© Springer International Publishing Switzerland 2017
Norbert Weidner, Rüdiger Rupp and Keith E. Tansey (eds.)Neurological Aspects of Spinal Cord Injury10.1007/978-3-319-46293-6_2626. Clinical Trials and Spinal Cord Injury: Challenges and Therapeutic Interventions
(1)
School of Kinesiology, University of British Columbia, Vancouver, BC, Canada
(2)
University of British Columbia, Vancouver, BC, Canada
(3)
International Collaboration on Repair Discoveries (ICORD), University of British Columbia, Vancouver, BC, Canada
Abstract
In SCI, like other diseases, randomized control trials (RCTs) represent the gold standard towards establishing the efficacy of novel treatments. RCTs in SCI present with their own unique set of challenges, including considerable injury heterogeneity, low numbers, variable outcome measures, and ethical concerns. Many of these challenges are compounded by difficulties in translation between animal models and the real human condition. As a consequence, current treatments to improve neurological outcomes after SCI remain limited. In this chapter, we detail many of the challenges of conducting RCTs in SCI, whilst exploring progress of several potential therapies to protect and repair nervous system tissue in humans.
26.1 Overview: Basic Premise of Acute Interventions to Improve Neurological Outcomes for Individuals with SCI
In an attempt to overcome the limited capacity for regeneration in the central nervous system (CNS), acute therapeutic interventions generally fall into one of two categories: neuroprotection and neural regeneration. Following primary damage to the spinal cord, a cascade of biochemical events triggers secondary injury to spared white and grey matter (see chapter 19). Towards preventing the cascade of secondary injury mechanisms, a window of opportunity for neuroprotection is thought to emerge in the initial hours to days after injury [51]. While preventing secondary injury represents a reasonable target for improving neurological outcomes, full restoration of sensory and motor function requires regeneration in the spinal cord [54]. Emerging regenerative therapies are focused on promoting functional recovery by inhibiting factors that prevent endogenous repair or biochemicals that promote axonal sprouting (e.g. short-distance growth) [23]. The goal of this chapter is to provide an overview of studies supporting acute neuroprotective and regenerative interventions as effective strategies to enhance neurological outcomes after spinal cord injury (SCI).
26.2 Challenges of Spinal Cord Injury Trials
Before reviewing interventions conducted in the field of SCI, it is important to briefly describe the challenges facing researchers and clinicians performing clinical trials. While not necessarily unique to SCI, these challenges represent a significant barrier to detecting therapeutic benefits of any intervention and linking neurological improvements with long-term functional benefits.
26.2.1 A ‘Moving Target’: Effect of Spontaneous Neurological Recovery
SCI is a devastating neurological condition, often characterized by severe and lifelong impairments. Similar to other traumatic neurological conditions (e.g. stroke), SCI is associated with some degree of neurological and functional recovery. Neurological recovery is most evident in the initial days to weeks post-injury and is characterized by a rapid increase in muscle strength for up to 6–9 months and generally plateauing by 1 year [14, 41, 56, 66]. Neurological recovery is best predicted by the initial severity of damage in the spinal cord, as individuals with less severe injuries are capable of greater recovery than individuals with more severe injuries [14]. Neurological recovery is fundamental to the return of functional independence, such as self-care and ambulation. In terms of designing clinical trials, the benefits of a therapeutic intervention must be distinguishable from naturally occurring recovery of function. Moreover, it requires careful consideration in terms of study design, ensuring that injury severities are equally distributed between treatment arms of a clinical trial [55]. This can be problematic and is related to our second challenge: low numbers of suitable patients.
26.2.2 Low Numbers: SCI as an ‘Orphan’ Disease
Thankfully, SCI is not a common neurological condition and is recognized in Europe and the United States as an ‘orphan’ disease. While providing incentive for industry partners to target SCI (e.g. extended patent protection), the low incidence of acute SCI makes performing a clinical trial very challenging. High heterogeneity in injury severity leads to variable neurological recovery profiles, further exacerbating this problem [55]. This, in addition to other factors, prevents individuals with SCI from being eligible to participate in trials and creates a ‘funnel effect’ in which a large number of potentially eligible participants are significantly reduced by inclusion/exclusion criteria. As an example, 1,816 patients were prescreened for a recent phase 2 multicentre study examining the acute neurological effects of autologous cellular therapy in individuals with SCI [35]. After excluding subjects for being more than 14 days post-injury, having the incorrect injury characteristics and/or failing to meet MRI criteria, the final recruitment number was 50. Of these 50 individuals, only 32 completed the follow-up assessment at 12 months [35].
26.2.3 Enrolment and Assessment into an Acute Clinical Trial: Very Early Interventions May Be Necessary
Low subject numbers for clinical trials are particularly problematic for neuroprotective strategies, which necessitate very acute interventions (i.e. initial hours after SCI). Several practical constraints limit recruitment, including patient transportation to a participating centre, acquiring informed consent and confirming that a participant meets all inclusion criteria. Substantial barriers exist for each of these requirements, including ethical debates as to whether an individual with acute SCI can reasonably be expected to provide informed consent (see Sect. 26.2.5 below). Of paramount importance, detecting efficacy of an acute intervention is contingent on the validity and reliability of the acute neurological assessment. This is important both in terms of enrolling the correct subjects and establishing baseline measurements that will serve as anchors from which to measure change. In practice, an accurate (i.e. reliable) neurological exam may be very difficult to perform in patients with very acute SCI (<72 h), related to spinal shock, co-concomitant injuries, ventilator use or the influence of drugs or alcohol in the very early stages [18].
26.2.4 Neurological Outcome Measures: What Is the Best Outcome to Assess Efficacy?
As already described, an accurate neurological examination soon after injury is integral for assessing subsequent neurological recovery. Equally important, neurological outcomes need to be sensitive to subtle changes, yet representative of important changes in function. The selection of different neurological outcomes may vary depending on the phase of study and range from neurophysiological, e.g. somatosensory evoked potentials (SSEPs), to a more conventional examination of muscle strength and sensory function (i.e. the International Standards for the Neurological Classification of Spinal Cord Injury (ISNCSCI)) [55]. The incorporation of neurophysiological tools is important for detecting subtle changes in neurology, which may go undetected according to other, more subjective measures. Neurophysiology may also play an important role in early phases of study, supporting a potential mechanism and substrate for neurological repair and regeneration (e.g. decreased latencies of SSEPs as an indication of remyelination (see chapter 11)). Several outcomes can be derived from the ISNCSCI and have been used to measure efficacy of acute therapeutics, including changes in total motor score (i.e. upper and lower extremities), and the American Spinal Injury Association Impairment Scale (AIS) grade conversion [55]. Changes in motor levels also have been proposed as a viable clinical trial endpoint among individuals with tetraplegia. Motor level recovery may be particularly important among individuals with cervical sensorimotor complete injuries, where recovery of segmental muscle strength near the injury site may precipitate significant improvement in hand function [41]. Several prominent issues with the ISNCSCI remain for applications in clinical trials. For example, information regarding the extent of neurological improvement that is considered ‘minimally clinically important’ is lacking [64]. This complex issue requires delicate weighting of potential benefits of neurological improvements with functional outcomes, as well as an analysis of the potential cost to the individual (i.e. side effects).
26.2.5 Ethical Issues: Informed Consent and Potential Risks
There is an ongoing debate as to whether an individual sustaining an acute SCI can provide informed consent to participate in a clinical trial [17]. This is a barrier to recruitment and worthy of serious consideration. Ethical issues around this topic stem from the capacity of acutely injured individuals to make decisions and process the relevant information regarding potential risks, rewards and long-term implications (e.g. potential to be excluded from other clinical trials) and therefore knowledgably consent or decline participation [17]. Other ethical issues arise for invasive treatments in SCI (e.g. ‘first-in-man’ therapies) and trials where a true placebo arm might be unethical and/or introduce unnecessary risk or harm [35, 60]. There is a particular concern regarding the possibility that some cellular therapies may lead to tumour growth and that strategies promoting plasticity may lead to the development of neuropathic pain.
26.2.6 Translation from Animal Models to Humans with Spinal Cord Injury: What Is the Right Model?
Prior to application in human clinical trials, an intervention is required to have a demonstrated history of safety and efficacy in animal models of SCI (see chapter 25). Animal models of SCI have included a variety of experimental injuries (e.g. contusion or transection) in mice, rats, cats, dogs, swine and non-human primates [54]. Despite substantial preclinical evidence of neurological improvements from neuroprotective or reparative therapeutic interventions, no treatment has transitioned successfully into humans (note: more on the specifics of failed clinical trials below). However, challenges emerge within animal studies, particularly with regard to the replication of preclinical results, even within the same species and/or similar model conditions [50, 52]. This lack of reproducibility in seemingly identical animal models can occur for a variety of experimental and biological reasons [52]. The assessment of functional recovery within and across species can vary widely, and even commonly accepted measures (such as the Basso, Beattie, and Bresnahan (BBB) scale for hindlimb recovery in rat models) may be improperly conducted or subjectively interpreted [24]. Other sources of variation across studies include lesion severity, strains of animal and behavioural influences (e.g. a stressful environment) [24]. These failures have led some investigators to suggest that animal models do not accurately predict whether a therapy will be effective in humans. However, the translational path to clinical studies is not ‘smooth or well travelled’. Among suspected translation problems are the human condition being more heterogeneous than experimental SCI, differences in the natural history of recovery as well as the appropriate design of study protocols and outcome measures [16].
26.3 Past and Current Interventions in Spinal Cord Injury
Despite the numerous challenges, researchers and clinicians have embarked on several acute randomized clinical trials to improve long-term neurological outcomes after SCI (see also Chapter 20). Below we have provided a summary of several prominent investigations that have applied an acute neuroprotective or regenerative intervention in humans with SCI.
26.3.1 Neuroprotection
26.3.1.1 Surgical Decompression
Surgical decompression following SCI is performed to reduce pressure on the spinal cord and involves the removal of the bone as well as the instrumentation of the vertebrae to stabilize the spinal column and prevent further injury (see chapter 7) [51, 63]. Although successful in animal models, surgical decompression in humans has generated more variable outcomes. In terms of neurological recovery, retrospective studies have largely confirmed the safety of surgical decompression but provided limited evidence of efficacy [62]. An important caveat to safety is that postsurgical interventions may compromise spontaneous neurological recovery [53]. Prospective observational studies have found more encouraging evidence for surgical decompression after SCI. A recent multicentre study found that early (<24 h) surgery was associated with reduced length of stay in a hospital and greater neurological recovery compared to late decompression (>24 h) [21]. Neurological recovery was defined in this study as an improvement by two AIS grades at 6 months post-injury. However, an important limitation of this study is that individuals were not randomized to early and late decompression. This obvious limitation means that other factors such as the severity of concomitant trauma along with SCI or longer time to stabilize a person for surgery may confound the benefits of acute surgical decompression. The only randomized clinical trial reported so far only demonstrated a significant effect on neurological recovery for very early decompressive surgery (<8 h) compared to very late (3–15 days) surgical intervention in a small (n = 27) patient study [11]. Nevertheless, based on these observations, surgical decompression now represents a standard of care for many individuals with acute SCI across many trauma centres in Canada, the United States, and Europe.
26.3.1.2 Therapeutic Hypothermia
Hypothermia has been proposed as a neuroprotective strategy based on a potential application to slow metabolism and thus reduce a variety of secondary injury mechanisms, including decreasing free radical generation, inhibiting excitotoxicity and apoptosis, ameliorating inflammation, preserving the blood-spinal cord barrier, inhibiting astrogliosis, promoting angiogenesis as well as decreasing axonal damage and encouraging neurogenesis [61]. In rats, systemic moderate hypothermia (32°C) initiated 2 h after an ischemic SCI improved behavioural outcomes and increased tissue sparing [48]. Similar behavioural and histological outcomes have been reported for contusion models of SCI, although hypothermia has been applied at considerably earlier time points (e.g. 5–30 min post-injury) [46, 65]. A systematic review of studies investigating the use of hypothermia in humans indicated that there have been encouraging results with regard to neurological recovery so far; however, this review only spanned five articles for a total of 70 people with SCI [1, 15]. A large, multicentre randomized clinical trial has yet to be performed for hypothermia.
26.3.1.3 Pharmacological Interventions
Methylprednisolone
The application of corticosteroids to reduce secondary inflammation represents the most extensively tested neuroprotective intervention examined to date for human SCI. Based on animal studies, corticosteroids (i.e. methylprednisolone [MP]) became widely used in humans to improve neurological outcomes after SCI. In the first multicentre double-blind randomized controlled trial (RCT), initiated in 1979, 330 patients with acute SCI were administered low and high doses of MP [4]. The study compared two different dosages with no control group and yielded no beneficial effect on neurological function at 6 or 12 months. Based on new data from animal models, indicating a requirement for even higher dosages to have a beneficial neurological effect, a second larger, prospective, placebo-controlled RCT was initiated. In response to MP administered within 8 h of injury, improved neurological function (i.e. sensory and motor scores according to the ISNCSCI) was observed compared to placebo [5]. The timing and dosages from this study were then adopted as the ‘standard of care’ [9, 42, 54]. The National Acute Spinal Cord Injury Study (NASCIS) III randomized, double-blind, multicentre trial (n = 499) was then performed without placebo in order to refine the ideal duration of treatment [7]. They found that administering MP within 3–8 h after injury and continuing for 48 h improved motor function at 6 weeks and 6 months post-injury, though not at 1 year. The NASCIS studies used motor and sensory index scores as primary endpoints, and NASCIS III also incorporated a functional outcome (i.e. the Functional Independence Measure) [5–7]. These studies spurred other RCTs examining the effects of MP, which produced uncertain or mixed results, in addition to growing criticisms regarding the claims and methodologies (e.g. skewed groups, complex weighting scheme, post hoc analysis) of the original NASCIS studies. Also of major concern were the documented adverse effects of MP treatment, including immunological compromise, sepsis, pneumonia, gastrointestinal and pulmonary complications and myopathy [9, 42, 54]. As a result of these concerns, as well as a growing lack of evidence supporting efficacy, MP use in SCI began to fade [42, 51]. The three NASCIS studies included a total of 1242 participants [5–7]. Interestingly, for a time, MP was widely adopted as a treatment even though it was never approved by a regulatory agency as a treatment for acute SCI.
GM-1 Ganglioside (Sygen)
Monosialotetrahexosylganglioside (GM-1) is a naturally occurring ganglioside found in human cell membranes, believed to have neuroprotective and regenerative properties [27, 54]. A small (n = 37) double-blind pilot RCT, examining the effects of GM-1 on neurological recovery after acute SCI, reported a significant improvement in both the Frankel scale and the American Spinal Injury Association (ASIA) motor score with no associated adverse effects [28]. The safety and early success of this pilot study propelled a much larger phase III multicentre trial (n = 760), which began in 1992, and compared two dosages to a placebo control. A priori, a two-grade improvement from baseline in the Modified Benzel Scale (a scale similar to the AIS grades but with additional grades to more clearly identify improvements within the mildest severity of SCI) was chosen as the primary outcome measure. Six months after SCI was selected as the primary trial endpoint [27]. Despite the encouraging evidence from the pilot study, this larger RCT showed no significant difference between those treated with GM-1 and the placebo control group. Although the results were disappointing, the study introduced many important criteria to improve the rigour of human SCI studies, including a defined a priori endpoint, improved outcome definitions and ongoing assessment for the training and reliability of outcome examinations [42].
Autologous Activated Macrophages
The inflammatory and immune response by the damaged CNS includes a prominent mobilization of endogenous microglia and exogenous macrophages to the SCI site, which has been suggested to be a significant source of secondary damage and cell death. However, some experimental studies in animals have shown that autologous activated macrophages promote growth and healing, as opposed to harmful inflammation [42, 54]. Based on preclinical evidence, involving the direct injection of autologous activated macrophages into the spinal cord, a small (n = 8) phase I non-randomized study was initiated in 2000. Marked neurological improvements were reported for the treatment group in terms of AIS grade conversion from A (i.e. sensorimotor complete) to C (i.e. sensory and motor incomplete) [38]. These results stimulated a multicentre phase III RCT 3 years later. Recruiting a total of 43 subjects, autologous activated macrophages failed to show significant improvements in the primary outcome measure (i.e. AIS grade conversion) within the treatment group at 6- and 12-month follow-up [43]. Although disappointing, this study represented a milestone for acute SCI: the first time a cell-based therapy had been administered after SCI [42].
Minocycline
Minocycline is a broad-spectrum antibiotic that has been investigated for its potential to decrease inflammatory reactions following SCI [54, 63]. Although laboratory results have shown conflicting results with regard to its efficacy in animal models, a phase II placebo-controlled clinical trial was recently completed in Canada, administering minocycline within 12 h of injury [10, 54]. This study included 44 individuals with complete and incomplete SCI and confirmed the safety of minocycline use in humans, but did not show any significant neurological improvement compared with the placebo group. Although motor improvement in the treatment arm for incomplete SCI was greater than that of the placebo group, it remains within the range of plausible spontaneous neurological recovery outcomes [40]. The authors suggested that the low numbers in each treatment arm and the heterogeneity of the study groups warranted further research into the efficacy of minocycline after acute SCI [40].
Riluzole
Increased activation of voltage-gated sodium ion channels is thought to play a pivotal role in secondary cell death after SCI through a variety of secondary injury mechanisms (e.g. swelling, acidosis and glutaminergic excitotoxicity) (see chapter 19) [19]. Based on this information, riluzole, a sodium channel blocker, has been proposed as a pharmacological intervention to modulate concentrations of glutamate, thereby protecting the spinal cord from secondary damage [51, 54]. Currently administered for management of amyotrophic lateral sclerosis (ALS), riluzole is in the early stages of clinical trials in SCI [54]. A phase I safety trial administering an initial dose of riluzole (n = 36) within 12 h post-injury reported no increased risk of adverse events. Compared to historical controls, riluzole also had a small beneficial effect on motor outcomes and a tendency for greater AIS grade conversion [31]. A multicentre phase II trial is currently being conducted by the North American Clinical Trials Network (NACTN), as well as a multicentre phase II/III trial known as Riluzole in Spinal Cord Injury Study (RISCIS) [19, 22, 49, 63].
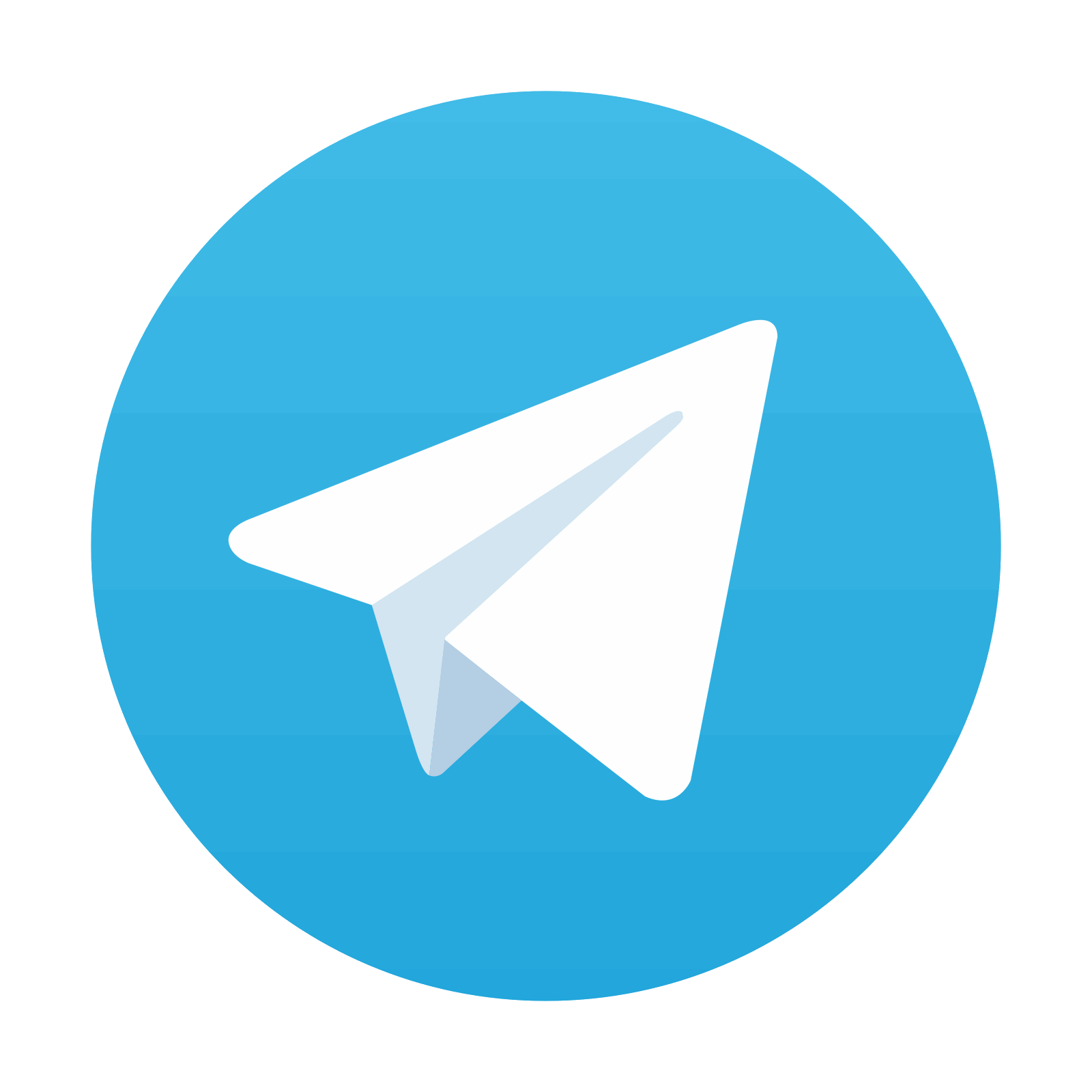
Stay updated, free articles. Join our Telegram channel

Full access? Get Clinical Tree
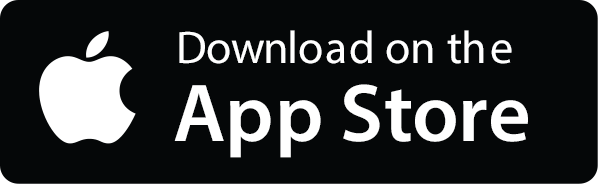
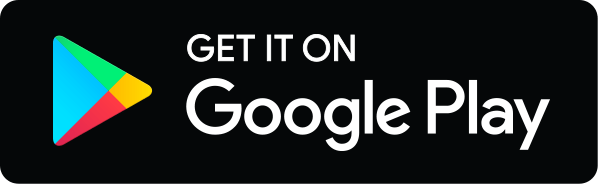
