Circadian Rhythms and Circadian Rhythm Sleep-Wake Disorders
Chad Whittlef
Katherine M. Sharkey
LEARNING OBJECTIVES
On completion of this chapter, the reader should be able to:
1. Define and give examples of circadian rhythms.
2. Define the common terms used to describe circadian physiology.
3. Describe the neurobiology of the circadian clock and the effects of light, darkness, and other factors on circadian function.
4. Identify the clinical features of circadian rhythm sleep-wake disorders (CRSWD).
5. Describe circadian rhythm measurements used for clinical diagnosis of CRSWD.
6. Explain laboratory assessments of circadian rhythms.
7. Discuss the most common treatments for CRSWD.
8. Appreciate the potential risks associated with shift work in the sleep laboratory.
KEY TERMS
Advanced sleep-wake phase disorder
Chronotherapy
Circadian rhythms
Circadian rhythm sleep-wake disorders
Constant routine
Delayed sleep-wake phase disorder
Dim light melatonin onset
Entrainment
Forced desynchrony
Free running
Infradian
Irregular sleep-wake rhythm
Jet lag disorder
Light therapy
Masking
Melatonin
Phase
Phase response curve
Phase shift
Shift-work disorder
Sleep-wake diaries
Suprachiasmatic nuclei
Ultradian
Wrist actigraphy
Zeitgeber
FUNDAMENTALS OF CIRCADIAN RHYTHMS
Throughout history, scientists have observed that most living things have physiologic rhythms that parallel the 24-hour cycle of day and night. These are called “circadian rhythms” from the Latin “circa,” meaning about, and “diem,” meaning day, a term coined by Franz Halberg in 1959 (1). Circadian rhythms are found in virtually all living things, including one-celled animals and plants. The sleep-wake cycle, rhythm of core body temperature, and hormonal rhythms (e.g., melatonin) fluctuating across the 24-hour period are examples of human circadian rhythms. Circadian rhythms are so foundational to physiology and behavior that the scientists whose work unraveled the molecular mechanisms of circadian rhythms, Jeffrey Hall, Michael Rosbash, and Michael Young, were awarded the 2017 Nobel Prize in Physiology or Medicine for their contributions to science (2).
In addition to circadian rhythms, there are other oscillations that are present in humans and animals. Ultradian rhythms are rhythms shorter than 24 hours, for instance, the rapid eye movement-nonrapid eye movement (REM-NREM) cycles that are observed in sleep. The term infradian rhythms refers to oscillations that are longer than 24 hours. As an example, the menstrual cycle approximates 28 days.
Typically, circadian rhythms are represented by a sine wave (Fig. 17-1). Various terms are used to describe different aspects of the rhythm. The period, also referred to as tau, is the length of the rhythm. This is usually very
close to 24 hours. The amplitude is the magnitude of the rhythm from its peak to nadir. The amplitude of different circadian rhythms is variable and can be affected by age, sex, sleep state, and so on. The phase refers to the circadian position at any specific instant of time. Depending on what rhythm is measured, different phase markers are used. For instance, when the circadian rhythm of core temperature is measured, the temperature minimum or maximum is commonly used as a marker of phase. When hormonal rhythms are measured—most commonly melatonin—the onset, offset, or peak of hormone secretion is used as a phase marker.
close to 24 hours. The amplitude is the magnitude of the rhythm from its peak to nadir. The amplitude of different circadian rhythms is variable and can be affected by age, sex, sleep state, and so on. The phase refers to the circadian position at any specific instant of time. Depending on what rhythm is measured, different phase markers are used. For instance, when the circadian rhythm of core temperature is measured, the temperature minimum or maximum is commonly used as a marker of phase. When hormonal rhythms are measured—most commonly melatonin—the onset, offset, or peak of hormone secretion is used as a phase marker.
NEUROBIOLOGIC BASIS OF THE INTERNAL CIRCADIAN CLOCK
Circadian rhythms are endogenously driven by a master circadian clock located in the suprachiasmatic nuclei (SCN) of the anterior hypothalamus (3, 4, 5). Information about the lighting conditions of the external environment is conveyed to the SCN from the retina through the retinohypothalamic tract (6, 7) (Fig. 17-2). The cells in the retina responsible for conveying information about light and dark are called intrinsically photosensitive retinal ganglion cells (8); these ganglion cells differ from the conventional ganglion cells that transmit rod and cone input to the brain for visual image formation. The SCN also receive input from the intergeniculate leaflet of the thalamus and the midbrain raphe nuclei. These two pathways are thought to transmit both photic (light) and nonphotic information to the circadian clock (6).
Most SCN projections stay within the hypothalamus, although a small fraction of SCN neurons terminate in the basal forebrain and midline thalamus (7). Secondary efferents from these three areas project to many brain regions, including the neocortex, hippocampus, basal ganglia, anterior pituitary, hypothalamus, reticular formation, and pineal gland. It is through this expansive network that the SCN exert control over many physiologic functions, including endocrine regulation, body temperature, the sleep-wake cycle, metabolism, autonomic regulation, psychomotor and cognitive performance, attention, memory, and emotion (7).
Recent progress in circadian biology has demonstrated that circadian physiology is driven by a hierarchical multioscillator system where the SCN serves as the central circadian clock orchestrating peripheral clocks in the various organ systems throughout the body (8). These peripheral clocks are responsive to, and integrate, information from input outside of the SCN. For instance, circadian rhythms of liver function and metabolism are influenced by a food-entrainable oscillator located in brain regions outside the SCN.
Circadian rhythm researchers measure physiologic rhythms that are downstream outputs of the endogenous clock to infer the phase position of the circadian rhythms. The circadian rhythms of the hormone melatonin and core body temperature are common measures of circadian phase in humans.
EFFECTS OF THE LIGHT-DARK CYCLE ON CIRCADIAN RHYTHMS
As mentioned, sensory receptors in the retina provide information about the light-dark cycle to the master circadian clock in SCN. The light-dark cycle helps the organism remain synchronized or entrained to the 24-hour day of the external environment (Fig. 17-3). In the absence of a light-dark cycle and other time cues, circadian rhythms “free run” at a rate not equal to 24 hours, but equal to the endogenous period length, or tau. The period (tau) is close to, but not exactly, 24 hours. Free running is observed in humans when they have no photic (light) stimuli, for instance in people with blindness and no photoreception (9, 10, 11, 12), or when the light-dark cycle to which they are exposed is outside of the time range of possible biologic entrainment, as occurs in orbiting astronauts, for instance.
Wever and colleagues (13) performed some of the initial experiments using bright light to entrain human
circadian rhythms, confirming an important principle of human circadian rhythms: the light-dark cycle is one of the most powerful synchronizers of the endogenous circadian pacemaker in humans. More recent studies have tested various intensities of light (14) and have shown that in humans, as in animals, the brighter the light is, the stronger it is as an entraining stimulus. More recent studies suggest that the circadian system is most sensitive to short-wavelength (blue-green spectrum) light (15, 16, 17).
circadian rhythms, confirming an important principle of human circadian rhythms: the light-dark cycle is one of the most powerful synchronizers of the endogenous circadian pacemaker in humans. More recent studies have tested various intensities of light (14) and have shown that in humans, as in animals, the brighter the light is, the stronger it is as an entraining stimulus. More recent studies suggest that the circadian system is most sensitive to short-wavelength (blue-green spectrum) light (15, 16, 17).
Whether free running or entrained, circadian rhythms can be reset to a new time. This process is called “phase shifting.” Humans are frequently faced with situations in which their circadian rhythms are misaligned with the environmental light-dark cycle. This can be a result of jet lag, daylight savings time, or night shift work. In these situations, circadian rhythms may (but do not always) phase-shift to adapt to the new light-dark cycle. Various external stimuli, called zeitgebers (from the German “time giver”), act as time cues and can affect the internal clock, causing a resetting or phase shifting of circadian rhythms. Photic information about the light-dark cycle is the strongest stimulus for resetting the biologic clock (18). Phase shifts differ in direction and magnitude depending on where in the circadian cycle (at what circadian phase) the zeitgeber is administered.
PHASE-SHIFTING EFFECTS OF LIGHT AND LIGHT PHASE RESPONSE CURVES
The relationships between circadian rhythms, phase shifting, and various time cues or zeitgebers are described using phase response curves (PRCs). A PRC is derived by administering a stimulus at many different circadian phases and measuring the resulting shifts in the circadian clock. The multiple phase-shift data points are then plotted to predict the magnitude and direction of a phase shift given a particular stimulus at a specific circadian phase.
Several PRCs describing how humans respond to light exposure have been published (13, 19, 20, 21). A schematic PRC (22) for light is shown in Figure 17-4. When humans are exposed to light at the end of the
day and beginning of the night, circadian rhythms shift to a later time, which is called a “phase delay.” In contrast, when light exposure occurs at the end of the night and early part of the day, circadian rhythms shift to an earlier time, which is called a “phase advance.” Minimal phase shifting occurs when humans are exposed to light in the middle of the day. Shifts in circadian phase elicited by light are dependent not only on the timing and duration of the exposure (14, 19, 23) but also on the intensity of light, which decreases as distance from the light source increases (17), and the wavelength of light (24, 25, 26).
day and beginning of the night, circadian rhythms shift to a later time, which is called a “phase delay.” In contrast, when light exposure occurs at the end of the night and early part of the day, circadian rhythms shift to an earlier time, which is called a “phase advance.” Minimal phase shifting occurs when humans are exposed to light in the middle of the day. Shifts in circadian phase elicited by light are dependent not only on the timing and duration of the exposure (14, 19, 23) but also on the intensity of light, which decreases as distance from the light source increases (17), and the wavelength of light (24, 25, 26).
PHASE-SHIFTING EFFECTS ON NONPHOTIC STIMULI
In addition to light, other nonphotic stimuli have been shown to affect circadian rhythms. For instance, exogenous administration of the hormone melatonin can lead to phase shifts in the circadian clock. Lewy and colleagues (27, 28) produced a PRC for melatonin that demonstrates that circadian phase delays are produced when melatonin is administered in the later hours of sleep and in the morning, and phase advances are produced when melatonin is administered in the afternoon and early evening.
Physical activity or exercise has also been shown to phase-shift the human circadian clock (29, 30, 31, 32). Phase advances in circadian rhythms occur after exercise in the evening, and phase delays are produced with exercise in the middle of the night (30). The magnitude of the circadian phase shifts produced with exercise appears to be related to the duration and intensity of the physical activity.
TYPES OF CIRCADIAN RHYTHM SLEEP-WAKE DISORDERS
Before describing the types of circadian rhythm sleep-wake disorders (CRSWD), it is important to note that by influencing the timing of virtually all physiologic and behavioral phenomena, circadian rhythms have an impact on health and safety that extends far beyond CRSWD. Examples of circadian variation include the timing and severity of clinical symptoms unrelated to sleep disorders and the daily pattern of critical events in the population, such as stroke, myocardial infarction, and motor vehicle accidents. In addition, the outcomes of clinical experiments, diagnostic tests, and treatments can all vary as a function of administration time. Clearly, the influence of circadian rhythms needs to be appreciated throughout the biomedical sciences.
Shift-Work Disorder
The misalignment of the sleep-wake cycle and intrinsic circadian rhythms seen in CRSWD is demonstrated in the case of shift work. Shift-work disorder (SWD) can occur when work schedules conflict with the timing and duration of an individual’s normal sleep period. Working a fixed night shift (when the circadian clock is programmed for sleep) is a primary example of such a conflict, but early morning shifts and rotating shifts can be just as difficult to tolerate.
Symptoms of SWD include reduced sleep quality and quantity as well as sleepiness during time awake. Sleep duration during the day following a night shift is typically reduced by several hours (33, 34). Subjective sleep quality can be impaired, and sleep is nonrestorative. Impairments of sleep quality during shift work include prolonged sleep latencies, sleep maintenance difficulties, and early termination of the sleep period. Owing in part to this reduction in sleep quality and quantity, an individual typically experiences excessive sleepiness during the time of day in which they are attempting to remain awake and work. Another major factor contributing to sleepiness during a night shift is the acute reduction in alertness that usually occurs in the early morning hours (35). This early morning nadir of alertness is a circadian phenomenon, occurring independent of accumulated sleep loss. At the end of a night shift, sleepiness is typically high because of this reduced alerting effect in tandem with the long period of wakefulness that has elapsed since the last sleep period. This can potentially place night shift workers in a dangerous position during the commute home (36).
A number of other disturbances unrelated to sleep may be reported by shift workers (37, 38). A common complaint is increased gastrointestinal disturbance. Cardiovascular disease, as well as several major risk factors for this disease—smoking, high cholesterol, hypertension, and obesity—have been found to be more prevalent among shift workers (39, 40). Reproductive difficulties such as menstrual irregularities and increased time to conception may be more prevalent in female shift workers (41, 42, 43). Night shift work has repeatedly been associated with increased risk of developing cancer and has been classified as a “probable carcinogen” by the International Agency for Research on Cancer (44). The psychosocial consequences of shift work can include disturbances of family and marital relationships (45).
Although SWD may be transient in that resuming a daytime work/nighttime sleep schedule will attenuate its symptoms, adaptation to late night, early morning, and rotating shifts is difficult to achieve, and in most cases does not occur. Adaptation to a nighttime work and daytime sleep schedule, however, is possible if appropriate steps are taken to reinforce a nocturnal
lifestyle. This is rare among most shift workers because environmental zeitgebers, such as light, as well as social and domestic responsibilities, reinforce a diurnal schedule, preventing significant adaptation from occurring. In addition, maintaining a nocturnal lifestyle is not a priority for most individuals, suggesting that not only is circadian adaptation difficult, but that it may be undesirable. Figure 17-5 shows the activity of a shift worker over a several-week period, and Figure 17-6G depicts a typical phase relationship between the temperature minimum and the sleep period for a night shift worker.
lifestyle. This is rare among most shift workers because environmental zeitgebers, such as light, as well as social and domestic responsibilities, reinforce a diurnal schedule, preventing significant adaptation from occurring. In addition, maintaining a nocturnal lifestyle is not a priority for most individuals, suggesting that not only is circadian adaptation difficult, but that it may be undesirable. Figure 17-5 shows the activity of a shift worker over a several-week period, and Figure 17-6G depicts a typical phase relationship between the temperature minimum and the sleep period for a night shift worker.
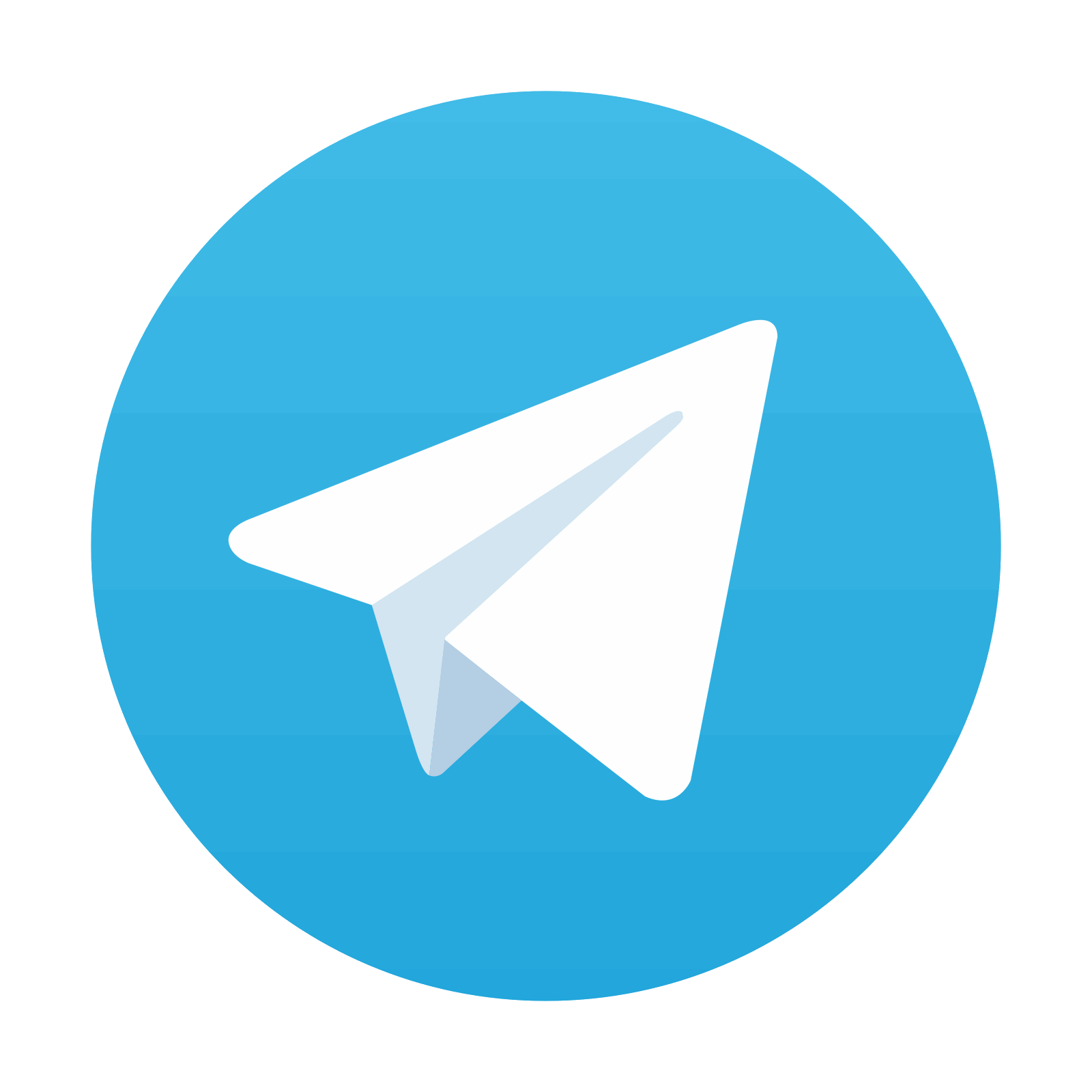
Stay updated, free articles. Join our Telegram channel

Full access? Get Clinical Tree
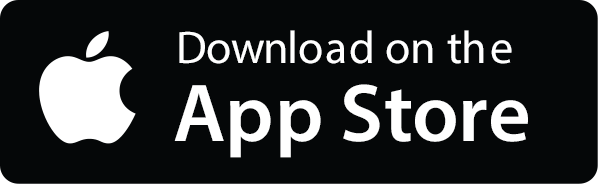
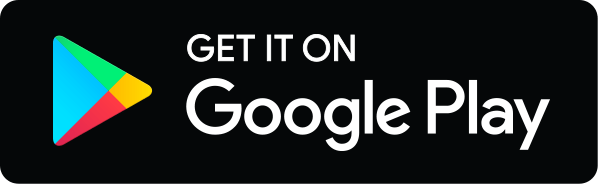