© Springer International Publishing Switzerland 2017
James M. Ecklund and Leon E. Moores (eds.)Neurotrauma Management for the Severely Injured Polytrauma Patient10.1007/978-3-319-40208-6_2121. Coagulopathy in Traumatic Brain Injury
(1)
Walter Reed National Military Medical Center, Bethesda, MD, USA
Keywords
Isolated traumatic brain injuryInternational mission for prognosis and analysis of clinical trials in TBI (TBI-IMPACT)HemostasisVon willebrand factor (vWF)Advanced bleeding care in trauma (ABC-T)CoagulopathyFibrinogenesisFibrinolysisThrombocytopeniaProcoagulantsDesmopressinCryoprecipitateFresh frozen plasmaTranexemic acidAminocaproic acidProthrombinWarfarinHeparin apixabanDabigatranBilvalirudinAspirinClopidogrelAbciximabFibrinogenActivated factor VIIHemorrhagic and is chemical complicationsPlatelet dysfunctionTraumatic coagulopathyCoagulopathy in Traumatic Brain Injury
The coagulopathy commonly seen with head injury is associated with worse clinical outcomes than trauma without head injury. Head injury is associated with delayed or progressive bleeding, ischemic secondary injury, and microvascular thrombosis [1]. The general prevalence of trauma-associated coagulopathy in trauma can be as high as 97.2 % [2]. Low Glasgow Coma Score, high Injury Severity Score, hypotension on admission, the presence of cerebral edema, subarachnoid hemorrhage and midline shift are independent risk factors for developing coagulopathy in patients with isolated traumatic brain injury [1]. The International Mission for Prognosis and Analysis of Clinical Trials in Traumatic brain injury (IMPACT) study in 2007 showed that an abnormal PT on admission was an independent risk factor for bad outcome after traumatic brain injury [3]. While multiple studies have demonstrated that abnormities of international normalized ratio, (INR) PTT, platelet count, or fibrinogen degradation products are associated with worse outcomes [4]. A coagulopathy after head injury that develops within 24 h is associated with 55 % mortality while the mortality was 23 % for those who developed coagulopathy after 24 h [5]. The pathophysiology of this coagulopathy is a work in progress. Blood loss and hemodilution secondary to fluid resuscitation is not the most likely mechanism for coagulopathy associated with head injury [1]. Direct injury with activation of coagulation by tissue factor, insufficient control of fibrinogenesis and fibrinolysis play a role in the acute coagulopathy of trauma seen in these patients. A basic understanding of the coagulation cascade is essential.
Coagulation begins almost instantly after injury of a blood vessel [6]. Primary hemostasis , the activation of platelets and secondary hemostasis, the activation of the coagulation cascade both occur upon injury. Exposure of blood to the subendothelial space results in the presentation of subendothelial tissue factor to both platelets and plasma factors. The platelets bind to collagen directly with collagen-specific glycoprotein Ia/IIa receptors. With the help of von Willebrand factor, (vWF) produced by endothelium and platelets, collagen binds to the glycoprotein Ib/IX/V on the platelets. Activated platelets release ADP, thromboxane A2, vWF, platelet activating factor, and platelet factor 4. The activated platelets change their shape from spherical to stellate and have an increased affinity of their glycoprotein IIb/IIIa to assist the platelet to bind to fibrin. This change in shape and increased platelet binding to fibrin are important in clot formation.
Factor VII leaves the circulation through the damaged blood vessel and comes in contact with tissue factor. Factor VII is converted to VIIa. VIIa converts factor X to Xa. Xa combines with factor V to form a prothrombinase complex which activates prothrombin to form thrombin. Thrombin has many effects on both factors and cells. Thrombin activates and releases Factor VIII. Thrombin converts factor XI to XIa. Thrombin converts factor V to Va and fibrinogen to fibrin. Thrombin also activates factor XIII needed for crosslinking fibrin to form covalent bonds in the final clot. High molecular weight kininogen (HMWK), prekallikrein, and Factor XII are all activated by exposure to collagen. Factor XIIa activates factor XI with in turn activates IX. IXa combines with factor VIIIa which forms a Tenase complex that along with Va produces even more thrombin. Activation of HMWK, Prekallikrein, and factor XII are probably more important in inflammation.
Calcium and Vitamin K are important cofactors for coagulation. Calcium is needed for many parts of the coagulation cascade. Vitamin K is needed for factors II, VII, IX, X, Protein S, and Protein C.
The anticoagulant pathways down regulate the coagulation pathway. Thrombin has an important role in modulation of coagulation. Thrombin also combines with thrombomodulin an endothelial cofactor important in inflammation. Thrombin-thrombomodulin acts as a cofactor for protein C activation. Activated protein C along with protein S degrade factor Va and VIIIa. Antithrombin made by the liver, targets factors Xa, IXa, XIa, XIIa, and factor IIa. (Heparin activates antithrombin) Tissue factor inhibitor (TFI) inhibits Xa. TFI-Xa complex binds to the VIIa tissue factor complex further inhibiting Xa production. Plasmin is created by the effect of tissue plasminogen activator released by the endothelium on plasminogen released by the liver. Plasmin proteolytically cleaves fibrin and controls fibrin deposition. The destruction of clot primarily by plasmin with the assistance of other anticoagulant pathways is termed as fibrinolysis .
How drugs effect the coagulation system is enlightening. Procoagulants work on either platelets or the coagulation cascade. Desmopressin promotes the release of von Willebrand factor which can improve platelet function as well as increase factor VIII levels [7]. Cryoprecipitate has fibrinogen , factor VIII, von Willebrand factor, and factor XIII [8]. Fresh frozen plasma contains an unconcentrated source of all clotting factors. Recombinant activated factor VII can increase thrombin production by activating factor X directly [9]. Tranexemic acid and aminocaproic acid inhibit the activation of plasminogen to plasmin [10]. Prothrombin complex concentrate has factors II, VII, IX and X, protein S, and protein C. (Prothrombin complex concentrate contains heparin ) [11]. Warfarin affects the production of vitamin k-dependent clotting factors, Heparin , both fractionated and nonfractionated, increases the activity of antithrombin on factors Xa by as much as a 1000 fold. Apixaban is a direct Xa inhibitor. Dabigatran and Bilvalirudin are univalent and bivalent direct thrombin inhibitors [12]. Aspirin inhibits the production of thromboxane from platelets. Clopidogrel blocks the P2Y12 ADP receptor on platelets. Abciximab inhibits GpIIb/IIIa receptors [13].
Laboratory testing for the extrinsic tissue factor pathway is the prothrombin time (PT). The PT is sensitive to dysfunction of factors VII, V, X, and II while the partial thromboplastin time (PTT) is prolonged with abnormal function of factors I, II, V, VIII, IX, X, XI, and XII. Thrombin levels are difficult to measure directly but can be estimated my measuring thrombin antithrombin complex and prothrombin fragments. Thrombin time (TT) is measured by adding bovine thrombin to plasma and seeing how long it takes to clot. Decreased fibrinogen levels or the presence of an anticoagulant can increase TT. Fibrinogen levels can be measured directly. Platelet function can be measured by analyzers such as the rapid platelet function assey. (Verify Now) [14]. The detection of fibrin degradation products suggests ongoing fibrinolytic activity. D-dimers are most commonly measured. Tissue type plasminogen activator inhibitor and plasminogen activator inhibitor are also abnormal during fibrinolysis [15].
Thromboelastography (TEG) has the ability to measure the kinetics of clot formation and stability. It can measure both hypercoagulable and hypocoagulable states. Thromboelastograms typically describe an R value, an alpha angle, a maximum amplitude (MA) and the percentage of clot lysed at 30 min LY30. The R value is a reflection of coagulation factor activity, the alpha angle represents the thrombin burst and fibrin production, the MA measures both platelet function and fibrin level (80/20 %) and the LY30 describes fibrinolysis. Studies have shown goal-directed therapy of coagulation abnormalities diagnosed with TEG might lower the morbidity and mortality associated with trauma [16–18].
The coagulopathy seen after traumatic brain injury is multifactorial. This coagulopathy can occur without significant dilution of clotting factors. Etiologies for coagulopathy after head injury include: Release of tissue factor, production of microparticles, fibrinolysis , platelet function abnormalities, abnormalities of protein C, elevated thrombomodulin, activation of inflammatory mediators, and reduction of factor V levels may all may play a role in the acute coagulopathy seen with traumatic head injury [19, 19].
Tissue factor is released into the systemic circulation which activates factor VII and causes a consumptive coagulopathy. Tissue factor release has been reported in generalized trauma, traumatic head injury, and sepsis in some brain tumor patients [20, 21]. Head injury has also been associated with circulating microparticles released from either apoptotic or activated cells. These micro particles can be of platelet or endothelial origin [22]. Traumatic brain injury is associated with the formation of microthrombi in the small arterioles and venules. A local or systemic coagulopathy triggered by injured brain can contribute to the production of these microthrombi [23]. In animal studies, an immediate postcontusional blood flow reduction is associated with platelet thrombi in the microcirculation [24]. By blocking the microcirculation, the microthrombi can cause both transient and permanent injury to brain tissue. Head injury is associated with elevation of tissue plasminogen activator, increased factor C and depletion of plasmin inhibitor. Increased levels of these factors can result in increased fibrinolysis [25]. Fibrinolysis as evidenced by elevated levels of fibrinogen degradation products is associated with worse outcome in patients with traumatic brain injury [26]. Thrombocytopenia after head injury is associated with increased mortality [27]. Platelets do not function as well after traumatic head injury. An unknown platelet inhibitor or exhaustion of intracellular platelet mediators could be mechanisms of platelet dysfunction [28, 29]. Elevated thrombomodulin is seen in head injury and can be associated with increased activated protein C [4]. Inflammatory mediators such as cytokines or compliment also contribute to coagulopathy. Increases in levels of both coagulation factors and inflammatory mediators can be obtained in the CSF of head injury patients suggesting that there is and arteriovenous difference in these factors in patients with traumatic head injury [30]. Acidosis as a consequence of massive hemorrhage changes the activity of factor VII and to a lesser extent factors V and X [31]. Hypoperfusion in trauma is associated with a marked reduction in factor V activity with a small reduction in II, VII, IX, X, and XI. The marked drop in factor V may represent fibrinolytic reaction because factor V is very susceptible to breakdown by fibrinolysis [32].
Postraumatic abnormalities of the coagulation cascade can result in both hemorrhagic and ischemic complications . Coagulopathy has been associated with the development of new or progression of existing lesions in TBI patients. Hemorrhagic progression or new development of ischemic lesions was found in 85 % of those with coagulopathy on admission while on 31 % of those without coagulopathy had progression of symptoms [33]. Hemorrhagic contusions were the lesions most likely to expand on intracranial CT especially within the first two hours of the trauma. A significant increase in mortality is noted in coagulopathic patients with hemorrhagic progression on follow up CT. The greatest risk factor for progression of hemorrhagic lesion was coagulopathy within the first 24 h after traumatic brain injury. In one study, patients with an elevated PTT demonstrated a 100 % hemorrhagic progression rate after traumatic brain injury. Thrombocytopenia with a platelet count less than 100,000 was associated with a 90 % progression rate while increased PT was associated with a 70 % progression rate [34]. A review of 113 TBI patients with 229 non-operated intraparenchymal hemorrhages did not show coagulopathy to be a significant risk factor for hemorrhagic progression [35]. Documented mortality rate in trauma patients with fulminant fibrinolysis was 85.7 % compared with 11.1 % those with low-grade fibrinolysis. Patients with fibrinolysis had higher ISS, lower GCS, lower SBP, and higher lactate levels than patients without fibrinolysis [36].
Management of bleeding and coagulopathy following traumatic brain injury is paramount to a successful outcome. Early monitoring of coagulation, early administration of FFP and platelets, maintaining adequate calcium and fibrinogen levels, treatment with desmopressin , prothrombin complex concentrate, activated factor VII, and tranexamic acid may play a role in the treatment of traumatic brain injury.
Early monitoring of coagulation is important to detect trauma-associated coagulopathy. Monitoring can assist in the proper diagnosis of the coagulopathy including possible fibrinolysis . Early therapeutic intervention of coagulopathy based on early goal directed therapeutic treatments has been shown to reduce the need for packed red blood cells, fresh frozen plasma , and platelets [37].
The multidisciplinary Task Force for Advanced Bleeding Care in Trauma guidelines was updated in 2013 [38]. The task force recommend fresh frozen plasma or fibrinogen should be administered very early in the management of trauma patients with massive bleeding. Further plasma should be transfused at a ratio of at least 1:2 with red blood cells. Initial plasma transfusion should be avoided in patients without substantial bleeding [37]. Fresh frozen plasma (FFP) has about 70 % of the normal level of all clotting factors. Decreased mortality has been demonstrated in patients who were able to meet a 1:1 ration of FFP/PRBCs [39]. Use of fresh frozen plasma is associated with increased incidence of post injury multiple organ failure, acute respiratory distress syndrome, infections, and transfusion-related lung injury [40, 41]. Therefore, administration of FFP is most appropriate for patients with massive bleeding or bleeding complicated by coagulopathy . Fibrinogen concentrate or cryoprecipitate should be used to keep the plasma fibrinogen level above 1.5 g/dl [42].
After trauma, it has been found that the platelet dysfunction is noted even before substantial fluid or blood transfusion takes place and continues during the resuscitation period. Severe injury can result in increased platelet activation and abnormal function in traumatic brain injury [38]. This suggests that early platelet transfusion may be helpful [43]. There is evidence to support an appropriate platelet transfusion threshold in the trauma patient both due to thrombocytopenia or pre-trauma ingestion of antiplatelet drugs. In massively transfused patients, a platelet count of 100,000/ml has been found as the threshold for diffuse bleeding [44]. A platelet count 50,000 or fibrinogen level of <0.5 g/l have been found as sensitive laboratory predictors of microvascular bleeding [45]. A platelet count of <100,000/ml was an independent predictor of mortality in patients with traumatic brain injury [46]. A target level of 100,000/ml can be suggested for those with multiple trauma, brain injury and massive bleeding [47, 48]. For the management of traumatic coagulopathy , there is still no high-quality evidence supporting up-front platelet transfusions. A prospective randomized trial evaluating prophylactic platelet transfusion at a ratio to the whole blood of 1:2 versus the same amount of plasma in patients receiving ≥12 units of whole blood in 12 h concluded that platelet administration did not affect microvascular nonsurgical bleeding [49]. A metaanalysis of 16 retrospective studies published between 2005 and 2010 that investigated the impact of platelet transfusion in severe trauma and massive transfusion showed an improved survival rate among patients receiving high platelet:RBC ratios [50–52]. High platelet:RBC ratios and high plasma:RBC ratios are often administered concurrently and it is therefore hard to isolate the benefit of one with the other. A potential shortcoming of ratio-driven blood support is over transfusion with plasma and platelets, resulting in possible increased morbidity from multiple organ failure that can be seen with the transfusion of blood products [40]. Although there is a demonstrated decrease in morbidity due to aggressive use of plasma and platelets, routine early prophylactic platelet transfusion as part of a massive transfusion protocol is probably not justified [37, 53–55].
Platelets should be administered to head injury patients with intracranial hemorrhage who have been treated with antiplatelet agents. Antiplatelet agents, mainly aspirin and clopidogrel, can have an affect on traumatic bleeding. Head trauma (GCS 14 to 15) while on antiplatelet agents is associated with a high incidence of intracranial hemorrhage [56–58]. Patients who used clopidogrel prior to both spontaneous and traumatic intracranial hemorrhage are associated with worsened outcome [59–62]. Compared to controls, patients on clopidogrel demonstrated a 14.7-fold increase in mortality, increased morbidity and a 3-fold increase in disposition to a long-term facility [60, 61]. Pre-injury aspirin did not affect outcomes in mild to moderate head injury or morality. However, greater platelet inhibition was identified among patients taking a combination of antiplatelet agents compared to those on single agents [63–66]. Early platelet dysfunction was found after severe traumatic brain injury in the absence of antiplatelet drugs [67]. These findings coupled with the fact that 20–30 % of patients are nonresponders to aspirin, clopidogrel or both agents suggest that reliable measures of platelet function would be useful in the setting of the bleeding trauma patient to guide therapy [38, 68].
Besides platelet transfusion, other potential antiplatelet reversal therapies can be used [69]. Desmopressin has been shown to improve platelet function in volunteers on aspirin , clopidogrel and perioperatively in patients with mild inherited platelet defects [70–72]. One metaanalysis suggested a benefit of desmopressin in patients taking aspirin [73]. Desmopressin has been recommended in patients taking platelet inhibitors with intracranial hemorrhage [69, 74]. Activated factor VII reverses the inhibitory effects of aspirin and clopidogrel in healthy volunteers [75]. Tranexemic acid and fibrinogen concentrate may also be helpful in improving hemostasis in trauma patients receiving antiplatelet agents [76, 77].
Head injury patients are increasingly on new oral anticoagulants. These agents are indicated for the prevention of venous thromboembolism, prevention of stroke in atrial fibrillation, reduction of cardiovascular events in patients with acute coronary syndrome, treatment of pulmonary embolism, and treatment of deep venous thrombosis. The primary modes of action by these novel drugs are direct factor Xa inhibition (rivaroxaban, apixaban , and endoxaban) or thrombin inhibition (dabigatran) [78–80]. It has been demonstrated that the effect of these drugs on coagulation tests of factor Xa (rivaroxaban) but not of factor IIa (dabigatran ) antagonists in human volunteers could be reversed with high-dose prothrombin complex concentrate (PCC) [81, 82]. Anti-factor Xa activity can be measured with a substrate-specific anti-factor Xa test in trauma patients treated with factor Xa antagonists. Factor IIa antagonist treatment does prolong PTT and thrombin time but does not respond to prothrombin complex concentrate [82, 83]. Prothrombin complex concentrate can also be used for the emergency reversal of vitamin K-dependent oral anticoagulants in patients with head injury. The use of PCC carries the increased risks of both venous and arterial thrombosis during the recovery period; therefore, the risk of a thrombotic complication due to treatment with PCCs should be weighed against the need for rapid and effective correction of coagulopathy [84–86]. Thromboprophylaxis, as early as possible after control of bleeding, has been achieved as recommended in patients who have received PCC. Idarucizumab, a monoclonal antibody fragment that binds dabigatran, was approved by the FDA on October 16, 2015. For patients treated with dabigatran when reversal of the anticoagulant effects of dabigatran is needed for emergency surgery or urgent procedures or in life-threatening or uncontrolled bleeding [38, 87, 88].
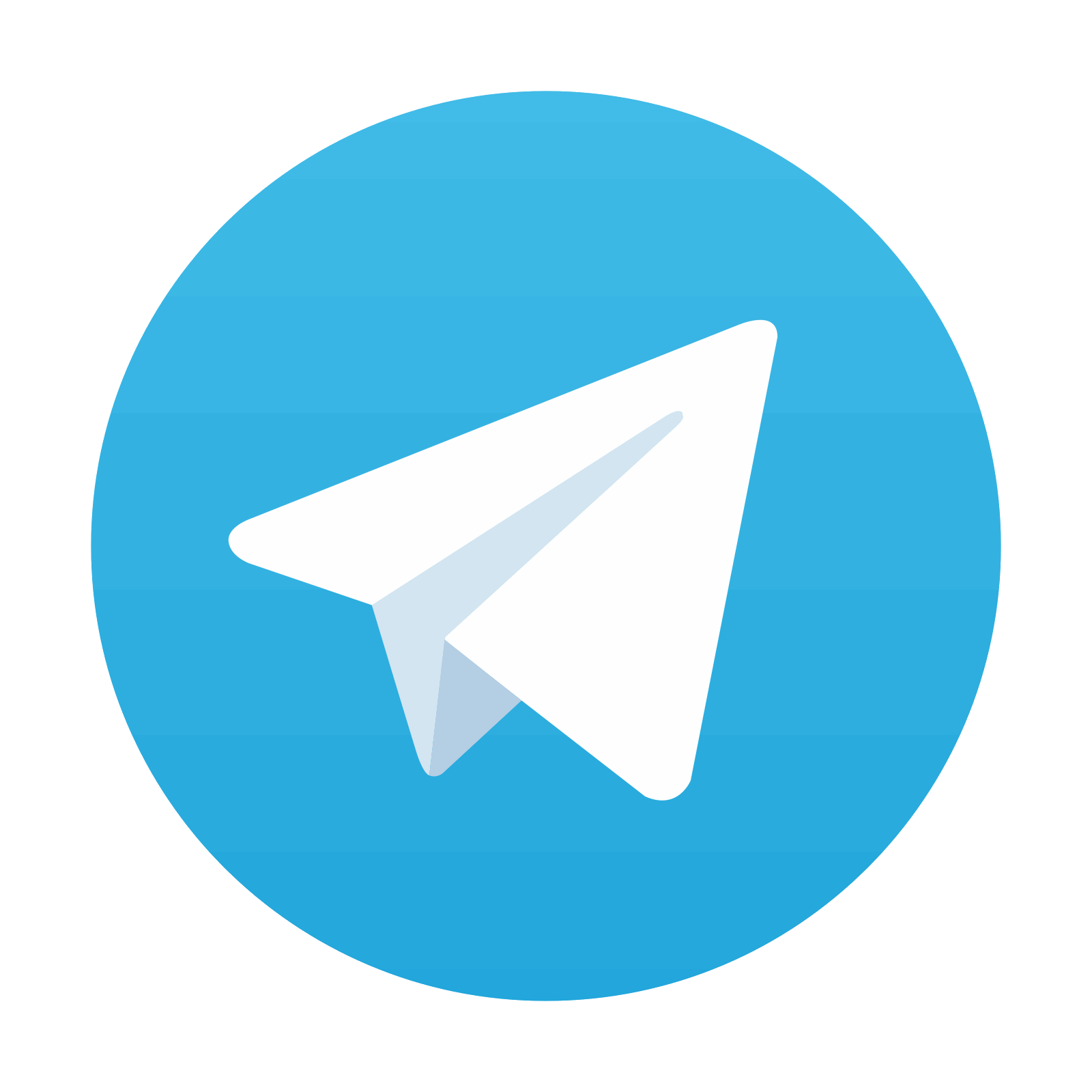
Stay updated, free articles. Join our Telegram channel

Full access? Get Clinical Tree
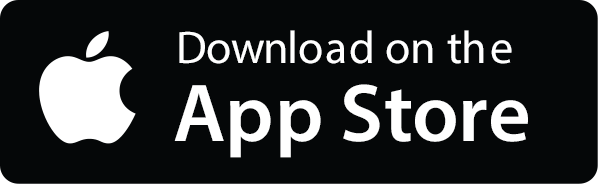
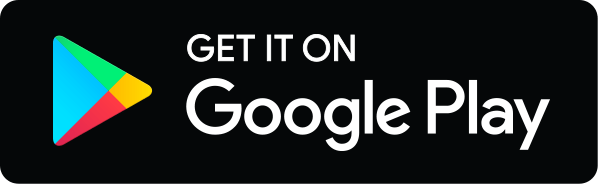