20 Clinical Neurophysiology of Tethered Cord Syndrome and Other Dysraphic Syndromes Robin L. Gilmore, Sun Ik Lee, and John Walsh Tethered cord syndrome is a complex developmental malformation, with the underlying pathological anomaly being a dura mater defect or dural schisis. The dural schisis may not be the only developmental defect, but it is probably the most basic one1 and one that occurs more commonly than is generally recognized.2,3 Establishing the diagnosis and assessing the extent of functional disability is often difficult but may be aided considerably by the use of several clinical neurophysiological studies, including somatosensory evoked potentials (SSEPs),4–7 urodynamics with sphincter and pelvic floor electromyography (EMG),8,9 and anal sphincter EMG and pressure monitoring for intraoperative use.8,10 This chapter reviews the contributions of these techniques to the diagnosis and management of patients with tethered cord syndrome. To convey an appreciation for the use of these techniques, the chapter includes a discussion of the relevant developmental anatomy, specifically the development of the neural pathways, assessed by these techniques. In addition to the diagnostic studies, the multimodality monitoring of sensory and motor systems as well as reflex circuits have recently been emphasized as the intraoperative diagnostic tool in many publications.11–18 These monitoring methods have been shown to reflect not only acute but also chronic insults to the cauda equina or spinal cord. The degree of injury paralleled the degree of electrophysiological changes in an animal study.19 Thus the spinal cord and cauda equina have been successfully protected by the aid of intraspinal monitoring. The stimulation of the filum has been claimed to be useful when the normal anatomy is distorted by pathological structures.15,17 The spinal cord is freer within the vertebral canal than is the brain within the cranium. The spinal dura mater is composed of dense connective tissue with few elastic elements derived from paraxial mesoderm.20 It is separated from the vertebral internal periosteum by the epidural space, which contains fat cells, blood vessels, and loose connective tissue. The spinal cord needs to be completely free from the vertebral column during development because the rates of growth of the two structures are different. Early in development, the caudal region of the spinal cord undergoes a progressive upward displacement or retrogression relative to the caudal vertebral column.21 The conus medullaris, which is initially at the coccygeal level in the 30 mm embryo, ascends through the S4 level in the 67 mm embryo, to the L3 level by birth (40 weeks conceptional age),1 and to the adult L1–2 level by 49 to 50 weeks conceptional age.22 The subarachnoid space elongates progressively to accommodate the elongating spinal nerve roots and filum terminale. The filum terminale must also elongate because the cord retains its original coccygeal attachment through this structure. Early dural schisis (below L3) through which the spinal cord comes in direct contact with subcutaneous tissue lends to tethering of the spinal cord to this tissue. Later, subcutaneous adipose tissue penetrates and expands into the intraspinal space.1 This results in a low conus medullaris and a short, thick filum terminale. It is possible that the adipose tissue is stimulated by its direct contact with neural elements and the abundant arachnoidal vascularity through the dural schisis. Evoked potentials recorded from the body’s surface are either near field or far field in nature; that is, the generator source is close or distant to the site of recording. The generators may be in gray matter or white matter. Generators in gray matter produce postsynaptic potentials (PSPs), which may be near field or far field. Near field PSPs are probably responsible for cortical components of SSEPs. White matter generates compound action potentials (APs), which are propagated through fiber tracts. The latencies of the propagated APs increase proportionately to the distance from the point of stimulation and hence are dependent on the recording electrode position. These are recorded only in close proximation to the fiber tract itself and thus are termed near field potentials (NFPs). Because they are close to the site of origin, the amplitude is relatively large (> 1 µV). Other evoked potentials may be recorded at long distances from the point of propagation and are generated when a traveling impulse (signal) passes through a certain anatomical site or fixed point along the nerve. These are called far field potentials (FFPs). It was previously considered that FFPs reflected the approaching volley recorded beyond the point of termination of an active fiber.23 More recently, it has been suggested that FFPs are generated because of abrupt changes in the geometry of tissue surrounding the nerve,24,25 a change in the medium through which the volley is transmitted, or a change in the direction of the fibers.26 In summary, NFPs have a specific distribution (topographic specificity), latencies that vary according to the recording electrode placement, amplitudes > 1 µV, and generally negative polarity. FFPs have a diffuse distribution, fixed latencies, amplitudes < 1 µV, and polarity that probably reflects a volume-conducted positivity. Potentials are labeled according to polarity and mean latency from a sample of the normal population. As one would expect, latencies change with body growth and nervous system maturation. Hence, labels differ between children and adults. There are less standard evoked potential component designations for posterior tibial nerve (PTN) SSEPs than for median nerve SSEPs.25 For the purpose of discussion of generators of SSEP-PTNs, adult terminology is used, with child or infant notation following in parentheses. Following PTN stimulation, electrodes over the popliteal fossa record the electronegative peripheral nerve action potential N8 (N5). Electrodes over the lower spine record two electronegative potentials: the N19 (N11) and the N22 (N14). The N19 (N11) represents the afferent volley in the cauda equina.27 The N22 (N14) is a stationary potential and probably reflects postsynaptic activity of internuncial neurons in the gray matter of the spinal cord.27 Electrodes over the cervical spine record another later stationary potential: the N29 (N20). This component may reflect postsynaptic activity in the nucleus gracilis.28 The P37 (P28) is the first major localized recorded component on the scalp. It reflects the ipsilaterally oriented cortical surface electropositivity, whereas the electronegative end of the dipole may be recorded contralaterally.29,30 There is a great deal of inter-subject variability in the topography of the P37 (P28) in adults31 and especially in children.32–34 This is probably related to the known anatomical difference in the location of the primary sensory area for the leg.35 When the leg area is located at the superior edge of the interhemispheric fissure, the cortical generator for P37 (P28) is vertically oriented and its amplitude is maximally close to the vertex. When the leg area is located more deeply in the fissure, the cortical generator is more horizontally turned and the P37 (P28) projects ipsilaterally.30,36 SSEP is a valuable tool in detecting spinal cord anoxia secondary to inadvertent involvement of supplying vessels around the S1 level. Kothbauer et al13 used tibial SSEP without any surgical complications. Because the tibial nerve covers predominantly L5 and S1 dermatomes and leaves higher and lower segments relatively uncovered, these authors advocate additional dermatomal SSEP and pudendal monitoring. Monitoring the pudendal nerve activity (S2-S4) had been a promising technique for preventing damage to the sensory roots during the surgery within the cauda equina because it provides additional information at lower sacral levels below S1 that is associated with bowel, bladder, and sexual dysfunction.37 In 2004, Kothbauer14 provided an update article in which sensory potentials evoked by tibial or pudendal nerve stimulation were recorded from the dorsal columns via an epidurally inserted electrode and/or from the scalp as cortical responses. Monitoring of the motor system was achieved with motor evoked potentials recorded from limb muscles and the external anal sphincter. Amplitudes and latencies of these responses are then interpreted. The bulbocavernosus reflex, with stimulation of the pudendal nerve and recording of muscle responses in the external anal sphincter, is used for the continuous monitoring of reflex circuitry.10,13–15 Two decades ago, the usual explanation for the neurological deficit associated with tethered cord syndrome was the effect of traction in preventing the ascent of the spinal cord within the spinal canal during growth. However, Barson22 pointed out in 1970 that the spinal cord does not ascend significantly after birth. The incongruity in observations is due to the fact that the spine grows most rapidly during embryogenesis and after puberty (during teenage growth spurts), whereas symptoms of tethering are most often observed in early childhood (age 3 to 10 years). James and Lassman reported a clinical case in which, during a postmortem examination, a small bony septum from the midline of the laminae of L3–4 to the underlying vertebral body was found in an aged woman. She had never had any neurological deficits. Had there been significant ascension of the spinal cord after birth, she would have had neurological deficits, so the authors argued.38 Although this was not necessarily so because issues of the divided cord segments rejoining below the spur and the size of the cleft between cord segments (small or large) were not addressed, the concept that the spinal cord ascended postnatally and produced neurological deficits by traction alone in tethered cord syndrome seemed untenable. Yamada et al39 examined the mitochondrial oxidative metabolic changes in the spinal cord before and after subjecting it to stretching. Using reflection spectrophotometry, they monitored in vivo changes in the reduction:oxidation (redox) ratio of cytochrome a,a3 in animal models and in human tethered spinal cords (Fig. 20.1 ). They found a marked metabolic and electrophysiological susceptibility of the lumbosacral cord subjected to hypoxic conditions, especially under traction with hypoxic or electrically stimulating energy stress (Fig. 20.2).39,40 They concluded that symptoms and signs of tethered spinal cord were associated with lumbosacral neuronal dysfunction and that this dysfunction is possibly due to impairment of mitochondrial oxidative metabolism. This is supported by the associated evoked potential changes (see later discussion). Most pediatric neurosurgeons now believe that the chronic stretch on the cord produced by tethering is an essential part of the problem but that superimposed insults such as acute flexion episodes or cord hypoxia accentuate symptoms to become more prominent. Fig. 20.1 Redox changes during hypoxia in one group of the human tethered cords (type 1). No redox change is seen before untethering (dotted line), but a reduction similar to that in normal cat cords is noted after untethering (solid line). No reduction occurs while the cord is temporarily retethered (interrupted line). FiO2, fraction of inspired oxygen. (From Yamada S, Zinke DE, Sanders D. Pathophysiology of “tethered cord syndrome.” J Neurosurg 1981;54:494–503. Reprinted with permission.) Kang et al41 tethered and untethered the cords of immature kittens and studied the effects of these manipulations on regional spinal cord blood flow, clinical features, and SSEPs. They found that cord tethering caused decreases in regional spinal cord blood flow in the distal spinal cord close to the site of tethering. The decreases in regional spinal cord blood flow (rSCBF) became progressively worse over the weeks following the tethering (Fig. 20.3). Untethering of the cord led to an increase in the rSCBF if the untethering occurred by 2 weeks after tethering. Delaying the untethering until 8 weeks caused failure of the rSCBF to recover the normal level. Changes in the evoked potential occurred when rSCBF fell below 14 mL/100 g/min. The decrease in rSCBF had occurred by 2 weeks after tethering. Fig. 20.2 (A) Normal cord potentials in response to dorsal root stimulations. IMS: from the posterior column; N1a: from the afferent terminals; N1b: from the interneurons of the first order; N2: from the interneu-rons of the second and third orders. (B) Marked change in the cord with traction of 5 g. (From Yamada S, Zinke DE, Sanders D. Pathophysiology of “tethered cord syndrome.” J Neurosurg 1981;54:494–503. Reprinted with permission.) Fig. 20.3 Changes in somatosensory evoked potential (SSEP) were observed if regional spinal cord blood flow (rSCBF) was below 14 mL/100 g/min. Animals untethered after 2 weeks showed normal SSEP, which corresponded with an increase in rSCBF. (From Kang JK, Kim MC, Yim DS, et al. Effects of tethering on regional spinal cord blood flow and sensory-evoked potentials in growing cats. Childs Nerv Syst 1987;3:35–39. Reprinted with permission.) Electrophysiological studies that help with diagnostic formulation include SSEPs after peroneal nerve stimulation (SSEP-PN), after pudendal nerve stimulation (SSEP-PuN), and after posterior tibial nerve stimulation (SSEPPTN), bulbocavernosus reflex (BCR) responses, and urodynamics with sphincter and pelvic floor EMG. It has been a decade since SSEPs were first used to evaluate patients with occult spinal dysraphism. Cracco and Cracco42 recorded scalp and spinal responses after peroneal stimulation over the cauda equina and rostral spinal cord in adult and child control subjects (Fig. 20.4). These spinal potentials consisted of low-amplitude triphasic waves over the cauda equina and larger potentials over the caudal spinal cord. Scalp potentials had latencies of 30 to 34 millisecond for electropositive components and 40 to 45 millisecond for electronegative components. In patients with sacral lipomas and no or minimal neurological findings, spinal potentials normally recorded over the lower thoracic spine (T9–12) were recorded over the lumbar spine, suggesting caudal displacement of the spinal cord (Fig. 20.5). In children with more extensive neurological findings (foot deformities, neurogenic bladders), relatively normal potentials were recorded over the cauda equina, and cerebral potentials were absent.42 Others have subsequently verified the diagnostic value of SSEPs.43,44 One group reported a patient who had postoperative SSEP-PN studies that were slightly improved compared with preoperative studies, and the patient had improved clinically.43 The authors have systematically studied children and young adults with tethered cord syndrome using SSEP-PTNs. Because SSEP-PN scalp and spine components are lower in amplitude than those produced by PTN stimulation45 and because the topography of the scalp component of SSEP-PN is more variable than that of SSEP-PTN, SSEP-PTNs were used rather than SSEP-PNs for evaluation in children suspected of having tethered cord syndrome. Clinical, myelographic, and operative studies were prospectively evaluated in 22 consecutive patients, aged 18 months to 22 years, with symptoms of tethered cord syndrome.7 Ten had previously undergone repair of lumbosacral meningomyelocele. In 19 patients, the diagnosis was established radiologically and/or intraoperatively. In three patients with clinical symptoms but without radiographically demonstrable lesions, SSEP-PTNs were normal.
Developmental Anatomy of the Spinal Cord
Neuroanatomy of Somatosensory Evoked Potentials
Posterior Tibial Nerve Somatosensory, Motor, and Reflex Evoked Potentials
Pathophysiology of Tethered Cord Syndrome
Diagnostic Clinical Neurophysiological Studies
Somatosensory Evoked Potentials
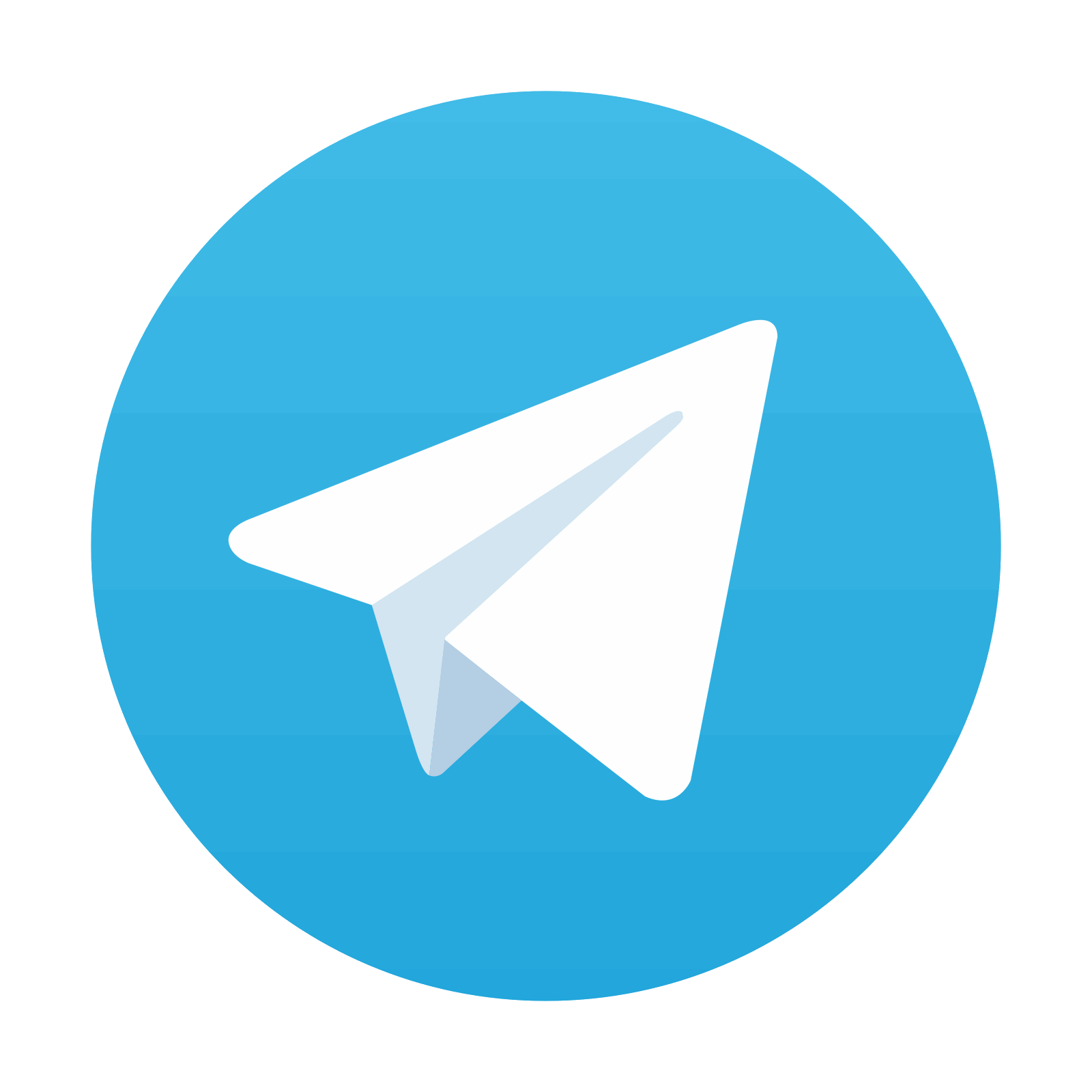
Stay updated, free articles. Join our Telegram channel

Full access? Get Clinical Tree
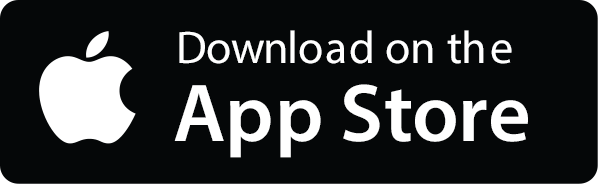
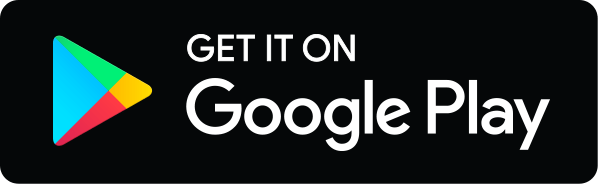