For many decades, the central nervous system (CNS) of living individuals could be examined only indirectly, for example by using x-rays to study changes in the bones surrounding the CNS or the blood vessels around or within it. In addition, these imaging studies involved projecting all the x-ray density under investigation in the head (a three-dimensional structure) onto a two-dimensional sheet of film. As a result, the images of structures actually separated in space (e.g., the middle cerebral and anterior cerebral branches in Fig. 9.17A ) are superimposed on each other in these studies.
The past 40 years have seen revolutionary changes in clinical imaging, partly a result of the use of computers to reconstruct two-dimensional “slices” at various levels of a patient’s head (i.e., tomography ) and partly a result of the ability to construct images based on parameters other than x-ray density.
The most commonly used clinical imaging techniques at present are x-ray computed tomography ( CT ) and magnetic resonance imaging ( MRI ). CT provides images based on x-ray density, so structures that attenuate x-rays, such as bone, appear light; areas filled with air or cerebrospinal fluid, which do not attenuate x-rays as much, appear much darker. Appropriate techniques can accentuate brain, bone, or blood ( Figs. 9.1 to 9.3 ). MRI ( Fig. 9.4 ), in contrast, provides images based on chemical concentrations (most commonly emphasizing the concentration of free water). This chapter provides a series of examples of the use of CT and MRI to demonstrate normal anatomy in clinical imaging. In addition, although traditional angiographic techniques are no longer used very often, they still yield the most highly detailed images of the cerebral vasculature, so examples of angiograms are also provided.





















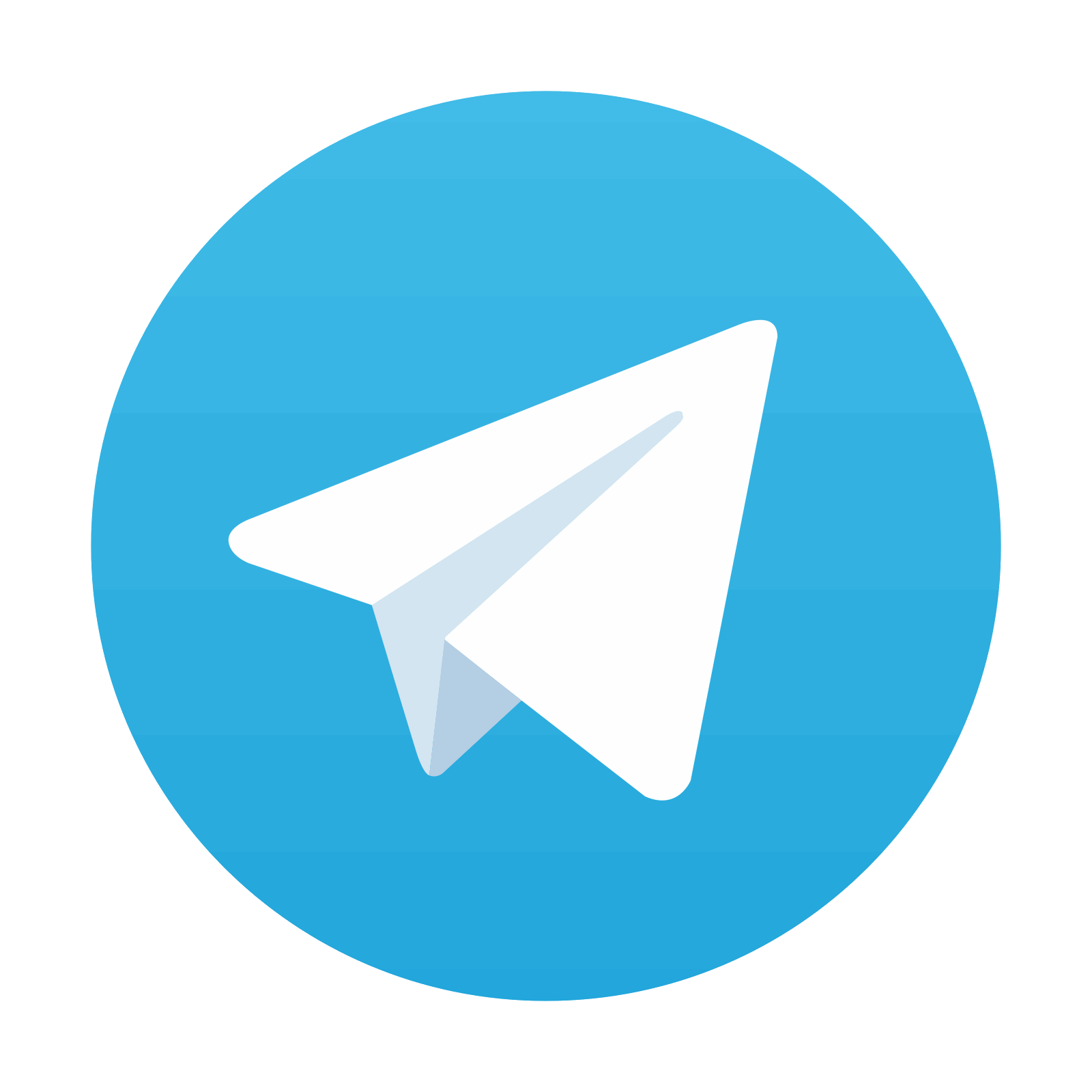
Stay updated, free articles. Join our Telegram channel

Full access? Get Clinical Tree
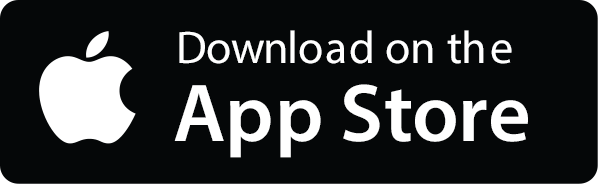
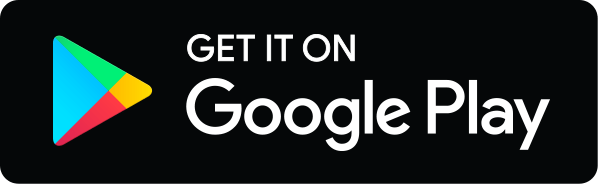
