Abstract
Diseases that afflict the nervous system remain among the most difficult pathologies for clinicians to manage. Currently there are nearly 1000 clinical trials that have been launched worldwide to study motor neuron disease (MND) clinical pathologies, or validate the safety and efficacy of promising MND therapeutics. Even with this high level of scientific investigation and clinical interest, there are no cures or therapies proven to provide long-term benefits. Efforts are continuing to translate potentially beneficial therapies to the clinic. This chapter outlines the current trials for MNDs.
Keywords
Clinical trials, amyotrophic lateral sclerosis, spinal muscular atrophy, gene therapy, stem cell
Outline
Situation and Introduction 278
Therapeutic Technology 280
Viral-Mediated Gene Therapy 280
Stem Cell Therapy 281
Review of Clinical Trial Process 283
Stem Cell Clinical Trials in Amyotrophic Lateral Sclerosis 284
North America 285
United States (Multicenter): Neuralstem 285
Minnesota: Mayo Clinic 291
Louisiana: TCA Cellular 293
Mexico: Servicio Hematologia Hospital Universitario 293
Europe 293
Italy: Azienda 293
Spain: Hospital Universitario Virgen de la Arrixaca 295
Poland: Pomeranian Medical University Szczecin 297
Turkey 297
Asia 298
Israel 298
Iran 300
India: Neurogen Brain and Spine Institute 301
South Korea 302
Spinal Muscular Atrophy: Gene Therapy Clinical Trial 303
Conclusions 304
References
Situation and Introduction
The characteristic symptoms of neurological disorders are caused by the dysfunction or death of cells required for normal function of the central and/or peripheral nervous system. Neural pathways, originating from the highest brain levels, send out complex signals through a series of neuronal synaptic connections arranged through the spinal cord and on through peripheral nerves, and must be maintained in order to preserve normal neurophysiology. For example, amyotrophic lateral sclerosis (ALS) is a devastating degenerative neuromuscular disease believed to occur as a result of cellular dysfunction at the spinal cord level and/or the neuromuscular junction, a specific location where the electrochemically propagated nerve impulse regulates muscle contraction and tone. There is evidence that the motor neurons start to malfunction at a time that precedes detection of motor neuron death. Unfortunately, the sobering reality is that in many neurological diseases there remains a deep chasm that is stalling the development of effective therapies due to a general lack of understanding of the underlying pathological mechanisms driving the disease processes.
The vast majority of preclinical and clinical studies are focused on those disease processes affecting the largest patient populations. The two most funded research areas for neurological disorders are in Alzheimer’s and Parkinson’s, diseases for which there are over 3000 clinical trials listed on ClincalTrials.gov . Alzheimer’s and Parkinson’s diseases are the two most common neurodegenerative diseases and were among the first to be considered for clinical trials involving gene- or cell-based therapies. The advanced development of gene based therapeutics in these disorders compared to others is due to a slightly better understanding of the disease process. For example, researchers have determined that Parkinson’s disease symptoms occur due to dopamine deficiency caused by neuronal cell death within the substantia nigra pars compacta, an area of the brain that regulates body posture and movement by signaling other neurons through synaptic release of dopamine. Therefore, clinical trial strategies have been designed and implemented to elevate or replace dopamine levels to improve functional movement. Some of these strategies have improved the standard of care for many Parkinson’s disease patients, but still no cure has been found. For many of these age-related neurodegenerative diseases, the key to development of effective therapeutics remains in achieving a stronger understanding of the disease process.
The National Institutes of Health defines motor neuron disease (MND) as a group of neurological disorders where the critical cells that control muscle activity are destroyed. These essential cells are the motor neurons that control breathing, swallowing, speaking, and walking. Upper motor neurons are found within the brain and lower motor neurons are found within the brainstem and spinal cord. Currently there exists no cure for MND. While treatment options are available for MND they only provide the patient temporary symptomatic relief or maintain quality of life. The most prevalent MND is ALS. There have been no therapies to date shown to provide long-term benefit for those suffering from this devastating disease. Currently the best option for ALS patients is a drug called riluzole, and it has been shown to increase life expectancy by 2–3 months, albeit on a ventilator. Neurodegenerative diseases such as MND greatly reduce a patient’s quality of life and can be life threatening. Patients who have exhausted all other treatment options are prime candidates for gene- or cell-based therapeutics.
Currently there are nearly 1000 clinical trials that have been launched worldwide to study MND clinical pathologies, or to validate the safety and efficacy of promising MND therapeutics. Even with this high level of scientific investigation and clinical interest there are no cures or therapies proven to provide long-term benefits. While the majority of ALS diagnoses are sporadic, there are many patients who have a genetic link associated with the familial form of ALS. Research into familial ALS has uncovered over 150 mutations in the gene encoding superoxide dismutase 1 (SOD1) that are linked to the disease. These mutations are associated with either a loss of the SOD1 enzymatic activity or a toxic gain of function. These potential causes of ALS lead to increased oxidative stress, protein misfolding and aggregation, mitochondrial dysfunction, and excitotoxicity. Identification of these mutations can be used to study and validate therapeutic targets to treat those patients diagnosed with a specific mutation. While these are exciting possibilities, the fact remains that the majority of ALS patients are diagnosed without a known genetic component.
While the vast majority of MND is diagnosed in patients over the age of 40, an MND called spinal muscular atrophy (SMA) occurs in patients during the early stages of life. Ninety-eight percent of patients with SMA have an alteration of the gene SMN1 through deletion, rearrangement, or mutation. The discovery of alterations within this gene in 1995 spawned many SMA studies focused on the structure of the SMN1 gene. It is believed that the SMN protein protects and sustains the motor neuron population through the maintenance of axonal transport and neuromuscular junctions. Patients presenting with SMA-like symptoms are tested for homozygous deletion for the SMN1 gene, which if confirmed, is 100% specific for developing SMA. Therefore strategies aiming to replace the deleted gene within these motor neurons are believed to very likely provide therapeutic relief. Preclinical studies using SMA models have shown that viral-vector-mediated expression of SMN1 in skeletal muscle and in the brain increases motor function and motor neuron survival. Following up on the preclinical success of this gene therapy strategy, a clinical trial has been launched to treat SMA using modified viral vector expressing the native SMN1 protein (NCT02122952). This viral-mediated gene therapy is intended to halt or delay the disease progression, thus offering a better quality of life for patients suffering from this terrible disease. Diseases with a strong genetic link, such as SMA, are prime candidates for the implementation of gene- or cell-based therapies, which are designed to replenish or correct aberrant gene expression.
Therapeutic Technology
For decades, researchers and clinicians have advanced gene- and cell-based therapies to the cusp of fundamentally altering the way we treat human disease. The ability to transplant multipotent or pluripotent cells or to constitutively express a therapeutic gene in the target diseased tissue provides an attractive alternative to continuous pharmacological intervention. Cellular therapies have been approved by the U.S. Food and Drug Administration (FDA) to treat cosmetic flaws (Laviv®, Fibrocell Technologies Inc.), blood disorders (Ducord, Duke University), cartilage deficiencies (Carticel, Genzyme Biosurgery), dental pathologies (Gintuit, Organogenesis Incorporated), and cancer (Provenge®, Dendreon Corporation). There is widespread research in numerous diseases that utilize viral-vector-mediated therapies. A great many preclinical trials have shown efficacy with viral-mediated gene delivery in animal models of disease. Gene-based therapeutics have been shown to be generally safe for human application, however there were serious complications on the path to acceptability. Specifically, a patient died from a therapeutically induced catastrophic immune response in an early clinical trial. The death of this patient caused a significant delay in the development of gene-based technology as the safety of viral vectors was called into question. Some cellular therapeutic products are already approved for public use but gene therapy products are not yet approved for market release. A particular clinical trial (NCT00643890) using viral-mediated gene therapy in Parkinson’s disease did show efficacy above the placebo range but the study was terminated because it did not elevate the current standard of care. Modern generations of viral vectors have been demonstrated to be safe in multiple trials but their efficacy in clinical trials rarely surpass either the placebo-effect range or the current standard of care.
Viral-Mediated Gene Therapy
Gene therapy uses viral or synthetic vectors to deliver exogenous genetic material to living cells and tissues to elicit therapeutic benefit. Preclinical studies commonly use modified viral vectors derived from naturally occurring viruses that infect human cells. Modified viruses include retroviruses, such as lenti and HIV; Herpes simplex virus 1 presents as cold sores; adenovirus presents as respiratory sickness; or adeno-associated viruses, which have no known pathogenicity in humans. Researchers use the naturally evolved mechanisms of infectious viruses to transduce diseased cells and express a potentially therapeutic gene. For example, the adeno-associated virus serotype 9 (AAV9) is particularly useful for peripheral administration (i.e., intravenous) because it can cross the blood–brain barrier to express exogenous gene products within the central nervous system (CNS). While some particular genetic characteristics of these viral vectors make them attractive for therapeutic engineering, other pathogenic viral genes must be removed to make them safe, and therefore useful for therapeutic development.
Stem Cell Therapy
Stem cells are extremely promising therapeutics that have the potential to support or replace cells vulnerable to dysfunction and/or death in a wide range of human diseases. The term “stem cell” was first used by German scientist Ernst Haeckel to describe the fertilized egg that will give rise to a complicated organism. Stem cells demonstrate these three general characteristics: (1) they can divide and multiply for prolonged periods; (2) they are unspecialized cells; and (3) they can differentiate into specific cell types depending on stimuli ( http://stemcells.nih.gov.easyaccess1.lib.cuhk.edu.hk/info/basics/pages/basics2.aspx ). There are different types of stem cells depending on how they were isolated and from what tissue they were taken. Stem cells after transplantation have been shown to align with and become tissue-specific cells given specific physiological or experimental stimuli. Other stem cell strategies involve transplanting cells that will express growth factors or neurotrophic factors to support viability or function in vulnerable cell populations. Although to date there have been many stem cell-based clinical trials launched to treat neurological diseases, the field remains in the early stages of development and application. Many preclinical studies have shown great efficacy in a large number of wide-ranging disease models, but successful implementation into human therapies have been incremental.
The advancement of stem cell therapy has been relatively slow because early stem cell lines were embryo-derived, a stem cell source that has remained a national ethical dilemma from its inception. Many nations have government-imposed regulations on the development and use of stem cells in biomedical research. The controversial issues surrounding stem cell therapeutics span ethical, moral, and political debates.
The two main types of stem cells are human embryonic derived stem cells (hESCs) and somatic (adult) stem cells (ASCs) that are found in and isolated from specific body tissues. A particular advantage of hESCs is their ability to differentiate to become any cell found within the human body, which is termed “pluripotency.” Pluripotent cells are amenable to a broad range of therapeutic applications given their ability to divide for long periods of time in addition to their vast differentiation potential. Conversely, isolated ASCs do not readily produce large quantities of stem cells and may only be able to differentiate into the limited cell types found in the tissue of origin. Within the bone marrow, a common tissue where stem cells are isolated from, there are two types of stem cells: hematopoietic (HSCs; give rise to all blood cells) and mesenchymal stem cells (MSCs; give rise to bone, cartilage, and fat cells). In the 1990s it was discovered that neural stem cells (NSCs) inhabited the adult brain and could give rise to the three major brain cell types: astrocytes, oligodendrocytes, and neurons. This discovery was of particular interest to those researching neurological disease in that it could allow for isolation of neural precursors to develop novel strategies for advanced treatments.
The deleterious effect of the host’s immune system on stem cell survival after transplantation is of particular concern. Patients that receive allogeneic stem cells (usually hESCs) are likely to need continuous administration of immunosuppressive drugs, which can leave the patient vulnerable to infection. Stem cell transplantations using “autologous” stem cells are believed to reduce the risk of host immune-response mediated rejection as the patient is receiving their own cells and not cells from a foreign donor. While a patient is less likely to have a robust immune response after autologous cell transplantation, when compared to donor cell transplantation, the risk remains that newly transplanted autologous cells within diseased tissue will not function as they did within the tissue of origin. There is also potential that the transplanted cells isolated from a diseased patient could be in a diseased state as well. Since many millions of cells are needed for cell-mediated therapy, ESCs are more amenable to growing large numbers of therapeutic cells for the treatment of disease. Therefore, more research is warranted on the specific conditions that are required to achieve successful transplantation and subsequent engraftment.
Another type of stem cell, termed the induced pluripotent stem cell (iPSC) has recently been elevated as the new improved stem cell therapy that has characteristics of both hESCs and MSCs. iPSCs are pluripotent because they have been reprogrammed to replicate gene expression observed in ESCs. Human iPSCs express embryonic stem cell markers and are capable of generating cells characteristic of all three germ layers. This technology enables the transplantation of reprogrammed autologous cells that maintain somatic genetic homogeneity with the patient (as with autologous MSCs) but with the potential to differentiate into any cell found in the body (as with hESCs). While these cells have been met with great excitement in the therapeutic arena, a concerning issue with advancing the clinical use of iPSCs is their propensity to form tumors. As with any new medical technology, a patient’s safety must be paramount.
In recent years the FDA has approved many trials that aim to use stem cell therapy to treat many devastating diseases. These trials include evaluation of stem cell therapies in diseases such as neurodegenerative disorders, cardiovascular disorders, genetic disorders, cancer, musculoskeletal disorders, and degenerative eye conditions. Moreover, HSCs have been included in blood transfusions for almost half a century, and transplantations of isolated and expanded HSCs have been conducted ( http://stemcells.nih.gov.easyaccess1.lib.cuhk.edu.hk/info/scireport/pages/chapter5.aspx ).
Review of Clinical Trial Process
Clinical trials are used to determine whether or not novel therapies that exhibit therapeutic benefit in preclinical studies can be “translated” into effective treatments for human disease. In the United States, the FDA has regulated the clinical trial process since the 1970s. Prior to the FDA approving the commencement of human clinical trials, they first require that these clinical trials must be designed to comply with established good clinical practices (GCPs) to ensure the safety of human trial subjects. There are now international guidelines established in concert with the FDA to standardize the GCPs used to conduct clinical trials throughout the world. Therapeutics should only advance to early-stage clinical trials if there is sufficient preclinical data supporting the probable safe usage in human subjects. Therapies validated by the clinical trial process can be chemical, biological, thermal electrical, or behavioral. Depending on the nature of the therapy to be tested, there is a department within the FDA responsible for approval and oversight of the trial. Studies in gene and cellular therapies are specifically regulated by the FDA’s Center for Biologics Evaluation and Research (CBER). In addition to establishing safe effective parameters for the development of therapeutic biologics, the CBER also educates the public on the safe and appropriate usage of biological products.
The clinical trial process ( http://www.fda.gov/ForPatients/Approvals/Drugs/ucm405622.htm ) utilizes a series of trials to scientifically establish parameters for use of an investigational therapeutic pertaining to safety, dosage, efficacy, and side effects. In phase I trials, a team of investigators will test a new therapeutic in a relatively limited group of people to establish safety data. In addition to safety, the dosing range and potential efficacy may be monitored prior to launching the phase II trial. Phase I trials are usually conducted in healthy subjects to ensure that the therapeutic itself causes no adverse effects in the absence of a disease state. However, in cell- and gene-based therapeutics, phase I trials usually evaluate safety as a primary measure, and efficacy as a secondary measure in diseased patients. This diversion from enrolling healthy participants in phase I clinical trials is due to the potential for long-term or permanent adverse effects associated with gene- or cell-based therapies when compared to transient small-molecule drug therapies. Phase I trials are usually open-label, which means that the patient is aware of the potential therapeutic outcome. This means that there is a likely placebo effect that must be taken into consideration when looking to move therapeutics into the next trial phase.
The phase II trial remains committed to monitoring safety of treatment in a larger cohort while determining whether the therapeutic represents an effective treatment for the relevant disease. If possible, these larger cohorts will be conducted under “closed-label” and “blinded” conditions, meaning that that trial subjects are not told what therapeutic they are given or even whether or not they have been given the therapeutic or the inert placebo. This gives the trial more validity if there is evidence of efficacy in the treated group versus the control because neither group knows what they had received. Phase I and II clinical trials involving terminally ill patients or complex invasive procedures may not include a true “control or placebo” group. While the ideal trial involves blinding the researchers and the patients to the treatments it is unlikely that there will be true control groups in late-stage neurodegenerative disease studies, unless they advance to phase III, due to the invasive nature of intraparenchymal or intrathecal CNS delivery. In cases such as these, efficacy is usually established by a comparison with established disease progression rates.
If the therapeutic shows efficacy in a phase II trial then it will be moved into the phase III trial to be administered in multiple-center trials to confirm efficacy, generate more safety and dosage data, and compare it with currently available treatments. Phase III trials have large control and therapeutic cohorts (usually minimum of 100 participants) where randomized patients and investigational clinicians are blinded to who gets what. Finally, if the novel therapeutic drug or biologic advances successfully through the first three clinical trial phases, and it exceeds the current standard of care, it will be brought to market for distribution and clinical use. During this open-market period, ongoing phase IV studies continue to evaluate the therapeutic efficacy in diverse populations and determine long-term effects of use. These studies are of particular use to determine future applications for new therapeutics.
Stem Cell Clinical Trials in Amyotrophic Lateral Sclerosis
There are close to 5000 clinical trials related to stem cell therapies listed on ClincalTrials.gov , a US government website that lists proposed, ongoing, or completed clinical trials. Numerous clinical trials using stem cell therapy to treat ALS have been launched worldwide. These ALS clinical trials have shown the use of stem cell therapies to be safe for delivery to affected muscle regions, cerebrospinal fluid (CSF), and direct CNS parenchyma. As many of the following studies have yet to release their trial data, much of the information provided is taken from ClincalTrials.gov . However, the FDA cautions that while Clinicaltrials.gov makes available information provided by the trial sponsors, this information is neither reviewed nor confirmed by the FDA. These stem cell trials are organized by geographical location, and trials that are conducted by the same group of investigators are listed with one another ( Table 12.1 ).
Sponsor/trial location | Nomenclature (estimated start) | Trial phase: outcome measures | Stem cell type | Delivery technique | Dosages tested | Published results (PMID) |
---|---|---|---|---|---|---|
NORTH AMERICA | ||||||
Neuralstem/Emory University (Atlanta, GA) | NCT01348451 (2009) | Phase I | Human spinal cord-derived (fetal) | Direct injections into the cervical and/or lumbar region of spinal cord | 500,000 to 1.5×10 6 cells total | Riley et al. (22565043) |
Glass et al. (22415942) | ||||||
Feldman et al. (24510776) | ||||||
Boulis et al. (22158518) | ||||||
Tadesse et al. (25540804) | ||||||
Neuralstem/Emory University (Atlanta, GA) | NCT01730716 (2013) | Phase II | 500,000 to 1.5×10 6 cells total | N/A | ||
Mass general (Boston, MA) | ||||||
U. of Michigan (Ann Arbor, MI) | ||||||
Mayo clinic/(Rochester, MN) | NCT01142856 (2010) | Phase I | Autologous adipose- derived mesenchymal (adult) | Intrathecal infusion | 0.6×10 6 cells/kg | http://www.cellr4.org/article/530 |
Mayo clinic/(Rochester, MN) | NCT01609283 (2012) | Phase I | 1×10 7 to 2×10 8 cells total | N/A | ||
TCA cellular therapy/Covington, LA | NCT01082653 (2010) | Phase I | Autologous bone-marrow-derived mesenchymal (adult) | Intrathecal infusion | N/A | N/A |
Q Therapeutics, Inc./(Salt Lake City, UT) | NCT02478450 (2015) | Phase I/II | Glial restricted progenitor cells | Direct injections into the cervical or lumbar region of spinal cord | N/A | N/A |
Brainstorm-cell therapeutics/(Mass General, UMass, Mayo Clinic) | NCT02017912 (2013) | Phase II | Autologous bone marrow-derived mesenchymal that express neurotrophic factors | Intrathecal infusion and intramuscular injection | N/A | N/A |
Hospital Universitario/(Monterrey, Mexico) | NCT01933321 (2012) | Phase I | Autologous hematopoietic | Intrathecal infusion | N/A | N/A |
EUROPE | ||||||
Azienda Ospedaliera/(Santa Maria, Italy) | NCT01254539 (2001) | Phase I/II | Autologous bone-marrow- derived mesenchymal | Intrathecal infusion | 8×10 7 to 1.5×10 8 cells total | Mazzini et al. (19682989) |
Mazzini et al . (21954839) | ||||||
NCT01640067 (2011) | Phase I | Human neural (fetal) | Unilateral or bilateral direct spinal cord injection | 2.25×10 6 to 5.5×10 6 cells total | Mazzini et al . (25889343) | |
Foundation for health research and training/(Murcia, Spain) | NCT00855400 (2009) | Phase I/II | Autologous bone-marrow-derived mesenchymal (described as mononuclear) | Direct injections into the thoracic region of spinal cord | 1.38×10 8 to 6.03×10 8 cells total | Blanquer et al . (22415951) |
Andalusian initiative for advanced therapies/(Multi-Locations, Spain) | NCT02290886 (2014) | Phase I/II | Adipose-derived mesenchymal | Intravenous injection | 1×10 6 to 4×10 6 cells total | N/A |
Red de Terapia Celular/(Murcia, Spain) | NCT02286011 (2014) | Phase I | Autologous bone-marrow-derived mesenchymal (described as mononuclear) | Intramuscular infusion | 1×10 8 to 1.2×10 9 cells total | N/A |
Pomeranian medical uni./(Szczecin, Poland) | NCT02193893 | Phase I | Autologous bone-marrow-derived mesenchymal | Intrathecal infusion | N/A | N/A |
ASIA | ||||||
Haddassah medical Org./(Jerusalem, Israel) | NCT01051882 (2010) | Phase I/II | Autologous bone-marrow-derived mesenchymal that express neurotrophic factors | Intrathecal infusion and intramuscular injection | 2.4×10 7 to 6×10 7 cells total | Karussis et al. (20937945) |
NCT01777646 (2012) | Phase II | 9.4×10 7 to 1.4×10 8 cells total | N/A | |||
Neurogen Brain and Spine Institute/(Mumbai, India) | NCT02242071 (2008) | Phase I | Autologous bone-marrow-derived mesenchymal | Intrathecal infusion and intramuscular injection | <8×10 7 cells total | Sharma et al. (25973331) |
NCT01984814 (2008) | Phase II | N/A | N/A | |||
Royan institute/(Tehran, Iran) | NCT01759784 (2012) | Phase I | Autologous bone-marrow-derived mesenchymal | Intraventricular injection | N/A | N/A |
NCT01759797 (2012) | Phase I | Intravenous injection | N/A | N/A | ||
NCT01771640 (2013) | Phase I | Intrathecal infusion | N/A | N/A | ||
NCT02492516 (2014) | Phase I | Autologous adipose-derived mesenchymal | Intravenous injection | N/A | N/A | |
Alzahra hospital/(Isfahan, Iran) | NCT02116634 (2014) | Phase I/II | Autologous bone-marrow-derived mesenchymal | Intrathecal infusion | 1×10 8 cells total | N/A |
Corestem Hanyang University/(Seoul, Republic of Korea) | NCT01363401 (2011) | Phase I/II | Autologous bone-marrow-derived mesenchymal | Intrathecal injection | 1×10 6 cells/kg | Oh et al. (25934946) |
NCT01758510 (2012) | Phase I | Allogenic bone-derived mesenchymal | Intrathecal infusion | 2.5×10 5 to 1×10 6 cells/kg | N/A |
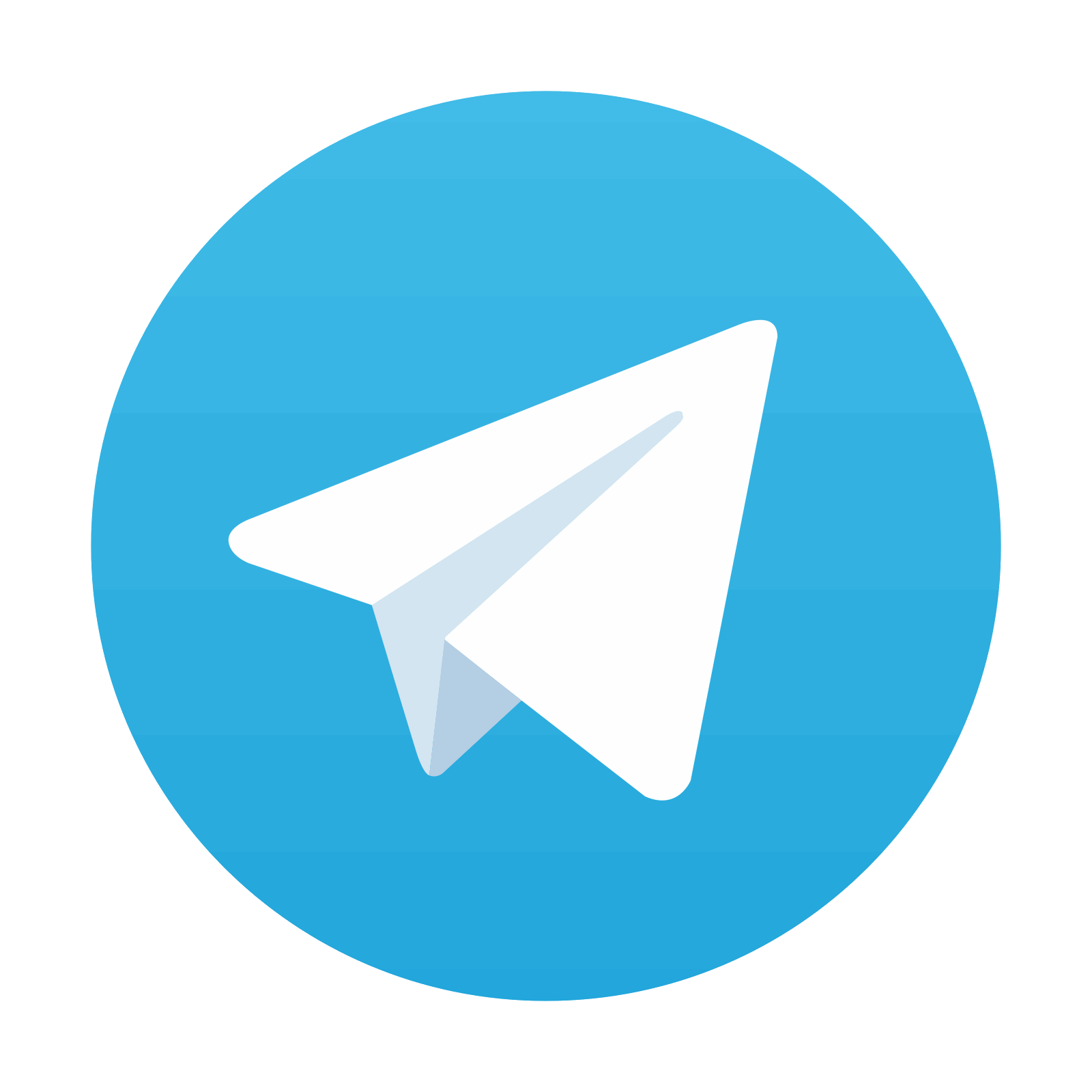
Stay updated, free articles. Join our Telegram channel

Full access? Get Clinical Tree
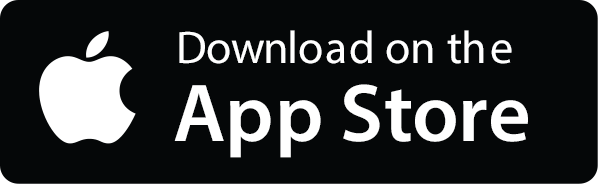
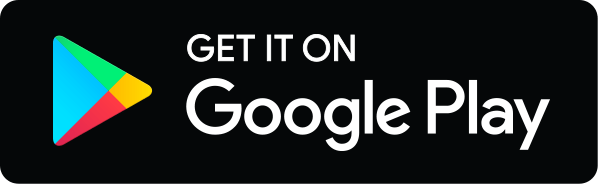
