Fig. 13.1
Subjective responses in healthy subjects caused by congruent or incongruent bimanual movements in front of a mirror or a whiteboard [106]. (a) Frequency of responses during hand movements which are either congruent or incongruent, either in front of a mirror or a whiteboard. Bars indicate the absolute number of responses in N = 113 participants. (b) Perceived location of a third hand in those participants who report an extra limb (reference points: shoulder, elbow, palm of the hand) in the incongruent mirror condition. Whiskers indicate the standard errors of the mean. While the mirror was placed in such a manner that the visible location of the mirrored hand matches that of the actual hand behind the mirror, these data indicate that the extra hand is perceived to be significantly closer to the midline of the body
In healthy subjects, the observation of one’s own body parts receiving acute painful stimuli results in a decrease both in subjective pain ratings and physiological responses of the central nervous system [107, 108]. This effect did not occur when a non-corporeal object was used, indicating a strong visually induced analgesia by observing one’s own body. In acute pain, optical enlargement of a body part was associated with an increased visually induced analgesia, whereas the illusion of a shrunken body part had the opposite effect and decreased pain perception [109]. However, there are also reports for an opposite effect in chronic pain patients. Wearing magnifying binoculars while looking at the actively moving limb increased the perceived pain evoked by movements in CRPS patients, whereas minimizing binoculars reduced the intensity of pain [110], and the use of size-changing lenses also reduced pain intensity in PLP patients [111]. There might be multiple or different interactions between vision and pain: as described earlier, visual enlargement of a body part in healthy participants increased visually induced analgesia [109], whereas a similar procedure in chronic pain patients enhances the pain [110, 111]. These two conflicting processes related to the interaction of vision and pain should be considered when altered visual feedback is used in pain patients.
The properties of the neuronal system might be a promising starting point for using the beneficial interactions between pain perception and the other sensory domains in the treatment of chronic pain. Functional neuroimaging studies suggested that the activity in cortical areas during perception experiments reflects the subjective experience rather than the physical stimulus characteristics [112, 113]. Due to the close relationship between nociception and higher-order body experiences, the use of bodily illusions to adjust altered body perceptions thus might be a powerful tool for treating chronic pain [114]. In the next section we will explain how bodily illusions could be used to manipulate the experience of self in chronic pain patients and which stimulation conditions they have to fulfil.
13.5 Bodily Illusions and Implications for Their Use to Treat Chronic Pain
The evidence discussed above has led to the development of several innovative and promising non-pharmacological treatments, all of which include the notion of providing the brain with at least part of the sensory input that it would expect from a functioning limb [115] in PLP and by extension a functional body part in other types of chronic pain. The key for developing such a treatment is the focus on the alterations in body representation and perception.
There are multiple discernible elements of the perception of one’s own body [40], but perceived agency and ownership seem to play a crucial role [2, 116]. Both sensations contribute to the perception of self, but represent distinct entities [24]. The original RHI paradigm is a passive setup for the application of visuotactile stimulation creating a strong sensation of illusory ownership (e.g., [29, 39]). However, newer adaptations indicate that passive and specifically active movements that combine visuoproprioceptive input and motor action lead to higher intensities in illusory ownership than tactile stimulation alone [24, 117]. Thus, more intense sensations of ownership and agency in the RHI experiment are associated with mislocalization of one’s own limb position insofar as the actual hand is perceived at the location of the artificial limb [40, 118], indicating a shift in proprioceptive body coordinates by successful illusion induction [1].
To create the subjective feeling of ownership and agency, an illusion setup has to fulfil several requirements in the interactions between visual, proprioceptive, and tactile stimulation [1] as well as motor feedback. In amputees, mirroring the intact limb enhances phantom limb awareness [119], indicating that a match between visual (reflection of the intact limb) and proprioceptive input (proprioceptive awareness of the phantom) enhances the perception of self. Thus, illusory self experiences in healthy participants are induced much more easily when the external object resembles a real hand rather than a non-corporeal object [43, 120]. The same applies for proprioceptive features. In healthy subjects the sensation of illusory ownership seems to be reduced by an incongruent postural position of an artificial limb in comparison to one’s real hand [29, 43, 121, 122] or a larger distance between the real and the artificial hand [123]. Ultimately, illusory self experiences are more easily inducible by a high degree of temporal and spatial congruence between the seen and felt tactile stimulation of the artificial body part and the own body [29, 39, 40, 124–126]. In a similar manner, conflicting visual feedback of own movements reduced sensations of self-awareness [24].
Therefore, an artificial body part, which shall replace the actual one, must have maximum possible congruence with the perceived characteristics of the affected body part [1] or the phantom in case of limb amputation. For that reason, the unique sensations in chronic pain patients—such as deformations, stiffness, and postural distortions—might be an additional source of sensory incongruence and a barrier for inducing the sensation of ownership and agency for an artificial body part.
In the next two sections, we review the evidence for two promising treatment methods for reducing chronic pain. While the first one (mirrors and VR setups) might be useful for different kinds of pain syndromes, the second one (prostheses) is exclusively suitable for the treatment of postamputation pain. Nevertheless, both treatment approaches might share one crucial element: restoring normal body representation and perception.
13.5.1 Mirrors and Virtual Reality
Ramachandran et al. [127] showed that creating the illusion of two existing limbs by using a mirror box setup was sufficient to trigger congruent feelings in the phantom limb of amputees. Additionally, this setup enabled some patients to regain control over their phantom limb and—most importantly—alleviated painful phantom sensations in some [18, 128]. This anecdotal evidence led to the suggestion of using a mirror setup specifically for treatment of PLP and other chronic pain states. Several recent studies showed that this so-called mirror therapy had positive effects on PLP [15, 16, 129–131] and CRPS [132–134], where mirror training can enhance range of motion and reduce pain and swelling. Motor imagery might be an important influencing or additional factor for effectiveness of the mirror therapy and has by itself been shown to be an effective treatment method (e.g., [89], but see [15]).
Chan et al. [15] conducted a controlled crossover study to test the effectiveness of mirror therapy treatment. They found that a four-week training of movements in front of a mirror significantly reduced the PLP intensity in people with leg amputation, while executing movements without a mirror or motor imagery had no effect. A problem with this study is that it was conducted with recently amputated veterans of the Iraq war. Especially in the first two years after amputation, however, the experience of phantom phenomena and PLP may still change. Moreover, the study had no long-term follow-up.
Foell et al. [16] evaluated the effectiveness of mirror therapy for chronic PLP patients who were on the average more than 20 years after amputation. In this study, the authors examined 13 unilateral upper limb amputees suffering from PLP, 11 of which also participated in a magnetic resonance imaging session immediately before and after treatment to determine the representation of the lip (which is located adjacent to the missing limb and indicative of dysfunctional reorganization) in primary somatosensory cortex. Eight subjects reported a telescopic distortion of their phantom limbs. The participants performed movements with the intact limb in front of mirror for four weeks, 15 min per day. They indicated the daily intensity of their PLP by using a visual analog scale ranging from 0 (no pain) to 100 (very intense pain). In addition, daily PLP intensity was assessed two weeks before (baseline) and after training.Across entire sample Foell et al. [16] observed a reduction in PLP intensity of 27 % over the duration of the mirror training. The dysfunctional cortical shift of the lip representation decreased over this period by about three millimeters. Interestingly, there was a strong correlation between the alterations in the experience of pain and changes in cortical reorganization (Fig. 13.2). The more the subjects benefited from the therapy, the more their cortical representation of the lip had normalized. However, a majority of subjects did not or only slightly profit from the treatment. The authors found that the ability to relate the mirrored image to the phantom significantly predicted the effect size of the therapy. Further, the presence of a telescopic distortion of the phantom was a strong predictor for treatment efficacy (Fig. 13.2). The overall efficacy of the treatment depended almost exclusively on those persons who did not report a telescopic distortion of their phantoms. Members of this subgroup showed a mean reduction of PLP of more than 50 %, while subjects with a telescopic distortion reported a mean change in their PLP intensity of less than one percent. This result emphasizes the importance of body perception and the integration of sensory input for this kind of therapy, which was emphasized by the significant positive relationship between pain reduction after treatment and a decrease of activity in inferior parietal cortex, a region involved in the processing of painful input [135–137] as well as the experience of agency [138, 139].
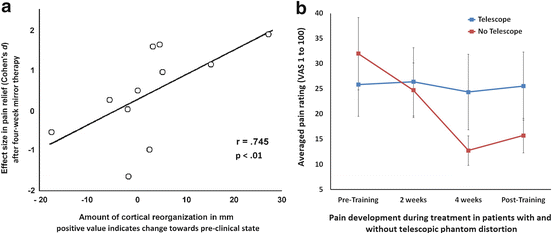
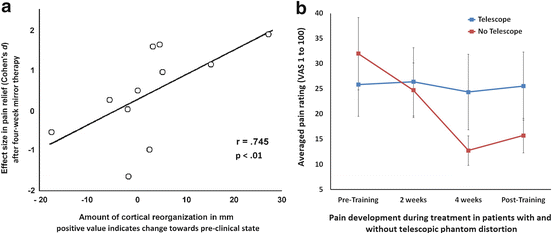
Fig. 13.2
Mirror therapy for chronic phantom limb pain [16]. (a) Correlation between cortical reorganization in primary somatosensory cortex and treatment effect after a four-week mirror training. Cortical reorganization was assessed by comparing locations of primary somatosensory lip representations between hemispheres. (b) Effect of mirror training on patients with (blue line) and without (red line) telescopic distortion of the phantom limb. Averaged pain ratings for two weeks prior to training, first two weeks of training, second two weeks of training, and two weeks without treatment after conclusion of training. Error bars indicate the standard errors of the mean
Healthy people can easily test the plasticity of their body perception by placing their outstretched arms in front of and behind a mirror and starting to open and close their hands slowly. If one observes the mirrored hand movements for a few seconds, in most people the sensation quickly arises as if they were no longer looking at a reflection, but directly on their hidden hand, as if they would look through a glass panel. This so-called mirror illusion illustrates the principle of sensory integration of body-related stimuli [55, 56]. A person sees a hand at the position where she or he actually feels the hidden hand, and the seen movements also match the executed and perceived movements of the hidden hand. The most plausible interpretation of these sensory impressions is that the hand the subject visually perceives is her or his real hidden hand. However, flexing the hidden arm’s elbow (i.e., simulating a telescoping distortion) usually prevents illusion induction. The brain appears to register that what one sees (an outstretched arm) does not fit to what one feels (a flexed, shortened arm). This sensory conflict might be responsible for the marginal effects of mirror therapy in amputees perceiving a telescope. The brain might not be able to combine visual and somatosensory input, which might be a requisite for reactivation of the former hand representation in upper limb amputees [75]. This might be a common problem in the application of mirror therapy for the treatment of pain.
Previous studies reported a large variance for the effectiveness of mirror therapy [15, 127, 140, 141]. In addition to difficulties in the alignment of visual and somatosensory input in the case of distortions of the affected body part [16], people might also differ in their ability to integrate body-related sensory information of different modalities [44]. This suggests that the attempts to reduce sensory incongruence may be the most important challenge for mirror treatment applications. Some problems could probably be solved by a suitable implementation and instruction [cf. 142], but previous results suggest that a voluntary movement [143, 144] or at least a movement intent [145] must be generated for the intact mirrored limb to produce an effective response in motor and somatosensory cortex representing the affected limb. The responsiveness of the motor cortex contralateral to the affected limb, however, appears to be differentially impaired in amputees [75]. By mirroring the movements of the intact hand, Diers et al.[7] showed that cortical responsiveness contralateral to the phantom hand is significantly reduced in amputees experiencing PLP, whereas healthy controls and amputees without PLP showed bilateral activity in sensorimotor cortex. These functional alterations might complicate the use of mirror therapy in some patients.
The use of advanced VR setups might solve some of the problems related to mirror therapy. In an immersive virtual reality (IVR) environment, healthy subjects perceive modelled upper limbs [126, 146–149] or even entire bodies [150, 151] as their own. Thus, a good alternative to training programs using mirrors or videos might be VR setups in which the user can freely adjust a wide variety of features such as the appearance and responsiveness of the limb or properties of the task or the environment. The idea is similar to that of mirror therapy: a virtual image of an unaffected body part should visually replace an affected body part in order to correct body integrity and perception.
For this reason, current efforts aim at creating an IVR environment in which an artificial functional limb can be simulated. By using IVR setups recent studies have shown that this might be a promising alternative to mirror treatment in PLP [20, 131, 152] and CRPS [153], and others have suggested a promising application in fibromyalgia [154]. There are several advantages of using virtual simulated limbs rather than mirrors. On the one hand, IVR devices might be useful in cases of bilateral amputations, where mirror treatment is not possible or in the case of deformations of the unaffected limb, which might reduce the benefit of such a therapy [155]. Additionally, this approach allows for adaptations of the perceived characteristics of the affected limb: if, for example, a patient suffering from PLP after amputation experiences a severe telescope, as mentioned above, a mirrored reflection of the non-affected moving arm might be too different in visual properties to match vision and somatosensation [16]. In a modified RHI paradigm, amputees experiencing a telescoped phantom tended to report fewer sensations of replacing incorporation of the artificial limb than patients without telescoped phantoms [156]. Instead, there are sporadic reports of an additional phantom limb, indicating some difficulties in combining visual and proprioceptive input similar to results in healthy participants [16]. We suggest that a visually adjusted limb in an IVR environment might be more adequate in the beginning of the therapy in case of distorted phantom experiences. In a similar fashion, IVR setups might be useful to treat other chronic pain syndromes by adapting the virtual body parts to the distorted experiences of the patients [3, 4] in order to increase the identification of the artificial counterpart at the beginning of treatment as well as the probably associated visually induced analgesia effect on chronic pain [19].
13.5.2 Prostheses
Especially in amputees, another realization of the concept of simulating the self is feasible: the use of prosthetic devices. Cosmetic prostheses recreate the lost limb visually and can be used as a support in several tasks, while myoelectric prostheses allow the user to control movements such as grasping or flexion of the elbow or knee by giving muscle commands. Based on the results and proposed mechanisms of mirror therapy described above, it could be argued that a controllable prosthesis is conceptually similar to a controllable mirror image of the intact limb, in that it can create sensations of agency and ownership. Thus, McDonnel et al. [157] suggested that manipulating the sense of self might be useful by asking, “Do artificial limbs become part of the user?” They found that the usage of a prosthesis leads to an overestimation of the length of the stump, and other studies found a positive relationship between prosthesis use and vividness of phantom limb awareness [158], indicating that the prosthesis altered the body perception in higher-order brain areas and might therefore prevent the development of PLP by the inclusion of residual motor and sensory nerve fibers within the residual limb. In cross-sectional studies, the use of myoelectric or other functional prostheses—in contrast to nonfunctional devices—was negatively correlated with the perceived level of PLP as well as dysfunctional cortical reorganization [22, 23]. Ownership and agency with respect to the prosthetic device seem to enhance the patient’s ability to accept this external object as a part of the body [159], probably depending on the temporal and spatial congruence between the visual and proprioceptive modality. If there is too much discordance between the senses, this incongruence will lead the patients to reject the prosthesis as a part of their body, which could explain the considerable number of prostheses rejecters [160]. Furthermore, recent results highlight the importance of congruent tactile feedback between seen and felt touch, since amputees can be induced to perceive a rubber hand [161] as well as a prosthetic device [162, 163] as belonging to their body by synchronous tactile stimulation of the artificial and the residual limb. The neuronal mechanisms of limb ownership experiences in non-amputees and amputees seem to be actually similar [164], highlighting the high degree of flexibility of body representation and perception.
This is of special importance, since ownership experiences for prosthetic devices are negatively correlated to the experience of PLP [9, 74] and might change the way how the body is neuronally represented. Brozzoli et al. [165] showed that the representation of peripersonal space in humans is centered on the limb and—as mentioned earlier—processed by a widely distributed frontoparietal network. Using the RHI paradigm in healthy volunteers, they showed that there are similarities between neuronal activation patterns in response to an object approaching the participant’s own hand as well as an artificial limb which is perceived as belonging to the participant’s body. Consequently, peripersonal space processing in humans does not seem to be centered at the physical body but rather at the locus of perceived body ownership. Thus, the results indicate that prosthetic devices perceived as belonging to the amputee’s body might be represented in a different manner than prostheses perceived as mere tools and foreign to the body [166] which might be a crucial factor in using the prosthesis in everyday life. It might be that the experience of ownership for a prosthesis might activate the “abandoned” neuronal representation of the missing limb through input of association areas of the brain, simulating the absent sensory influx of the former limb.
The important findings about positive effects of prosthesis use in previous studies underline current efforts to develop more effective artificial limbs which use sophisticated mechanic and electronic systems to achieve improved controllability. One current approach is the improvement of prosthesis controllability using biorobotics. In a recent proof-of-concept study [167], upper limb amputees were instructed to mentally perform movements with the missing limb. These movement commands were recorded using electrodes implanted directly into the patients’ median and ulnar nerves and could be used to control a robotic limb. Additionally, current trends in prosthesis development—such as osseointegration [168], targeted muscle reinnervation [169], and brain-machine interfaces [170]—reflect powerful innovations in restoring the body integrity in limb amputees.
In a similar fashion, recent approaches aim at collecting tactile information via sensors in the artificial fingertips and to relay this information to the residual limb using tactile or electrical stimulation [171–173], which is assumed to facilitate the incorporation of the prosthesis into the body representation [172]. Thus, in addition to the incorporation of a lifelike prosthesis, another critical starting point for preventing chronic pain can be combined: tactile discrimination, which seems to be deficient in body parts affected by chronic pain [174]. Since previous studies could show that implementing an active tactile discrimination training reverses dysfunctional cortical reorganization and decreases PLP [175], other studies investigated these effects on other pain syndromes such as CRPS and found similar results [176]. Interestingly, the positive effect of a tactile discrimination training is enhanced when chronic pain patients watch the reflected image of their unaffected limb during training [177], suggesting that in amputees a combination of a lifelike functional prosthesis in combination with VR and tactile feedback might be the best treatment for chronic PLP or even its prevention.
13.6 Conclusion
In this chapter we suggested that approaches in treating chronic pain syndromes by influencing the distorted body representation share a common element, namely creating an illusory perception of self for an artificial body part, be it by using mirrors, VR, or prosthetic devices. The neuronal correlate of the mechanism of mirror treatments might be a combination of analgesic effects due to vision [19, 107, 108], but might also be a correction of neuronal incongruence between the visual and somatosensory system [75, 98, 178]. As in actual body part perception [e.g., 26, 34], agency and ownership concerning an artificial limb are represented and maintained in a neuronal network including premotor [1, 29–31, 165], occipital [162], and parietal cortex [1, 29–31, 51]. Most importantly, the parietal cortex in general and the somatosensory cortex in particular show functional [76, 80, 81, 83, 85, 91] alterations associated with chronic pain, indicating that the distorted perception of self might have its origins in these regions. Recent findings suggest that inducing bodily illusions might adjust the distorted body representation via the multimodal perception of the external environment. However, the perception of self through the experience of ownership and agency seems to require certain conditions regarding the sensory modalities, such as visual, proprioceptive, tactile, and motor congruency. Prospective investigation has to focus on individual capabilities to apply certain methods for adjusting the distorted body representation as well as the neurocognitive processes which are responsible for their effectiveness.
References
1.
Tsakiris M (2010) My body in the brain: a neurocognitive model of body-ownership. Neuropsychologia 48:703–712PubMed
2.
Synofzik M, Vosgerau G, Newen A (2008) I move, therefore I am: a new theoretical framework to investigate agency and ownership. Conscious Cogn 17:411–424PubMed
3.
Lotze M, Moseley GL (2007) Role of distorted body image in pain. Curr Rheumatol Rep 9:488–496PubMed
4.
Schwoebel J, Friedman R, Duda N et al (2001) Pain and the body schema: evidence for peripheral effects on mental representations of movement. Brain 124:2098–2104PubMed
5.
Förderreuther S, Sailer U, Straube A (2004) Impaired self-perception of the hand in complex regional pain syndrome (CRPS). Pain 110:756–761PubMed
6.
Ehde DM, Czerniecki JM, Smith DG et al (2000) Chronic phantom sensations, phantom pain, residual limb pain, and other regional pain after lower limb amputation. Arch Phys Med Rehabil 81:1039–1044PubMed
7.
Ephraim PL, Wegener ST, MacKenzie EJ et al (2005) Phantom pain, residual limb pain, and back pain in amputees: results of a national survey. Arch Phys Med Rehabil 86:1910–1919PubMed
8.
Hanley MA, Ehde DM, Jensen M et al (2009) Chronic pain associated with upper-limb loss. Am J Phys Med Rehabil 88:742–751PubMedCentralPubMed
9.
Kern U, Busch V, Rockland M et al (2009) Prevalence and risk factors of phantom limb pain and phantom limb sensations in Germany. A nationwide field survey. Schmerz 23:479–488PubMed
10.
Kooijman CM, Dijkstra PU, Geertzen JH et al (2000) Phantom pain and phantom sensations in upper limb amputees: an epidemiological study. Pain 87:33–41PubMed
11.
Sherman RA, Sherman CJ (1983) Prevalence and characteristics of chronic phantom limb pain among American veterans. Results of a trial survey. Am J Phys Med 62:227–238PubMed
12.
Wartan SW, Hamann W, Wedley JR et al (1997) Phantom pain and sensation among British veteran amputees. Br J Anaesth 78:652–659PubMed
13.
Altschuler EL, Hu J (2008) Mirror therapy in a patient with a fractured wrist and no active wrist extension. Scand J Plast Reconstr Surg Hand Surg 42:110–111PubMed
14.
Cacchio A, De Blasis E, De Blasis V et al (2009) Mirror therapy in complex regional pain syndrome type 1 of the upper limb in stroke patients. Neurorehabil Neural Repair 23:792–799PubMed
15.
Chan BL, Witt R, Charrow AP et al (2007) Mirror therapy for phantom limb pain. N Engl J Med 357:2206–2207PubMed
16.
Foell J, Bekrater-Bodmann R, Diers M et al (2014) Mirror therapy for phantom limb pain: brain changes and the role of body representation. Eur J Pain 18:729–739PubMed
17.
Michielsen ME, Smits M, Ribbers GM et al (2011) The neuronal correlates of mirror therapy: an fMRI study on mirror induced visual illusions in patients with stroke. J Neurol Neurosurg Psychiatry 82:393–398PubMed
18.
Ramachandran VS, Rogers-Ramachandran D (1996) Synaesthesia in phantom limbs induced with mirrors. Proc Biol Sci 263:377–386PubMed
19.
Diers M, Zieglgänsberger W, Trojan J et al (2013) Site-specific visual feedback reduces pain perception. Pain 154:890–896PubMed
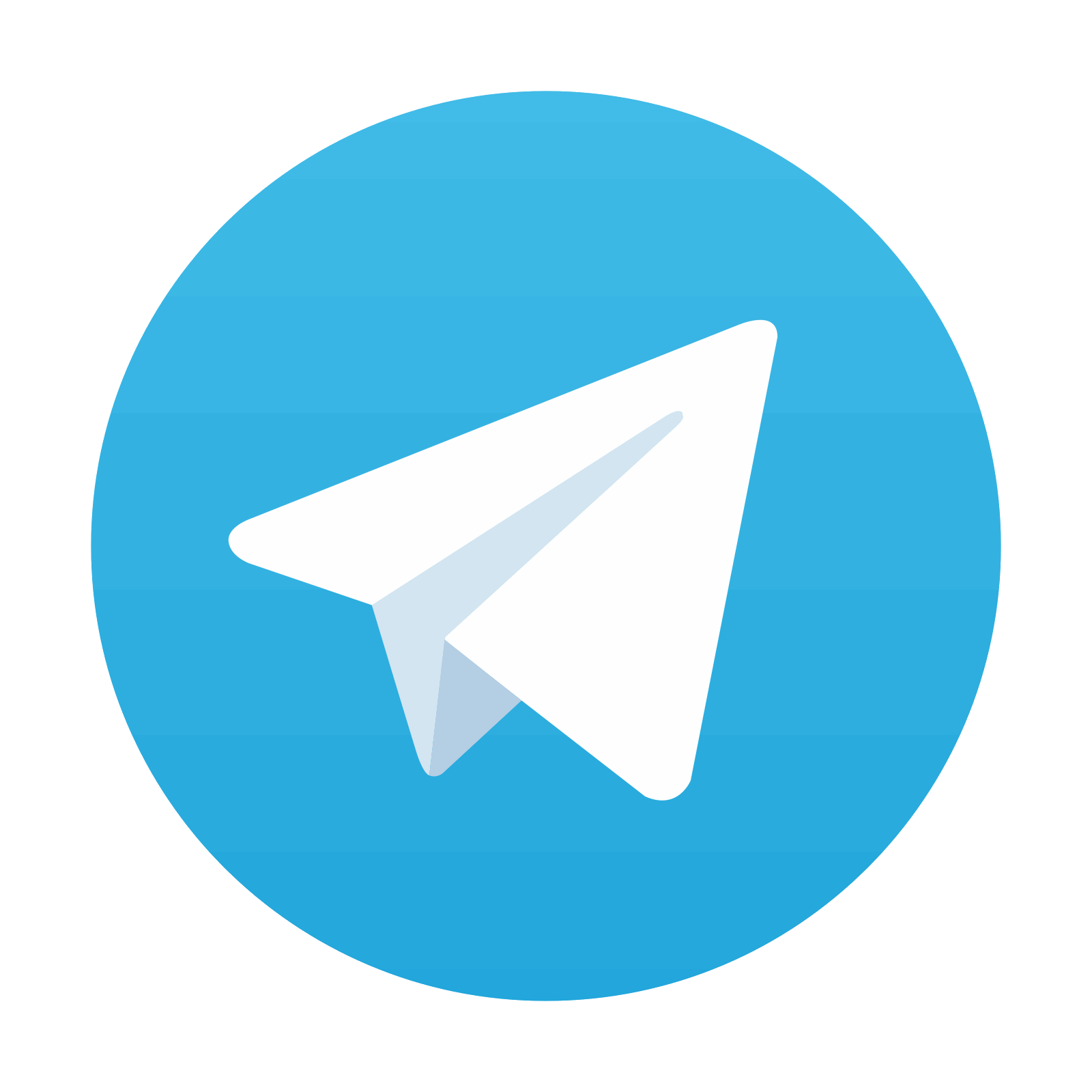
Stay updated, free articles. Join our Telegram channel

Full access? Get Clinical Tree
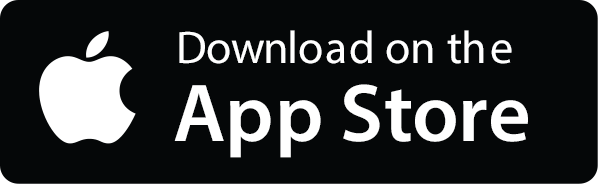
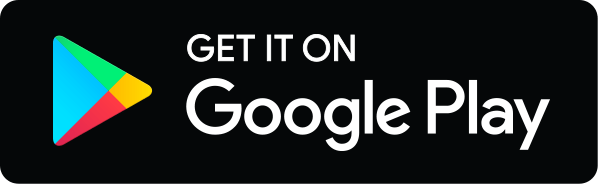