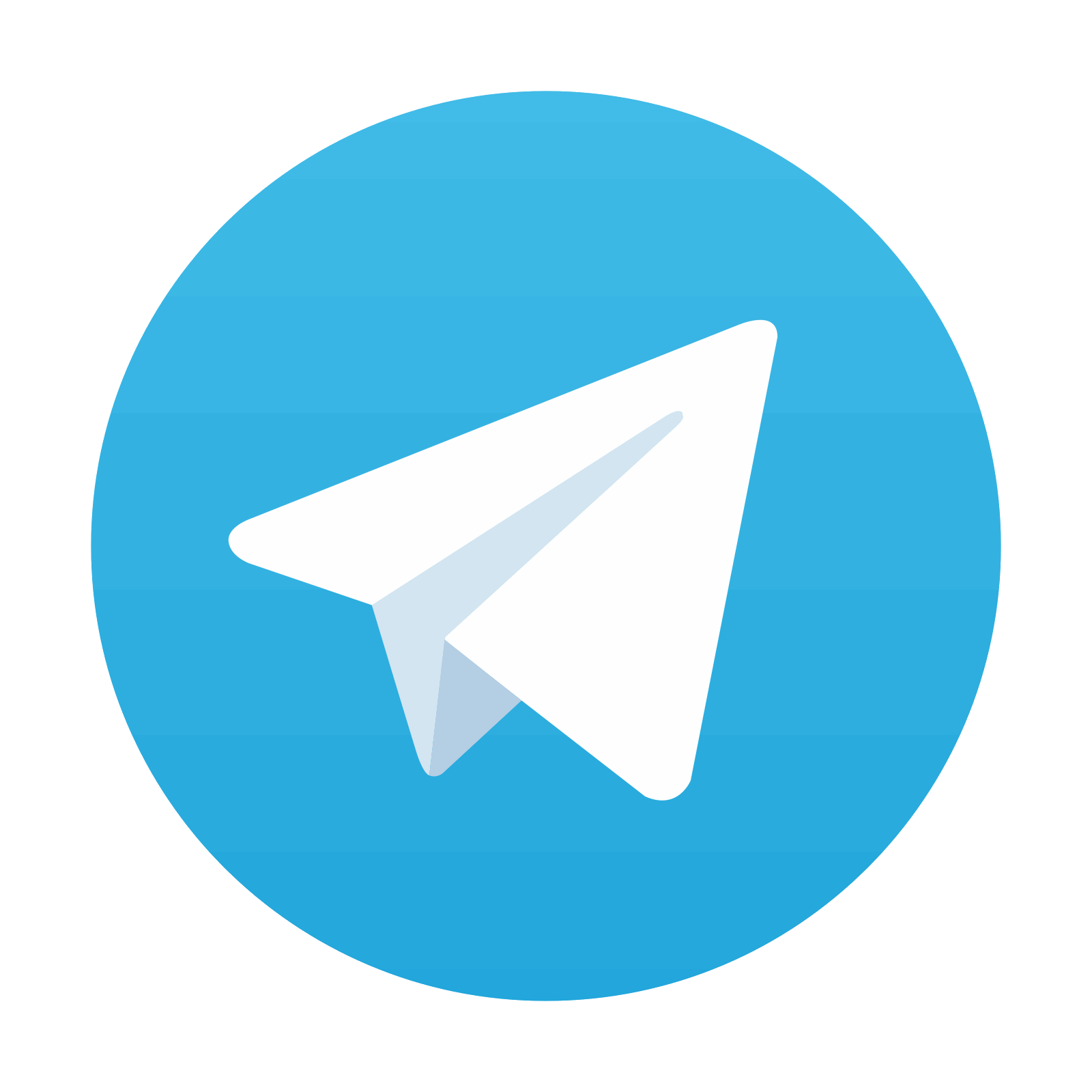
Stay updated, free articles. Join our Telegram channel

Full access? Get Clinical Tree
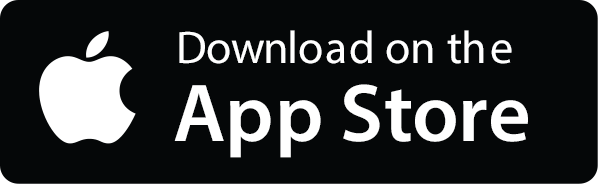
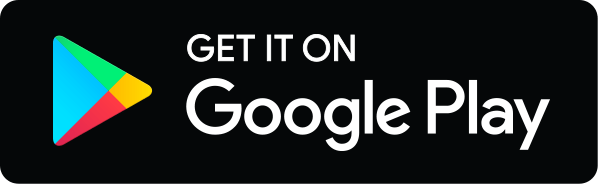
