Fig. 14.1
Grandaverage left parietal and parieto-temporal ERP to target and rare non-target Kanizsa illusory stimuli in ASD and control groups (N = 27/per group). The ASD group shows more negative N200 component both to targets and non-targets as compared to controls. The ASD group presents higher and delayed P3b peaks both to target and non-target stimuli as compared to controls
Over frontal ROI P50 amplitudes were significantly more positive to non-target Kanizsa and non-Kanizsa stimuli in individuals with ASD, while P50 latency was prolonged to target stimuli. These results are similar to our findings over parieto-occipital ROI as well as our previous study (Sokhadze et al. 2009b) where ASD patients had excessive frontal positivity to distracter stimuli compared to controls. Again since this early positivity is associated with early categorization and recognition processes and is generally higher to attended stimuli, these results suggest ASD patients are abnormally orientating to task irrelevant stimuli. An exaggerated response to sensory inputs may result in a global inundation of higher level integrative centers with task-irrelevant information during early stages of visual processing.
N100 amplitude over frontal ROI was significantly more negative to target stimuli in the ASD group relative to the control group. Also, a Group X Stimulus interaction for N100 latency indicated a significantly reduced latency in the ASD group to non-Kanizsa stimuli compared to target Kanizsa stimuli. These results corroborate with our previous study where we found an augmented N100 amplitude to target stimuli over frontal ROI as well as a prolonged N100 latency to targets over centroparietal ROI in ASD patients. Amplified and delayed responses to rare, target stimuli in the ASD group may point to visual hypersensitivity and increased general arousal relative to controls, and this may disrupt and delay the processing of target stimuli. Courchesne et al. (1985) reported larger N100 amplitudes at the Cz electrode to all stimuli (Target, Novel, Standard) in autistic patients compared to controls, but the group differences were not found to be significant. These findings may also point to augmented visual responses in ASD during early stages of processing.
P200 amplitude over frontal ROI was found to be equally more positive to all stimuli in the ASD group with a lack of stimulus discrimination; where P200 amplitudes over frontal ROI were indiscernible between target and distracter stimuli in the ASD group, where in the control group P200 amplitude was more positive to targets. The P200 over frontal ROI has been associated with the hierarchal selection of task-relevant features (Kenemans et al. 1993). In ASD globally augmented cortical responses, especially to irrelevant stimuli at early stages of visual processing may be complicating stimulus discrimination processes at the stage of the P200. At behavioral stages, responses of ASD patients did not differ from the control group in reaction time although they had a significantly higher rate or error. The significantly higher rate of error in ASD patients may be a manifestation of early-stage visual sensitivity and consequently a disruption in selective attention and executive function.
Our results show that individuals with ASD have abnormally large cortical responses to task irrelevant stimuli over both parieto-occipital and frontal ROI during early stages of visual processing compared to the control group. Also, ASD patients showed signs of an overall disruption in stimulus discrimination compared to the control group as evidenced by ERPs and a significantly higher rate of motor response errors. Sensory hyper-reactivity has been well documented in the auditory domain (see for review Gomes et al. 2008) but not during visual tasks. Patients with ASD may have sensitivities at early-stages of visual processing as well which may be sequentially affecting their ability to effectively discriminate irrelevant from relevant stimuli in visual processing tasks.
Evoked gamma frequency oscillations: One-way ANOVA analysis revealed that early (i.e., 401–200 ms post-stimulus) evoked gamma power was significantly higher to target Kanizsa stimuli at all channels in the control group compared to the ASD group (p < .001). A Stimulus (Target, Non-target) × Group (ASD, Control) interaction was significant at all channels (p < .001) indicating significantly higher evoked gamma power to target Kanizsa stimuli compared to non-target Kanizsa stimuli in controls; while the ASD group had a minimal difference in evoked gamma power between target and non-target Kanizsa stimuli actually demonstrating more gamma power to non-targets. An analysis of differences in evoked gamma power between anterior and posterior regions revealed a Topography (Anterior, Posterior) × Group (ASD, Control) interaction over the left hemisphere to all stimuli where controls had higher evoked gamma power over frontal (F7) compared to posterior (P7) regions (F = 5.48, p = 0.024); while the ASD group showed a negligible difference with slightly higher evoked gamma power over posterior (P7) regions. There were no significant hemispheric differences elucidated between ASD and control groups in evoked gamma power during baseline analysis (Figs. 14.2 and 14.3).



Fig. 14.2
Frontal evoked (peak around 50 ms) and induced (peak around 300 ms) gamma responses in children with autism spectrum disorder (ASD, N = 15) and age-matched neurotypical controls (N = 15) in Kanizsa oddball task. The control group shows higher amplitude of gamma burst to target stimuli, while the ASD group shows higher gamma response to non-target Kanizsa figures

Fig. 14.3
Early evoked gamma oscillations to target and non-target rare Kanizsa figures at the left lateral frontal (F7) and parietal (P7) sites in a group of children with autism (N = 16). Evoked gamma to non-target stimuli is comparable and even larger in amplitude than gamma response to target stimuli
Additional investigations characterizing early-stage visual processing deficits using similar ‘oddball’ paradigms maintain a large amount of significance for future ASD research and treatment. These visual tasks are capable of detecting difficulty in filtering irrelevant sensory stimuli in early stages of visual processing, and could potentially play an important role in identifying sensory processing characteristic of the autistic disorder.
14.4 Other ERP Outcomes in Children with Autism
14.4.1 Processing Novelty in Visual Oddball Task
In order to understand better the cognitive basis of visual processing abnormalities in autism, and in particular deficit of attentive orienting to novel signals, we investigated (Sokhadze et al. 2009a) the novelty-related anterior-frontal P3a component, which is recorded at the frontal sites as a response to unexpected novel event occurring in a sequence of repetitive stimuli, and other posterior ERP indices of orienting and sustained attention (N200, P3b). We investigated amplitude and latency of EPR components in a three-condition oddball task in visual modality to assess reactivity to the novelty. In this experiment we used task with variable novel distracters (target was “X” letter, standard was “O” letter, while distracters were various signs like “<”, “>”, “v”, etc.). Our hypothesis was that children with autism, as compared to controls, are more likely to show deficits in attentive orienting to novel distracters, and deficits in maintaining sustained attention to task-relevant targets due to excessive processing of distracter stimuli. We predicted that these impairments will be exhibited in a reduced parietal N200 and P3b amplitudes to targets in visual oddball. At the same time we predict delayed and attenuated P3a to novel stimuli in autistic individuals compared to controls. The visual three-stimuli oddball paradigm was aimed to test the hypothesis that individuals with autism abnormally orient their attention to novel distracters as compared to controls. A dense-array 128 channel EGI EEG system was used on 11 high-functioning children and young adults with ASD and 11 age-matched, typically developing control subjects. Patients with ASD showed slower reaction times but did not differ in response accuracy.
At the anterior (frontal) topography the ASD group showed significantly higher amplitudes and longer latencies of early ERP components (e.g., P100, N100) to novel distracter stimuli in both hemispheres (e.g., to novel distracters 4.17 vs. 2.09 μV, F = 5.65, p = 0.027). A Hemisphere (left, right) X Group (ASD, CNT) interaction effect was found (F = 5.54, p = 0.028) with the autism group showing significantly higher P100 amplitude at the right hemisphere, without any differences at the left hemisphere. Amplitude of the frontal N100 to targets at the right hemisphere was marginally more negative in ASD group compared to controls (−3.12 vs. −1.69 μV, F = 4.54, p = 0.046). Latency of the N100 was prolonged bilaterally in ASD group as compared to controls in response to novels (136.2 ms vs. 123.9 ms, F =4.79, p = 0.043). Stimulus (target, standard, novel) X Group interaction was significant (p = 0.019) with autism group exhibiting comparable latencies to each category of stimuli, whereas CNT group had faster latency to targets. The ASD group also showed prolonged latencies of late ERP components (e.g., P2a, N200, P3a) to novel distracter stimuli in both hemispheres. There was a Stimulus (target, novel) X Hemisphere X Group interaction (F = 5.78, p = 0.029) when responses to target and novel stimuli were compared. In particular, the anterior-frontal P2a was similar both to targets and novels in the ASD group and this effect was more visible in the right hemisphere. The only difference in the frontal N200 was found for latency, which was prolonged to novels in the ASD group as compared to the CNT group at the left hemisphere (F = 4.50, p = 0.048). The latency comparisons for P3a in response to targets and novels showed a Stimulus (target, novel) X Group interaction ( F = 5.30, p = 0.04), where the ASD group had delayed P3a latency to novels but not targets, whereas the CNT group had longer latency to targets but not novels. However, differences were more profound in the right hemisphere for both early and late ERP components. Our results indicated augmented and prolonged early frontal potentials and a delayed P3a component to novel stimuli, which suggest low selectivity in pre-processing and later-stage under-activation of integrative regions in the prefrontal cortices.
At the centro-parietal topography the ASD group showed significantly prolonged N100 latencies both to standard and novel stimuli (F = 5.22, p = 0.035), and reduced amplitudes of the N200 component to target stimuli. Amplitude of the cento-parietal N200 was globally lower at the right hemisphere in ASD group as compared to controls, however, a between group difference was significant only at the right hemisphere in response to novel distracters (F = 5.30, p = 0.034). We found a trend in the Stimulus (target, standard, novel) X Group interaction, which can be described as a more negative N200 to standards and novel distracters, but not to targets in the ASD group as compared to the CNT group (F = 3.62, p = 0.049). The latency of P3b to novel distracters was delayed in the ASD group (F = 4.96, p = 0.041), which was more pronounced in the right hemisphere (410 ms vs. 356 ms, F = 8.09, p = 0.012). In general, the autistic group showed prolonged latencies to novel stimuli especially in the right hemisphere. These results suggest that individuals with autism over-process information needed for the successful differentiation of target and novel stimuli (Fig. 14.4).


Fig. 14.4
Frontal (F2, FC2) ERP to target and target and novel distracters in visual oddball task in ASD and typically developing children (N = 11/per group). Children with autism show higher magnitude of N100 and N200 components and delayed P3a to novel stimuli
Neural systems in the brains of autistic patients are often inappropriately activated (Belmonte and Yurgelun-Todd 2003a; Brown 2005). In particular, abnormally enhanced sensory responses have been reported, and associated with this are deficits in orienting attention and transferring information to higher levels of processing (Townsend et al. 1996, 1999). According to Belmonte and Yurgelun-Todd (2003a, b) perceptual filtering selectivity in autism occurs in an all-or-none manner with little specificity for the task relevance of the stimulus. These authors suggest that perceptual filtering primarily depends on the control of general arousal rather than the activation of a specific perceptual system. Since in many tasks requiring attention persons with autism perform at close to normal levels (Belmonte 2000) despite generally high arousal and low selectivity, some compensatory mechanisms may be operating at a higher stage of processing to sort out the relevant stimuli from poorly discriminated background. One candidate mechanism has been suggested as the active inhibition of irrelevant distracters having passed through earlier filtering (Belmonte and Yurgelun-Todd 2003b). In general, the autistic group showed prolonged latencies to novel stimuli especially in the right hemisphere. These results suggest that autistic subjects over-process information needed for the successful differentiation of target and novel stimuli. The examination of ERP measures in novelty task holds promising potential for contributing to our knowledge of autism.
14.5 Cortical Premotor Potential in Spatial Attention Task
14.5.1 ERP and Lateralized Readiness Potential (LRP) in a Posner Cued Spatial Attention Task
Along with other executive function deficits, individuals with autism as well as individuals with ADHD present abnormalities of spatial attention. Our recent pilot study (Sokhadze et al. 2014) was aimed to understand the abnormal neural and functional mechanisms underlying attention abnormalities in autism and in ADHD by incorporating ERP and behavioral measures of spatial attention. The participants for the study were 24 high-functioning ASD and 14 individuals with ADHD who complied with ERP task requirements and tolerated EEG recording. Mean age of patients was 14.2 ± 3.4 years. The contrast group consisted of 24 age-matched (15.2 ± 3.1 year) typically developing children.
The spatial attention task was a modification of a cued Posner spatial attention task and had 2 blocks – one with horizontal, while the second with diagonal windows where target appeared either at the left or the right side. The analysis included comparison of behavioral performance (RT, accuracy) and ERP measures. In addition to the second cue stimulus (S2) locked ERPs, we analyzed also lateralized readiness potential (LRP) recorded as a difference wave between responses at motor strip (C3/C4) starting from the first cue (pre-cue S1). ERP data set was analyzed using ANOVA with within subject factors Cue Position (Horizontal, Diagonal), S1 Cue Congruence (valid, invalid), and Hemisphere (left, right) and between group factor Group (ASD, ADHD, Controls).
Reaction time (RT) analysis for ASD and typical children (CNT) showed a Congruence X Group effect (F = 7.14, p = 0.011), in particular the ASD group had similarly slower RT both in valid and invalid pre-cued conditions, while controls responded faster to correctly prompted targets. Accuracy of responses was lower in the ASD group, mostly due to more omission error rate (F = 6.17, p = 0.017). Comparison of ERPs across 3 groups (ASD, ADHD, CNT) yielded following results. Midline frontal N100 showed a target Position (horizontal, diagonal) X Pre-cue Congruence (valid, invalid) X Group (ASD, ADHD, CNT) interaction (F = 13.42, p = 0.001), where ASD group had more negative N100 amplitude during diagonal target condition regardless of congruence of cues, as pre-cue congruence had less effect in this group. In the ADHD group latency of N100 was prolonged, e.g., in a diagonal condition ADHD group had longer latency than ASD. Frontal N200 showed a Position X Congruence X Hemisphere X Group (ADHD, ASD, CNT) interaction effect (F = 4.11, p = 0.023). Amplitude was higher in ASD, more in horizontal target position, and effect was better expressed at the right hemisphere. Latency of the frontal N200 was showed significant group differences, namely shorter latency, along with lower amplitude in the ADHD (ADHD vs. ASD, F = 2.42, p = 0.018). The P3b component showed differences between ASD and CNT groups at the midline (F = 5.38, p = 0.026) in invalidly cued diagonal target condition and was significantly prolonged in the ASD group.
Most of ERP differences between controls and ADHD and ASD were observed at the frontal sites thus pointing at the possible frontal executive deficits both in autism and ADHD. Of particular interest are frontal hemispheric differences present at the pre-attentive early processing stages (N100), and less discrimination between correctly and incorrectly cued targets at the later stages of processing in autism, and globally lower magnitude and delayed latency of early frontal component in the ADHD (Fig. 14.5).


Fig. 14.5
Frontal (Fz, F2, FC2) ERP to imperative (S2) stimuli in cued Posner spatial attention task in ASD and neurotypical (NT) children groups (N = 24/per group). Children with autism show delayed latencies of N100 and P200 components in incongruent trials of the test
The lateralized readiness potential (LRP) is an index of motor processes and it is assumed that this brain potential is generated by a source within the Ml. The lateralized readiness potential (LRP, reviewed in Coles 1989; Eimer 1998; Leuthold et al. 2004; Leuthold and Jentzsch 2001) reflects the response-specific involvement of the left and right cortices of the brain. This LRP has mostly been recorded by just one pair of electrodes located at scalp locations overlying the motor cortex (C3/C4 or C3′/C4′). The LRP enables the determination of the point in time at which the activation of the motor cortex controlling one hand surpasses the activation of the motor cortex controlling the other side. On the basis of this feature, the LRP has been advocated as a tool for psychophysiological studies that can be used to index the time of response selection and the onset of hand-specific movement preparation (Coles 1989; Eimer 1998). Hand-specific movement preparation, as reflected in the LRP, can be detected, for instance, in the foreperiod of a warned RT task such as cued Posner task, when a subject knows which hand has to be moved (Leuthold et al. 2004). Moreover, it has been shown that the LRP is sensitive to covert aspects of movement preparation (Eimer 1998). In conjunction with RT (or EMG) measures, this sensitivity of the LRP to covert response tendencies has proven to be very valuable in studies on stimulus–response translation. Given the contralateral dominance and central distribution of movement-related potentials preceding self-paced and externally cued movements, it seems very likely that the different types of movement-related potentials at least share the primary motor cortex (MI) as a generator (Praamstra et al. 1999). That MI has a major part in the generation of the readiness potential is indeed well established by recordings of cortical field potentials, topographical EEG, and dipole source analyses (Cui et al. 2000; Praamstra et al. 1999).
The LRP is assumed to be related to selective response activation. It captures the asymmetric portion of the late BP preceding hand or foot movements. The LRP is computer on the basis of ERP potentials recorded prior to and during the execution of a motor response over the left and right motor cortices (C/C4 or C3′/C4′-later located 1 cm anterior to C3/C4 sites by 10–20 EEG system). The lateralization negativities are made explicit with the double substraction method, when at 1st stage C3-C4 amplitude differences are computed separately for the left and right hand responses. The differences for the right hand responses are then subtracted from difference waves for left-hand responses. As a result of these two subtractions, lateralized negativities of motor cortex are unrelated to the side of the response. There are known other methods of LRP extraction (e.g., Coles 1989, averaging method). In all methods LRP waveform reflects the lateralization of slow motor ERP activity observed prior to movement onset that is assumed to be related to a central activation of an unimanual response (Eimer 1998) Furthermore, it is important for the mental chronometry that LRP helps to determine exact point in time when sensory information starts affect motor processing and response execution. The LRP can be computed on the basis of stimulus-locked average waveforms or as a response-locked averages. In our study (cued Posner attention task) we used stimulus-locked LRP method and since S1-S2 interval was set on 1 s, we computed mean LRP and integrated LRP values for 2 windows (early 600–800 ms post S1 stimulus; and late – 800–1,200 ms post-stimulus). In our sample of ASD, ADHD and NT controls, differences at the early stages were not significant at the early stage, but became significant at the late stage of the LRP yielding significant Time (early, late) X Group interaction (F = 6.77, p = 0.012). Post-hoc analysis showed group differences at 1,000–1,200 ms post stimulus window (F = 4.81, p = 0.033) between autism and control groups. Autism group also showed more pronounced differences of LRPs in incongruent trials (Fig. 14.6).


Fig. 14.6
Lateralized readiness Potential (LRP) in incongruent trials of a cued Posner spatial attention task in three groups of children (N = 24/per group). Children with ASD have more negative LRP as compared to both ADHD and typically developing children groups
14.5.2 Audio-Visual Selective Attention Test
The purpose of our another study (Kiser et al. 2012) was to employ electrophysiological measures to study and provide observable evidence of atypical neurological multimodal sensory processing in ASD. This study used the audio-visual selective attention test and a measure of ERP and evoked gamma oscillations. It is believed that aberrations of the fundamental cortical structures, i.e., minicolnopathy of ASD subjects create a deficit in essential processing and integration of sensory modalities. We predicted that ASD subjects will display deficits in the ability to attend to and respond to rare, combined audio-visual target stimuli. It was hypothesized that because of a dysfunction in target discrimination ASD subjects will display impaired cognitive inhibition, thus exhibit hyper-excitable responses to non-targets. Furthermore, it was expected that lower ability to selectively discriminate between targets and non-targets will create delayed latency in the ERP components and induced EEG gamma responses of in the subjects with ASD.
This study was based on the premise that the previously described neuroanatomical differences in ASD – the hypotheses that ASD subjects have hyper-connected, hyper-excitable cortical regions – will be prominently observable through EEG recordings in combined audio-visual target detection task. Because of the aforementioned anatomical differences, ASD subjects were anticipated to display exaggerated responses to both target and non-targets alike eliciting early and late stage differences.
If there are deficits in single modality stimulus processing (as it was described above for visual target detection tasks), one would naturally expect there to be deficits in tasks when the individual is required to integrate information from multiple modalities. The anticipated deficiencies associated with ASD individuals may be reflections of the failure to successfully attend to and/or process multiple modalities, e.g. visual, auditory, or concurrently (O’Neill and Jones 1997). It is expected that the multimodal audiovisual tasks will more readily elicit observable processional sensory deficits (Marco et al. 2011). In accordance with the previously mentioned neuroanatomical observations linked to ASD, it was also likely that abnormal ERPs and gamma oscillations associated with multimodal sensory processing might be the results of altered minicolumn morphology and decreased inhibition (Baruth et al. 2010a, b; Sokhadze et al. 2009a, b, 2010a, b). The aim of this study was to establish any possible differences in concurrent audio-visual processing between in individuals with autism and typically developing control group.
Twelve ASD subjects, 12 control subjects, completed the tasks of this protocol. The ASD group was comprised of 4 female and 8 male participants while the control group consisted of 3 female and 9 male participants. Each participant performed 5 target detection tasks during a single ERP recording session. Total Task time lasted approximately 20 min. Each task consisted of a block of (100) trials with a break every (50) trials. Students were instructed to press a key for the specific target in each block. Stimuli were presented pseudo randomly with a target to standard ratio of (20:80). Stimuli had (150 ms) duration with a random inter-stimulus interval between 1,000 and 1,250 ms. In combined audiovisual task subjects were presented with concurrent visual and auditory stimuli, and were instructed to only respond when the visual target “X” and auditory target “low tone” were presented simultaneously.
Tailored algorithms generated in MATLAB were used to extract measures of gamma frequency from the EEG recordings. The extracted data was then processed using SPSS to assess between group differences for power (μV2), hemispheric activity, response to visual, auditory, and combined audiovisual target conditions (Baruth et al. 2010a; Baruth et al. 2011; Gross et al. 2012). ANOVA was used to analyze the following factors within all participants: (1) Modality (Visual, Auditory, Combined), (2) Stimulus (target, non-target), (3) Hemisphere (left, right), (4) Group, (ASC, control). Statistically significant findings were found in the latency of the N100 in the frontal region in response to the combined audiovisual non-target: the ASD group showed significantly shorter N100 latencies to a non-target stimulus (F = 8.93, p < 0.01). In the tempo-parietal region there was a significant difference in the amplitude of the N200 component in response to the audiovisual non-targets (F = 4.36, p = 0.049).
The parietal P300 showed a significant difference in amplitude response to audio-visual targets (F = 7.66, p = 0.01), manifested as higher P300 in autism. Upon collapsing the activities measured at frontal left and frontal right as well as the responses to targets and non-targets of the auditory and audiovisual stimuli, a significant hemispheric difference was found in gamma activity between ASD subjects and neurotypical controls. The individuals with autism displayed a significant bias towards right frontal hemispheric gamma activity (F = 4.93, p = 0.04). The ASD group shows increases in gamma activity within 0–50 ms and as well as the 150–200 ms across all modalities in the parietal region additionally showing frontal increases in activity for the combined condition (Fig. 14.7).


Fig. 14.7
Gamma frequency oscillations in response to combined audio-visual stimuli condition in selective attention task. Children with autism (N = 12) show similar power of the early (evoked) and late (induced) gamma oscillations (35–45 Hz range) in response to both target and non-target stimuli
14.6 Discussion and Conclusions
For high functioning ASC individuals it generally appears that higher cognitive inhibitory control remains intact, however it also appears that the parietal and frontal regions of these patients display increased activity related to standard and novel stimuli (Baruth et al. 2010b; Sokhadze et al. 2009a). It has been conjectured that there could be two reasons for the observed cortical hyperactivity: ASD subjects may have atypical neuroanatomical development, or ASD subjects may employ unconventional compensatory cognitive processing techniques that require more cortical activity (Sokhadze et al. 2009a). Tannan et al. (2008) found that in a sensory discrimination task, ASD individuals failed to adapt to the changes in the stimulus while controls did, and the authors suggested that the lack of adaptation is indicative of the hyper-excited network based on ineffective GABAergic interneuron network mediation.
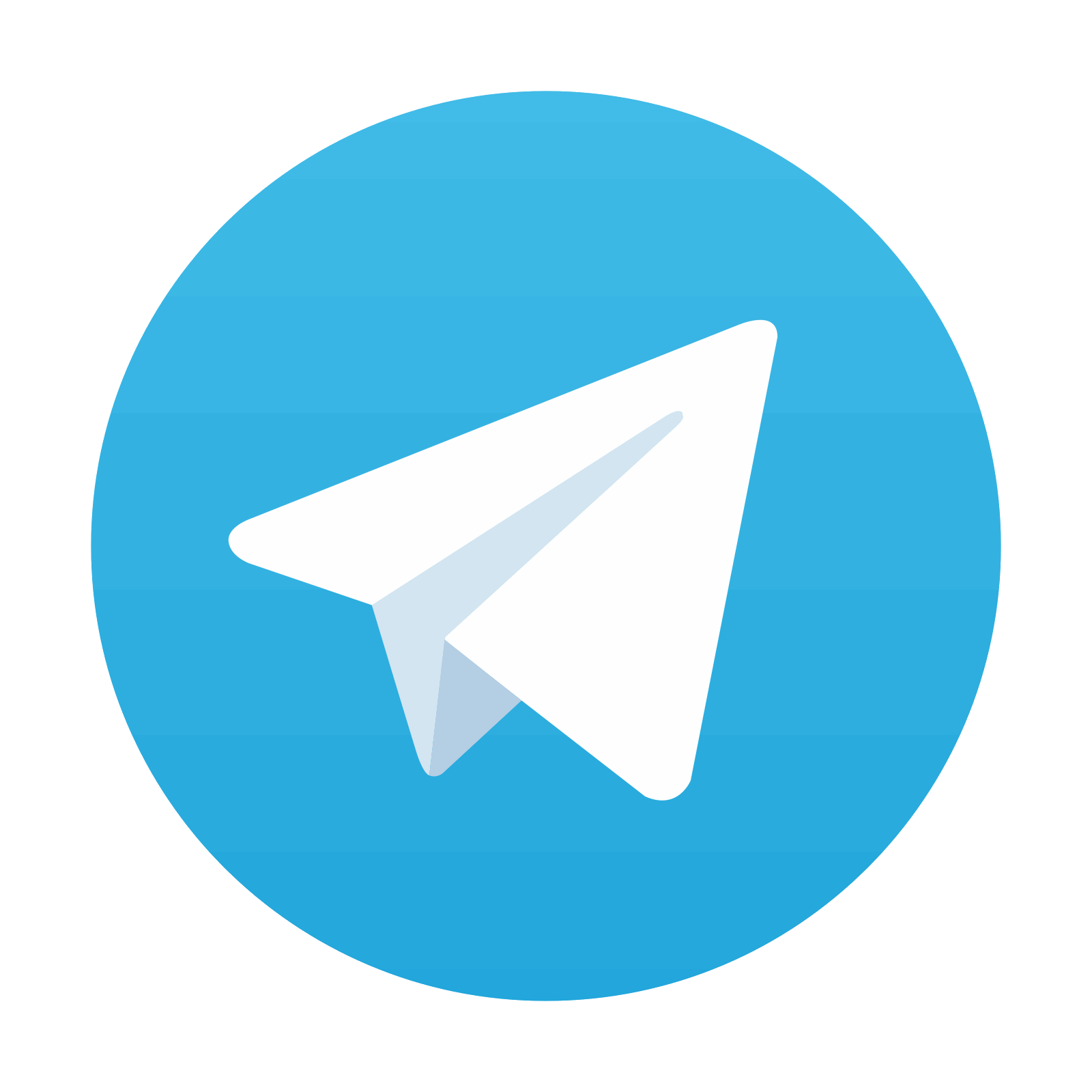
Stay updated, free articles. Join our Telegram channel

Full access? Get Clinical Tree
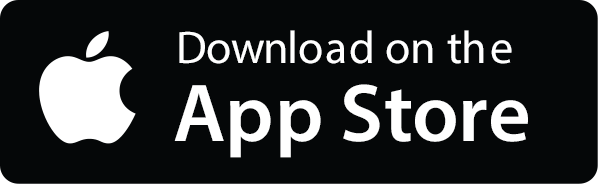
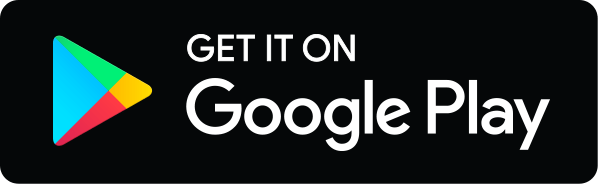