Glioblastoma multiforme is a malignant, relentless brain cancer with no known cure, and standard therapies leave significant room for the development of better, more effective treatments. Immunotherapy is a promising approach to the treatment of solid tumors that directs the patient’s own immune system to destroy tumor cells. The most successful immunologically based cancer therapy to date involves the passive administration of monoclonal antibodies, but significant antitumor responses have also been generated with active vaccination strategies and cell-transfer therapies. This article summarizes the important components of the immune system, discusses the specific difficulty of immunologic privilege in the central nervous system, and reviews treatment approaches that are being attempted, with an emphasis on active immunotherapy using peptide vaccines.
Glioblastoma multiforme (GBM) is the most common primary malignant tumor in the adult central nervous system. Current therapy for this disease usually includes surgical resection, radiation, and the administration of temozolomide chemotherapy. It is a devastating diagnosis, because even with this standard treatment, median survival is less than 15 months. Additionally, most current therapies are not selective for tumor cells; and therefore, large amounts of normal healthy tissue are injured as collateral damage while treating these tumors. This is a problem for most types of cancer, and particularly GBM, as it is highly infiltrative.
The ideal treatment for GBM needs to be selective enough to accurately discriminate between normal tissue and tumor cells and be able to reach across the blood brain barrier, while being potent enough to kill all of the tumor cells. The immune system certainly has this capability as evidenced by its ability to fight infections within the central nervous system (CNS). Therefore, the immune system could be an ideal candidate for an effective GBM therapy as well. Although it would appear that the immune system inherently possesses the ability to recognize and eliminate GBMs, the fact that GBMs are able to proliferate in these patients suggests that without intervention, the immune system is not an effective check against this disease. The reasons for this failure are likely multifactorial, including the immune-privileged status of the CNS and immunosuppressive effects of the tumor. As there is clearly a significant need for more effective treatment options against GBM, attempting to direct the immune system to attack this disease is a promising area of active research.
Immunotherapy
Multiple different approaches are being used to activate the immune system against the tumor. To provoke the immune system into being stimulated against tumor cells, attempts have included injections of peptides, proteins, DNA, RNA, viruses encoding cancer antigens, viruslike particles, whole tumor cells or their lysates, altered tumor cells that express cytokines, heat shock proteins, pulsed dendritic cells (DCs), and anti-idiotype antibodies. Other methods are directed at overcoming tumor-related immune suppression by blocking the effect of inhibitory cells of the immune system or by blocking the effect of inhibitory molecules secreted by tumors like transforming growth factor-β (TGF-β). Administration of cytokines that promote lymphocyte expansion have led to some cases of dramatic tumor regression. For example interleukin-2 (IL-2) has been studied in the treatment of renal cancer, metastatic melanoma, and non-Hodgkin’s lymphoma with some important treatment successes.
The two main approaches for immunotherapy can be grouped into (active) vaccine therapy or (passive or adoptive) cell-transfer therapy ( Fig. 1 ). To be successful, both approaches require the patient to develop lymphocytes that are activated against tumor cell antigens. Vaccine therapy in general follows the principle that injections of various substances that ultimately result in the presentation of tumor peptides to the patient’s immune system will sensitize lymphocytes against an antigen of interest, and then these lymphocytes migrate to the tumor and cause cell killing. Vaccines typically are derived from cancer cells, cancer cell lysates, or specific peptides or proteins that encode cancer antigens. These can be pulsed onto antigen-presenting cells (APCs), which are then injected, or can be administered by themselves with adjuvants or cytokines that stimulate the immune system in a nonspecific manner.

In contrast, cell-transfer therapy involves removing lymphocytes from the patient, expanding those lymphocytes, usually T cells, that demonstrate activity against the tumor antigens, and then reintroducing that expanded and enriched cell population back into the same patient so they can migrate to the tumor and cause cell killing. In one form of cell-transfer therapy, T cells that are able to respond to defined cancer antigens are harvested from resected tumors and are termed tumor-infiltrating lymphocytes (TILs). Importantly, ex vivo cell transfer therapy allows one to directly manipulate both the lymphocytes outside of the body and the host immune system itself before infusion. It is thought that these manipulations before infusion are beneficial because they allow for stimulation and expansion of the cells at the time of reinfusion and also correction of the host environment to make it more hospitable for the infused, activated cells to be able to attack the tumor. In fact, such host manipulations have recently enhanced the success of cell transfer therapy significantly and revolutionized this approach.
Immune system components
The role of the immune system is basically to discriminate between self and non-self, and thereby defend the body from foreign attack. This role is critical for defense from microorganisms and other pathogens, but also prunes the body’s own cells, causing removal of those cells that are no longer viable or exhibit a number of different warning signals to the immune system. The immune system is traditionally divided into two main arms, the innate and adaptive systems. The innate system is composed of macrophages/monocytes, neutrophils, natural killer cells, basophils, and eosinophils. The complement system is also a component of the innate system. The innate system is so named because it is present from birth, and it provides the initial line of defense, but it is not as specific as the adaptive system and does not have any memory of the protein previously defended against.
In contrast, the adaptive immune system is able to refine its response to improve its ability to detect and respond against a specific offender particularly if it has defended against this foreigner previously. The main components of the adaptive immune system are T and B lymphocytes, and both cell types are able to adapt their response to foreign antigens and microorganisms. T cells are named because of their maturation in the thymus. Mature T cells are mainly divided into CD8 + cytotoxic and CD4 + helper cell types. Both types possess a T-cell receptor, and through this they bind to and recognize foreign antigens that are presented on the surface of tumor cells or cells infected by viruses. These antigens are small peptide fragments that are presented in the groove of human leukocyte antigen (HLA) molecules that are present on almost all cells in the human body. CD8 + cells recognize HLA class I molecules, and the peptide antigens presented on these molecules are typically 8 to 10 amino acids in length. These peptides come from intracellular proteins that are broken down in the proteasome. When activated, CD8 + cells typically cause cell-mediated killing. CD4 + cells, in contrast, recognize HLA class II molecules, and these molecules present peptides that are typically 13 amino acids in length. These peptides that are presented on class II molecules arise from the digestion of extracellular proteins that were taken into the cell via endocytosis. After activation, CD4 + cells modulate the immune response by secreting different cytokines, and in general adjust the response of other cells of the immune system. Most cells in the body express HLA class I molecules, but HLA class II expression is limited to antigen presenting cells.
B cells are named because of their derivation from precursor cells in the bone marrow. They are a component of the adaptive response, and they secrete antibodies in response to foreign antigens. Antibodies are secreted in five main types: IgG, IgM, IgE, IgD, and IgA. Antibodies bind to specific antigens through protein-protein noncovalent biding, and this can lead to destruction of the offending cell or microorganism through a variety of means. In theory at least, each antibody recognizes a single antigen, but the wide diversity of antibodies that is created allows them as a whole to recognize a large number of antigens. When cells become abnormal from infection, mutation, or other danger to the host, they can change their surface protein expression. These altered or unique proteins can become antigens that can then be bound by antibodies, which leads to immune detection and subsequent killing of these cells by natural killer cells. This is called antibody-dependent cell-mediated cytotoxicity (ADCC).
Central nervous system immune privilege
It was classically believed that a few areas of the body, including the CNS, are privileged from standard surveillance by the immune system. Early studies by Medawar in 1948 suggested that tissue grafts transplanted into the brain and other sites were not rejected, suggesting that these areas were not under immune surveillance. This idea has been supported by numerous findings specific to the CNS, including the decreased amount of HLA antigen presentation, the lack of conventional antigen presenting cells, the lack of a conventional lymphatic drainage system, and the existence of the blood brain barrier (BBB), which helps to block entry into the CNS. However, the reality is much more complicated and cells of the immune system are able to enter and interact with the CNS to a great extent. Furthermore, HLA antigen presentation does occur on astrocytes, microglia, endothelial cells, and in the choroid plexus, and microglia in particular may be the predominant antigen presenting cells within the CNS. Although traditional lymphatics are not present in the CNS, it has been suggested that cervical lymph nodes do receive lymphatic drainage from the brain and that an immune response can be generated against antigens in the brain via this pathway.
Antibody penetration across the BBB has generally been thought to be poor, as there is a low level of immunoglobulin in the CNS as compared with serum levels. Importantly, antibody permeability can drastically increase in certain conditions such as widespread inflammation. Furthermore, antibodies have been shown to penetrate the CNS, although at 0.1% to 10.0% of the peripheral blood levels.
Early results from our group proved in humans that a radiolabeled monoclonal antibody against the extracellular matrix antigen tenascin was able to reach intracerebral tumor when given via intravenous administration, although only 0.0006% to 0.0043% of the total injected dose was measured at the tumor site. Despite the low dose, this demonstrates that antibody is able to reach intracerebral tumors. However, it also suggests that in order to achieve clinical benefits, it may be necessary to give higher or more sustained doses of antibody, or more specific antibodies that do not exhibit systemic binding.
Later studies using antibodies recognizing epitopes restricted to the CNS have confirmed that antibodies can localize to the site of an intracerebral glioma after systemic administration, as Fig. 2 depicts. In this study, monoclonal antibody 806 was used, which recognizes the tumor-specific antigen, epidermal growth factor receptor variant III (EGFRvIII). This antibody was radiolabeled with Indium-111, and after a single dose was administered, imaging verified that the radiolabeled antibody localized to the intracerebral tumor site with no evidence of uptake in normal tissues. Localization of antibody to subcutaneous tumor xenografts in mice has also been published. When an antibody is highly specific for the tumor and does not cross-react with systemic antigens, the lack of systemic binding may make it possible to get a much higher percentage of antibody penetration into the CNS and to the tumor site.
Moreover, the existence of paraneoplastic syndromes conclusively demonstrates that antibodies can penetrate the CNS at clinically significant levels. In this spectrum of disorders, antibodies directed against systemic tumor antigens have cross-reactivity with neuronal cells and therefore provoke an immune response in the CNS that leads to significant clinical morbidity. Numerous antibodies arising from a variety of different cancers have been detected, including anti-Hu, anti-Yo, anti-Ri, anti-CV2, anti-Ma, anti-Tr, anti-amphiphysin, voltage gated potassium channel (VGKC) antibodies, anti–voltage gated calcium channel (anti-VGCC) antibodies, and antibodies to the N-methyl- d -aspartate (NMDA) receptor. Many of these antibodies can also be detected in the cerebrospinal fluid (CSF) of affected individuals. Along with antibodies, T-cell responses in the CNS are also seen and it is thought that both responses contribute to the neuronal injury seen in these diseases. Often, in addition to treatment of the underlying tumor, immune suppression is used to control the CNS immune response.
Although it appears that antibody responses may be a more predominant aspect of the response against CNS antigens than cell-mediated responses, all aspects of the immune response are able to be generated in the CNS. Prior experiments have shown that activated T cells can readily cross an intact BBB. When these activated T cells are specific to myelin basic protein, they can cause normal rats to undergo lethal experimental autoimmune encephalitis (EAE). Numerous adhesion molecules and chemotactic factors have been implicated in this process. Naïve T cells are not believed to cross the normal BBB, however.
As an acknowledgment to the limitations caused by the BBB, there have also been attempts to bypass it via direct drug delivery to the brain. Some of the more promising of these methods include transnasal drug delivery and convection-enhanced delivery (CED). Transnasal drug delivery can theoretically bypass the blood brain barrier and achieve drug levels in the CNS, although this method has largely failed when it has been studied in humans. Another important method that may be successful in the future is convection-enhanced delivery (CED), where drug is delivered via catheters directly to the tumor site under a steady pressure. This method permits better drug distribution than simple diffusion, and should distribute drug over much greater areas in a more homogeneous fashion. This method has been successfully used by our group and others in humans for targeted treatment of GBM. Research is ongoing in all of these areas, and much more work still needs to be done to validate these various delivery techniques.
Immune system components
The role of the immune system is basically to discriminate between self and non-self, and thereby defend the body from foreign attack. This role is critical for defense from microorganisms and other pathogens, but also prunes the body’s own cells, causing removal of those cells that are no longer viable or exhibit a number of different warning signals to the immune system. The immune system is traditionally divided into two main arms, the innate and adaptive systems. The innate system is composed of macrophages/monocytes, neutrophils, natural killer cells, basophils, and eosinophils. The complement system is also a component of the innate system. The innate system is so named because it is present from birth, and it provides the initial line of defense, but it is not as specific as the adaptive system and does not have any memory of the protein previously defended against.
In contrast, the adaptive immune system is able to refine its response to improve its ability to detect and respond against a specific offender particularly if it has defended against this foreigner previously. The main components of the adaptive immune system are T and B lymphocytes, and both cell types are able to adapt their response to foreign antigens and microorganisms. T cells are named because of their maturation in the thymus. Mature T cells are mainly divided into CD8 + cytotoxic and CD4 + helper cell types. Both types possess a T-cell receptor, and through this they bind to and recognize foreign antigens that are presented on the surface of tumor cells or cells infected by viruses. These antigens are small peptide fragments that are presented in the groove of human leukocyte antigen (HLA) molecules that are present on almost all cells in the human body. CD8 + cells recognize HLA class I molecules, and the peptide antigens presented on these molecules are typically 8 to 10 amino acids in length. These peptides come from intracellular proteins that are broken down in the proteasome. When activated, CD8 + cells typically cause cell-mediated killing. CD4 + cells, in contrast, recognize HLA class II molecules, and these molecules present peptides that are typically 13 amino acids in length. These peptides that are presented on class II molecules arise from the digestion of extracellular proteins that were taken into the cell via endocytosis. After activation, CD4 + cells modulate the immune response by secreting different cytokines, and in general adjust the response of other cells of the immune system. Most cells in the body express HLA class I molecules, but HLA class II expression is limited to antigen presenting cells.
B cells are named because of their derivation from precursor cells in the bone marrow. They are a component of the adaptive response, and they secrete antibodies in response to foreign antigens. Antibodies are secreted in five main types: IgG, IgM, IgE, IgD, and IgA. Antibodies bind to specific antigens through protein-protein noncovalent biding, and this can lead to destruction of the offending cell or microorganism through a variety of means. In theory at least, each antibody recognizes a single antigen, but the wide diversity of antibodies that is created allows them as a whole to recognize a large number of antigens. When cells become abnormal from infection, mutation, or other danger to the host, they can change their surface protein expression. These altered or unique proteins can become antigens that can then be bound by antibodies, which leads to immune detection and subsequent killing of these cells by natural killer cells. This is called antibody-dependent cell-mediated cytotoxicity (ADCC).
Central nervous system immune privilege
It was classically believed that a few areas of the body, including the CNS, are privileged from standard surveillance by the immune system. Early studies by Medawar in 1948 suggested that tissue grafts transplanted into the brain and other sites were not rejected, suggesting that these areas were not under immune surveillance. This idea has been supported by numerous findings specific to the CNS, including the decreased amount of HLA antigen presentation, the lack of conventional antigen presenting cells, the lack of a conventional lymphatic drainage system, and the existence of the blood brain barrier (BBB), which helps to block entry into the CNS. However, the reality is much more complicated and cells of the immune system are able to enter and interact with the CNS to a great extent. Furthermore, HLA antigen presentation does occur on astrocytes, microglia, endothelial cells, and in the choroid plexus, and microglia in particular may be the predominant antigen presenting cells within the CNS. Although traditional lymphatics are not present in the CNS, it has been suggested that cervical lymph nodes do receive lymphatic drainage from the brain and that an immune response can be generated against antigens in the brain via this pathway.
Antibody penetration across the BBB has generally been thought to be poor, as there is a low level of immunoglobulin in the CNS as compared with serum levels. Importantly, antibody permeability can drastically increase in certain conditions such as widespread inflammation. Furthermore, antibodies have been shown to penetrate the CNS, although at 0.1% to 10.0% of the peripheral blood levels.
Early results from our group proved in humans that a radiolabeled monoclonal antibody against the extracellular matrix antigen tenascin was able to reach intracerebral tumor when given via intravenous administration, although only 0.0006% to 0.0043% of the total injected dose was measured at the tumor site. Despite the low dose, this demonstrates that antibody is able to reach intracerebral tumors. However, it also suggests that in order to achieve clinical benefits, it may be necessary to give higher or more sustained doses of antibody, or more specific antibodies that do not exhibit systemic binding.
Later studies using antibodies recognizing epitopes restricted to the CNS have confirmed that antibodies can localize to the site of an intracerebral glioma after systemic administration, as Fig. 2 depicts. In this study, monoclonal antibody 806 was used, which recognizes the tumor-specific antigen, epidermal growth factor receptor variant III (EGFRvIII). This antibody was radiolabeled with Indium-111, and after a single dose was administered, imaging verified that the radiolabeled antibody localized to the intracerebral tumor site with no evidence of uptake in normal tissues. Localization of antibody to subcutaneous tumor xenografts in mice has also been published. When an antibody is highly specific for the tumor and does not cross-react with systemic antigens, the lack of systemic binding may make it possible to get a much higher percentage of antibody penetration into the CNS and to the tumor site.
Moreover, the existence of paraneoplastic syndromes conclusively demonstrates that antibodies can penetrate the CNS at clinically significant levels. In this spectrum of disorders, antibodies directed against systemic tumor antigens have cross-reactivity with neuronal cells and therefore provoke an immune response in the CNS that leads to significant clinical morbidity. Numerous antibodies arising from a variety of different cancers have been detected, including anti-Hu, anti-Yo, anti-Ri, anti-CV2, anti-Ma, anti-Tr, anti-amphiphysin, voltage gated potassium channel (VGKC) antibodies, anti–voltage gated calcium channel (anti-VGCC) antibodies, and antibodies to the N-methyl- d -aspartate (NMDA) receptor. Many of these antibodies can also be detected in the cerebrospinal fluid (CSF) of affected individuals. Along with antibodies, T-cell responses in the CNS are also seen and it is thought that both responses contribute to the neuronal injury seen in these diseases. Often, in addition to treatment of the underlying tumor, immune suppression is used to control the CNS immune response.
Although it appears that antibody responses may be a more predominant aspect of the response against CNS antigens than cell-mediated responses, all aspects of the immune response are able to be generated in the CNS. Prior experiments have shown that activated T cells can readily cross an intact BBB. When these activated T cells are specific to myelin basic protein, they can cause normal rats to undergo lethal experimental autoimmune encephalitis (EAE). Numerous adhesion molecules and chemotactic factors have been implicated in this process. Naïve T cells are not believed to cross the normal BBB, however.
As an acknowledgment to the limitations caused by the BBB, there have also been attempts to bypass it via direct drug delivery to the brain. Some of the more promising of these methods include transnasal drug delivery and convection-enhanced delivery (CED). Transnasal drug delivery can theoretically bypass the blood brain barrier and achieve drug levels in the CNS, although this method has largely failed when it has been studied in humans. Another important method that may be successful in the future is convection-enhanced delivery (CED), where drug is delivered via catheters directly to the tumor site under a steady pressure. This method permits better drug distribution than simple diffusion, and should distribute drug over much greater areas in a more homogeneous fashion. This method has been successfully used by our group and others in humans for targeted treatment of GBM. Research is ongoing in all of these areas, and much more work still needs to be done to validate these various delivery techniques.
Tumor-related immune suppression
Tumors appear to be accompanied by immune suppression both locally and systemically. This is likely multifactorial in nature, and multiple immunosuppressive molecules have been implicated in addition to the important role suggested by inhibitory cells of the immune system. For example, TGF-β, a cytokine secreted by many tumor cells appears to be an important component of this immunosuppression. Fas ligand, a protein on the surface of many tumor cells that is capable of killing T cells directly, may also be important in inhibiting the immune response. Many other molecules have also been implicated and may play important roles, including IL-10, prostaglandin E 2 , and NF-κB. Regulatory T cells are a subset of CD4 + cells and generally function as inhibitory cells of the immune system. They have been shown to be an increased fraction of lymphocyte counts in patients with GBMs, and their depletion has been shown to improve antitumor responses. Also, the inhibitory T-cell receptor CTLA-4 is likely involved, as it has been shown that blockade of this receptor improves antitumor immune response. Tumors are also known to have immune evasion techniques, including decreased amount of antigen presentation molecules. It will undoubtedly be critical to understand and overcome the immune suppression and evasion techniques in order to develop an effective immunotherapy.
Monoclonal antibodies as therapeutics
There have been numerous clinical trials verifying the passive administration of monoclonal antibodies as a viable treatment for cancer. Antibodies have been approved for the treatment of breast cancers, lung cancers, colorectal cancers, and leukemias and lymphomas. Food and Drug Administration (FDA) approval has been granted for trastuzumab (Herceptin), bevacizumab (Avastin), cetuximab (Erbitux), panitumumab (ABX-EGF), ibritumomab tiuxetan (Zevalin), alemtuzumab (Campath), gemtuzumab ozogamicin (Mylotarg), rituximab (Rituxan), and tositumomab (Bexxar). Trastuzumab is a monoclonal antibody against the HER2 receptor and is approved for HER2-positive breast cancer. Bevacizumab is a monoclonal antibody against vascular endothelial growth factor and is approved for the treatment of non–small-cell lung cancer, breast cancer, colorectal cancer, and very recently recurrent GBM. Cetuximab and panitumumab are both directed against the epidermal growth factor receptor, and both are approved for colorectal cancer, whereas cetuximab also has approval against head and neck cancer. The remainder of the listed antibodies are directed against cell surface antigens and are approved for the treatment of leukemias and lymphomas.
Bevacizumab has also demonstrated efficacy against metastatic renal cancer and in combination with irinotecan, it has shown efficacy against recurrent GBM. The mechanism of this synergistic effect may be due to slowing tumor growth by slowing angiogenesis, or it possibly may allow for improved delivery of chemotherapy to the tumor by normalizing tumor vasculature. Further studies with bevacizumab against GBM are ongoing, and it recently obtained approval for treatment of recurrent GBM. Interestingly, bevacizumab has also demonstrated efficacy against pituitary adenomas in a mouse model, and newer antibodies that block vascular endothelial growth factor in both humans and mice have also been developed.
Trastuzumab has become an important component of HER2-positive breast cancer therapy, and because of the high prevalence of metastases to the CNS in this disease, there have been a number of studies with data related to the CNS. It has been shown that patients with HER2-overexpressing breast cancer have a greater risk of developing CNS metastases than other breast cancer patients. There is also an increased incidence of CNS metastases in those HER2-positive patients who are undergoing treatment with trastuzumab, but because of the improved control of extracranial disease, there is still improved survival of these patients when compared with unselected breast cancer patients. Another study showed that despite a survival benefit from trastuzumab therapy, there was no delay in onset of CNS metastases, which suggests that this drug is having little effect across the BBB. The possibility that the brain metastases lost their HER2 expression (and would therefore be resistant to the drug) was ruled out in other studies, which supports the conclusion that the drug is simply not being effectively delivered to CNS disease. Furthermore, the ratio of trastuzumab between serum and CSF has been measured during different stages of treatment and disease burdens. Before radiotherapy, the ratio of serum to CSF was 420:1, but this improves to 76:1 after radiotherapy, and after radiotherapy with meningeal carcinomatosis this ratio is 49:1. Other authors have reported in a patient with meningeal carcinomatosis that this ratio was 300:1, but others have also reported on possible treatment benefits of trastuzumab for meningeal carcinomatosis despite these ratios. In summary, it appears that large monoclonal antibodies like trastuzumab are able to penetrate the CNS, but at much lower levels than that found in the serum. In the case of breast cancer, it is important to emphasize that radiation weakens the BBB and improves serum to CSF ratios of trastuzumab. Because radiation has become part of the standard therapy for GBM, its effect may decrease the role of the BBB for that disease as well.
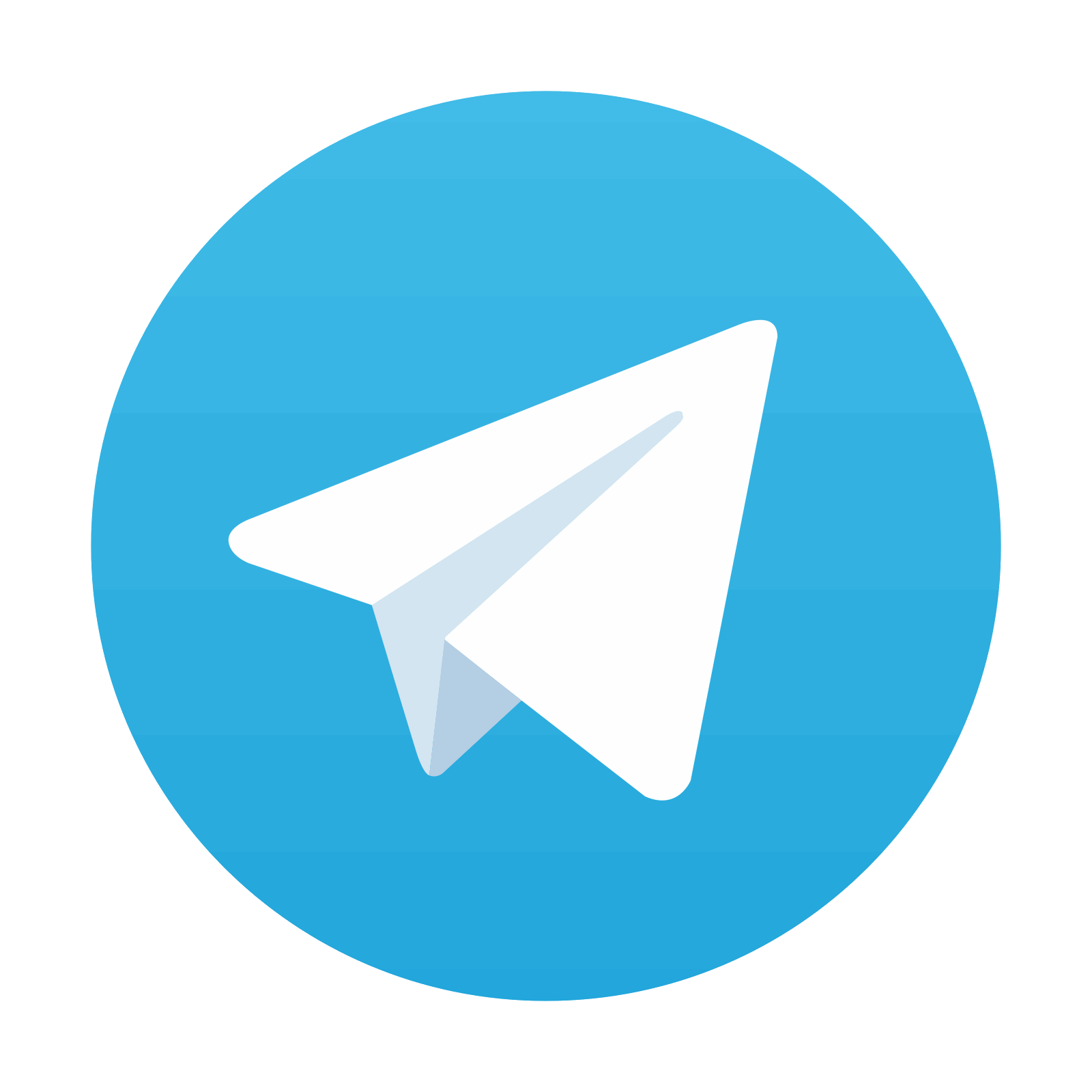
Stay updated, free articles. Join our Telegram channel

Full access? Get Clinical Tree
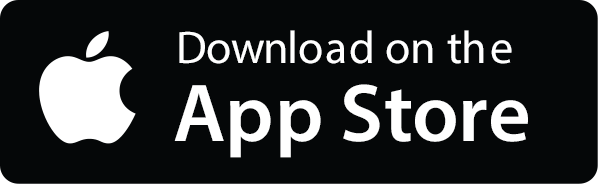
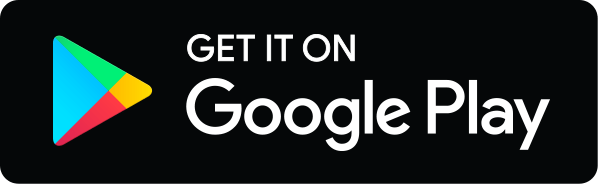