© Springer Science+Business Media New York 2015
S. Hossein Fatemi (ed.)The Molecular Basis of AutismContemporary Clinical Neuroscience10.1007/978-1-4939-2190-4_1919. Cognition, Motor Control and Other Aspects of Autism: A Pragmatic Review
(1)
The Urban Child Institute and the Department of Psychiatry, University of Tennessee Health Science Center, Memphis, TN, USA
(2)
Department of Anatomy and Neurobiology, University of Tennessee Health Science Center, 855 Monroe Avenue, Suite 515, 38163 Memphis, TN, USA
Abstract
Evolutionists point out that ASD’s continued presence in the human population may be because of the need for a few individuals to have extreme focus at the expense of human interaction. Regardless of the validity of this hypothesis, in a modern society autism places a considerable burden on the quality of life for patients and their caregivers. To date, the study of Autistic Spectrum Disorders (ASD) has yielded no specific common cause. This has made diagnosis and treatment difficult. This chapter reviews a variety of variables that have over the past years received clinical and/or animal experimental support for their relation to autism. They range from neuropathologies affecting individual cells or the size of entire brain areas, to considerations of the role of inflammation or environmental factors and autism. Important clues may also come from comparative studies of subjects with ASD and with schizophrenia, two disorders known to be associated with neuropathologies in the cerebellum and prefrontal cortex. Clearly, ASD research now faces the considerable challenge of integrating large amounts of diverse data. Qualitatively new insights will most likely arise from integrative approaches considering interactions between prominent variables.
Keywords
Cerebral cortexCerebellumMinicolumnsEvolutionSensorimotor and cognitive dysfunctionSportsSchizophreniaAnimal modelsIntelligenceEnvironmentStressBrain sizePrefrontal cortexBrain developmentSelfMemoryAutoimmunityLeaky gutDopaminePruningInflammationAutism in humans is a complex array of loosely related conditions rightly called Autism Spectrum Disorders (ASD). A specific cause common to all forms of ASD has not been identified and, as this brief review will demonstrate, most likely never will be because of the substantial array of manifestations and degrees of severity of ASD. Several other facets of understanding ASD, including diagnostic methods, objective measures, and similarities with other neurological conditions, have served to further complicate our knowledge of this spectrum of disorders.
If one compares an autistic brain with one from the general population, there is little in the way of gross abnormalities. Casanova (2005) discusses some of the few differences in his consideration of the minicolumns of cells in the cerebral cortex . He emphasizes the fact that few actual brains of autistic people have been studied. This continues to be a serious problem. Casanova found the cortical minicolumns of the autistic brain to be reduced in size , thus one dendrite will connect with more minicolums in the autistic person than in the normal.
The place of autism in the evolutionary history of mankind is interesting to consider and can give us an insight into the big picture of what autism is. Reser (2011) considers the possibility that autistic individuals during the time of early man fit into the scheme of daily life because food was scarce and the presence of “solitary foragers” could be a distinct advantage because large groups most likely had to disband periodically to survive. He says such an environment would favor the mildly autistic but that assortative mating would occasionally produce the more severe cases of autism. He suggests that autism is not necessarily a disease but a way of suppressing social information to focus on more specific tasks at hand.
Ploeger and Galis (2011) do consider autism a disorder that is detrimental to reproductive success, certainly a negative factor in evolution . They say that autism has not been eliminated by evolution because autism is a polygenic disorder whose genes interact. Probably, they say, these genes are also involved in positive traits, specifically increased intelligence which is related to reproductive success. Their hypothesis is that these genes normally contribute to normal or higher intelligence while unlucky individuals have to live with autism when the genes interact in such a way or spontaneously mutate to leave the individual in this low-fitness extreme.
Further, these authors describe the defensive responses of the autistic individuals as being more like that of the reptilian than the mammalian brain. A mammal tends to dampen the defensive system in response to stress by interaction in social situations while the reptile is in a chronic state of hypervigilance or shutdown. For the reptile and its environment, this strategy works, but for the mammal this is not an adaptive strategy. In other words, the autistic brain is not utilizing the full cognitive capacity of the mammalian brain in reaction to stressors.
In discussing the relationship between sensorimotor and cognitive dysfunction in autism and related disorders we take a general view of the link between brain function in general and motor control. Sessile organisms such as plants, have evolved successfully without the possession of a nervous system. The ability of an organism to move, however, whether in aquatic or terrestrial environments, seems to be inextricably linked with the possession of a nervous system, capable of sensing environmental and internal states and of generating movements appropriate for survival.
Based on evolutionary and neurophysiological considerations it seems possible to argue that cognitive and sensorimotor processes are closely related in terms of their underlying neuronal principles. A quote attributed to the late Walle Nauta (1916–1994) elegantly summarizes this idea: “What is a thought except a movement that is not connected to a motor neuron”. This view implies that the challenges and advantages associated with the ability to move around freely led to the evolution of neuronal mechanisms which can also support the development of complex cognitive processes such as self awareness, planning and decision making. In his 2002 book “I of the vortex”, Rodolpho Llinás (2002) also argues in favor of a common evolutionary origin of the neuronal mechanisms underlying sensorimotor and cognitive processes, suggesting that thoughts are “internalized movements” (Llinas 2002).
The brain structure most implicated in cognitive function is the neocortex, particularly its prefrontal areas, which are not primarily involved in sensory or motor processes.
The question arises as to whether the characteristic neural circuitry of the neocortex can be used to generate both movements and mental processes. The most fundamental forms of movement, such as swimming, walking, breathing or chewing, are also the ones most relevant for survival and basic life support. These movements are typically highly rhythmic and controlled by semi-autonomous neuronal circuits called “central pattern generators” (CPGs), located in the spinal cord and brain stem (Grillner 2006; Harris-Warrick 2010). A characteristic feature of CPGs is that they can generate functional output independent of sensory inputs but can be modulated by sensory activity or top-down signals from other parts of the brain. In an opinion article that appeared in the journal Nature Review Neurocience, Yuste and colleagues argued that neocortical circuits could be considered analogous to CPGs as the neocortex is able to generate output in the absence of sensory inputs but can also be strongly modulated or engaged by sensory or other inputs (Yuste et al. 2005).
Together the above ideas suggest that sensorimotor and cognitive processes cannot be considered independent of each other as they are generated by circuits based on common neuronal principles and consequently common vulnerabilities to disease. As a consequence, pathological changes affecting the function of basic neocortical circuits will inevitably cause both sensorimotor and cognitive deficits and those would not be limited to a specific cognitive disorder but rather apply to a broad spectrum of those.
Too often, generalizations about the effect of autism on motor function have, to a great extent, ignore the fact that a significant number of people with autism have become celebrated athletes, even in sports requiring keen sensorimotor coordination such as archery, surfing, weightlifting, running, and karate. These accomplishments are beyond what is often seen in special Olympics and amateur sports. Often the athletes themselves have said ASD has in a way been an advantage because the condition allows intense focus and the ability to isolate themselves from what would otherwise be considered the hyper-excitement of the moment (See the extensive number of reports on the popular autism internet general information sites concerning this and including discussion of people successful in such a diverse array of sports. Examples: http://www.attentionlearningcenter.com/add-athletes-autism.htm, http://autismandathletics.wordpress.com/about/). Indeed, that this is possible demonstrates that autism is a spectrum of disorders.
Two prominent cognitive disorders, autism and schizophrenia , are also associated with neuropathology of the cerebellum, a brain structure long believed to be exclusively involved in the coordination of movements and motor learning. However, recent years have seen a fast accumulation of evidence for a role of the cerebellum in cognition as well (Schmahman 1998; Strick et al. 2009). The neocortex and the cerebellum have coevolved and are heavily interconnected, suggesting a close functional interaction between the two structures, which is likely to be affected in ASD brains (Heck and Howell 2013). Here we approach the question of the relationship between motor and cognitive deficits in autism and other cognitive disorders by considering the neocortex and cerebellum as a functional entity and by reviewing known and proposed mechanisms of cerebro–cerebellar interaction.
Fatemi et al. published an exhaustive review of the pathological role of the cerebellum in autism (2012). The authors concluded their paper with the caution that “More definitive behavioral tests are required to confirm the validity of animal models for autism”.
Autism in the human presents both a positive and a negative opportunity for understanding the disorder. Invasive experiments are limited to animal models, but behavioral and clinical observations and direct questioning of patients are equally important and useful, provided they are conducted with sufficient caution.
Many primary questions about autism are still much argued in the literature. A good example of this is a study described by Dawson et al. (2007) concerning the level of autistic intelligence compared to that of non-autistic control subjects. Their conclusion was that the intelligence of autistic people has been too often underestimated, but the true value of the paper was their careful and systematic development and presentation of their methodology. This was a refreshing, educated insight into the nature of autism.
Of course we know that genetic mutations have been linked to autism. It is thought these could number in the hundreds (Buxbaum et al. 2012). Epigenetics also plays a role (Shulha et al. 2012), just as it does in normal brain development.
Epigenetics would be expected to play a role in the development of autism in response to stress. In fact, stress has been implicated and studied extensively by many as being a possible cause of ASD.
Prenatal stressors were correlated with an increased incidence of autism if they occurred during 21–32 weeks of pregnancy, with a peak between 25 and 28 weeks (Beversdorf et al. 2005). This was seen to be consistent with particular pathological outcomes of neuroanatomical development, e.g. showing pathological changes in the cerebellum, but the authors called for more studies related to their findings.
Kern and Jones (2006) implicated neuronal cell death, and brain damage from insult after birth caused by the effects of toxicity and oxidative stress . This, they said, was related to the loss of Purkinje cells found in autism and the related increase in the volume of the brain.
Eric Courchesne and others have taken good advantage of the limited amount of brain tissue available from autistic people who died and donated their brains. From these studies he found a 67 % increase in the number of brain cells of the prefrontal cortex when compared to corresponding tissue from people of the same age who were not autistic (Courchesne et al. 2011). Brain weight and overall size were also increased in autistic individuals (Courchesne et al. 2011). This was consistent with earlier reports of autism being related to overgrowth of the head and brain in the frontal region. In fact, Stanfield et al. (2007) pointed out that in autistic subjects they studied with magnetic resonance imaging (MRI), although the corpus callosum was reduced, the total brain, the caudate nucleus, and the cerebral hemispheres exhibited an increased volume.
Several investigators over the years have discussed the overgrowth of nerve cells in the prefrontal cortex as characteristic of autism and Courchesne’s continuation of this study has quantified some specific processes leading to this condition. The lack of selective pruning of collaterals during development in this region of the brain seems to be a primary factor. We know that pruning of nerve cells is a major component of brain development in the early years of life. This has been studied in several specific areas of brain development. One of the most striking investigations of this was of the role of nerve cell pruning in the development of stereo vision (Ding and Elberger 2000, 2001). Improper pruning would lead to impaired vision or even blindness. In the case presented by Courchesne et al. (2011) the massive prenatal build up of nerve cells resulting from lack of pruning in the prefrontal cortex might leave the individual with increased “neuronal noise” in this portion of the cognitive system.
Evidence suggests that the cerebral–cerebellar connection is greatly impaired in autism (Skoyles 2002) and this portion of the problem seems to be a central factor of the pathology of autism. That cerebral–cerebellar function of fine-tuning movement and muscle action is impaired, is easily seen in many people with autism because of their lack of smooth movement and various other motor deficits (Donnellan et al. 2013). On a simplistic level, this is understandable considering that it is generally accepted that the prefrontal cortex normally takes longer to mature than the cerebellum and, in autism, the communication between the cerebellum and prefrontal cortex is likely to be impaired due to the overpopulation of the prefontal cortex with nerve cells and the possibly resulting increase in “noise” (Courchesne et al. 2011).
Bernard Crespi (Crespi 2010; Crespi et al. 2010) hypothesized that autism spectrum disorder (ASD) and psychotic-affective spectrum disorders (schizophrenia) both involve problems with social interaction. He characterized ASD as exhibiting an over-development and schizophrenia as exhibiting an under-development of what he called the “human-specific social brain phenotypes.” He and his colleagues supported their view with studies of how autism risk alleles affect manifestations of the disorder and through analysis of the literature. Developmental neurogenesis work and physiological and genetic studies of synaptic function in the mouse support Crespi’s hypothesis (Kaphzan et al. 2012; Heck and Lu 2012; Hoerder-Suabedissen et al. 2013).
Another comparison between autism and schizophrenia can be made relative to the awareness of self or self-consciousness. Schizophrenia has been called a disorder of self which can take various forms and to varying degrees, but there is a general distortion of consciousness and lack of self presence (Sass and Parnas 2003). Autism can also be characterized by a lack of self-consciousness, but high-functioning autism or people with Asperger syndrome may have or acquire self-consciousness through learning (Frith and Happe 1999). Memory tests by Toichi et al. (2002) also revealed deficits in the consciousness of self by people with autism. It is noteworthy that many of the genes implicated in autism and schizophrenia are active only during specific stages of the developing brain (Hoerder-Suabedissen et al. 2013), suggesting the existence of critical periods for the normal development of consciousness of self.
Self in people with autism is often compromised because memories are persistent, not affected by emotion or discussed with others (Jordan 2008). Access to memories is not efficient and the memories are often not coherent. The proper handling of memory needs to be taught because otherwise it is difficult to ascribe particular memories as part of self. Because the autistic person can have obsessive chains of negative thoughts making him or her vulnerable to anxiety with depression being many times the result (Jordan 2008).
Raison et al. (2006) pointed out that stress (anxiety would certainly be a stress) can lead to inflammation in the nervous system associated with the pathogenesis of depression. In this situation, it becomes a difficult “chicken or the egg” question. These authors cite many studies which explore the association of inflammation and depression continuing through the adult life of the individual. Using brain tissue from the autopsies of 11 autistic patients, neuroinflammatory markers were found in the cerebellum and other areas by Vargas et al. (2004). The authors also point out that neuroglial reactions are manifested by immune responses, focused in the resulting chronic inflammatory condition of the cerebellum. The detected presence of antiinflammatory cytokines and proinflammatory chemokines support their conclusion that this condition is a characteristic of some autistic brains. They believe addressing these neuroglial conditions might be a viable therapy in some people with ASD.
We know that adverse neonatal experiences can alter brain development (Anand and Scalzo 2000). Brain inflammation is often hypothesized as a possible cause of several neurological disorders. Alzheimers is just one that is pointed out as a disease possibly resulting from brain inflammation. See the paper by Yao et al. (2004) as just one study in support of this concept.
One review of the literature (Angelidou et al. 2012) revealed a consensus that several genes exist which can make an embryo susceptible to autism, but environment is a big factor leading to the actual development of autism. A partially developed gut–blood–brain barrier would not protect against neurotoxins and stressors can cause autoimmune problems for the mother, which can make the embryo susceptible to the development of autism. Thus, stress can lead to brain inflammation, resulting in autism for embryos genetically predisposed to this disorder.
This view supports the earlier work of Pardo et al. (2005) who strongly suggested environmental factors and stressors could combine with genetic susceptibility in increasing the possibility an embryo would develop autism.
These two latter papers also emphasized the importance of the presence of an autoimmune condition and the role of auto-antibodies and neuroglia in the brain’s reaction to stress.
One argument has been presented in the literature, supported by several researchers, that schizophrenia and some other autoimmune diseases, such as autism, could result from what has been termed the “leaky gut” in which neuropeptides of food proteins escape the gut and get past the blood-brain barrier resulting in CNS dysfunction (Dohan 1988; Eaton et al. 2006). Of course, inflammation is part of the mechanism of this insult on the brain and resulting inflammation can come from many sources, both internal and external.
There is a wealth of epidemiological evidence that the environment might affect the prevalence of ASD in certain geographical areas (Fombonne 2005, 2006). We have not addressed this here. However, the importance of the environment on the occurrence of autism is quite apparent from an extensive recent study by Suren and colleagues (Suren et al. 2013) of over 85,000 children. Those children whose mothers took folic acid around the time of conception had a distinctly lower risk of autistic disorder than those whose mothers did not. This and similar studies strongly suggest that more effort should be directed towards the study of environmental factors in ASD.
Experiments in animals, in particular in genetic mouse models of ASD, provide important clues as to possible neuronal pathways and mechanisms of interaction between the cerebellum and the cerebral prefrontal cortex and their involvement in autism. Tracing studies in primates have shown that the cerebellum projects (via the thalamus) to the prefrontal cortex (Middleton and Strick 2001). In turn, the prefrontal cortex projects back to the cerebellar areas from where prefrontal cortical projections originated, forming what might amount to parallel loops of cerebral–cerebellar connections (Kelly and Strick 2003). While these anatomical connections clearly suggest targeted neuronal interactions between the two structures, how cerebellar activity modulates prefrontal cortical activity and vice versa and how their interaction is related to behavior is poorly understood.
Recent experiments in mice have revealed a surprising new aspect of prefrontal cortical interaction with the cerebellum. When stimulating the cerebellar dentate nucleus in healthy mice, Mittleman and colleagues discovered an increase in dopamine release in the medial prefrontal cortex (Mittleman 2008). Dopamine is a neuromodulatory transmitter generated by cells in the substantia nigra and ventral tegmental area (VTA) and is most widely known for its importance in the failure and disinhibition of movement initiation in Parkinson’s and Huntington’s disease respectively (Graybiel et al. 1994). But dopamine is also strongly implicated in reward and pleasure seeking behavior, drug addiction and cognitive functions (Iversen et al. 2010). Abnormal function of the dopaminergic system has been implicated in a variety of cognitive disorders including autism spectrum disorders and schizophrenia (Dichter et al. 2012a, b; Sesack and Carr 2002). Cerebellar controlled dopamine release in the mouse medial prefrontal cortex was mediated by two independent and equally contributing pathways, one involving the ventral tegmental area (VTA) which contains dopaminergic cells projecting to the prefrontal cortex, the other involving the thalamus (Rogers et al. 2011). Increased dopamine release via cerebellar activated thalamic projections involved stimulation of mesocortical dopaminergic terminals via appositional excitatory glutamatergic synapses (Del Arco and Mora 2005; Pinto et al. 2003). A recent study in a mouse model of fragile X syndrome, an autism spectrum disorder, showed a reorganization of the pathways involved in cerebellar modulation of mPFC dopamine release resulting in a weakening of the VTA and a strengthening of the thalamic pathway via the ventro-lateral nucleus (Rogers et al. 2013). Together with the known cerebellar deficits associated with autism (Courchesne 1997; Skoyles 2002; Webb et al. 2009; Fatemi et al. 2012) these findings suggest that a dysfunction of cerebellar dependent reward circuits may play a role in at least some forms of autism. Consistent with this hypothesis are recent findings by Dichter and colleagues who used functional magnetic resonance imaging (fMRI) to compare reward circuit responses in autistic and control subjects and reported hypo-activation of reward circuit activity in autistic individuals (Dichter et al. 2012b). While more studies are needed to determine how strongly the cerebellum is involved in reward seeking tasks like those chosen by Dichter and colleagues, a role of the cerebellum in human cocaine-related behavior has already been demonstrated (Anderson et al. 2006). Together these studies suggest that the cerebellum modulates prefrontal cortical activity via dopamine, thus contributing to a broad spectrum of sensorimotor and cognitive functions, especially behaviors involving reward seeking and positive reinforcement (Iversen et al. 2010).
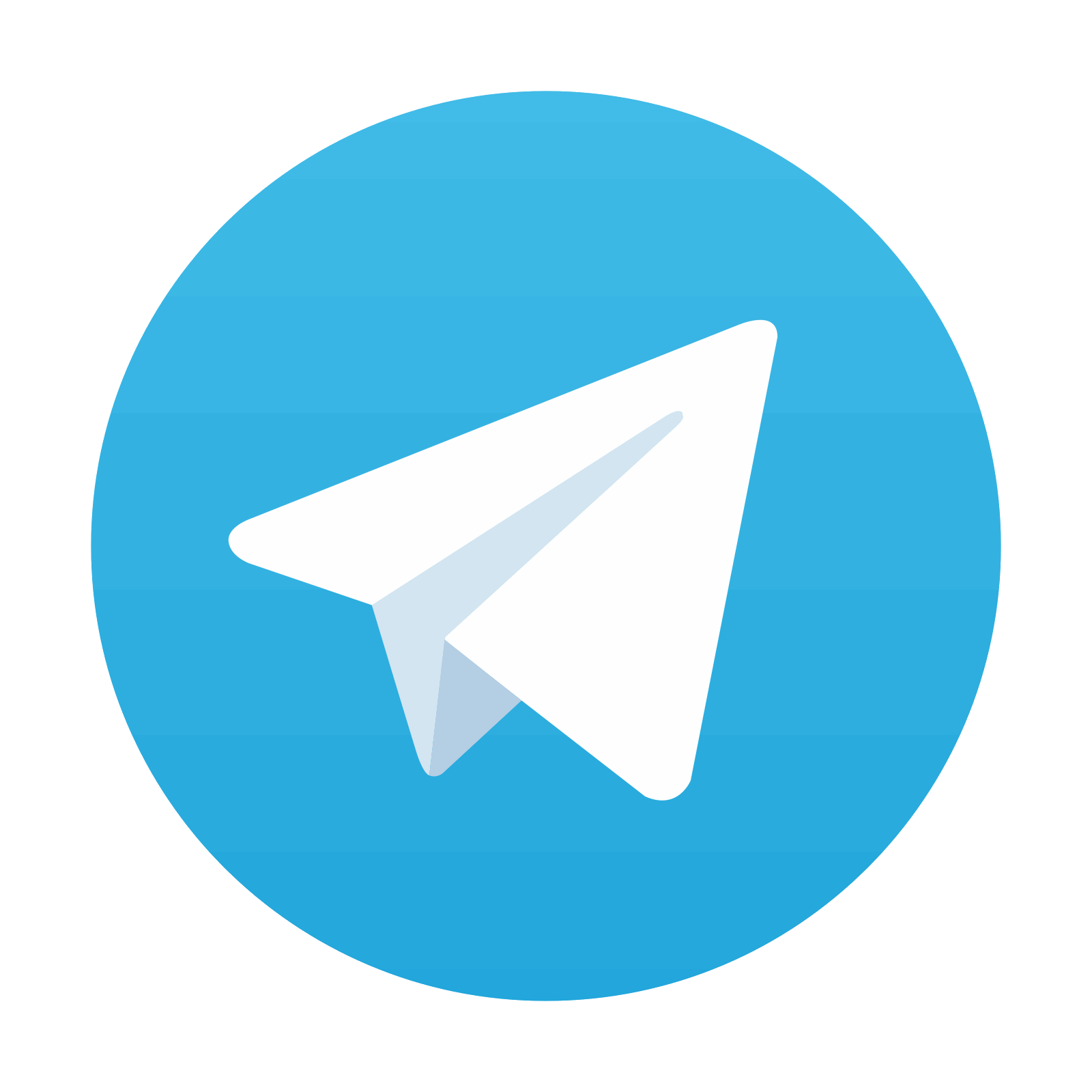
Stay updated, free articles. Join our Telegram channel

Full access? Get Clinical Tree
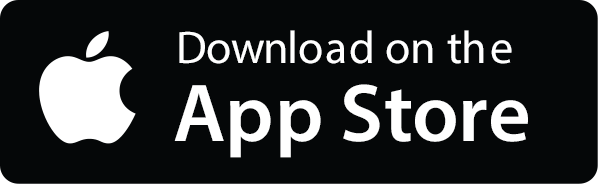
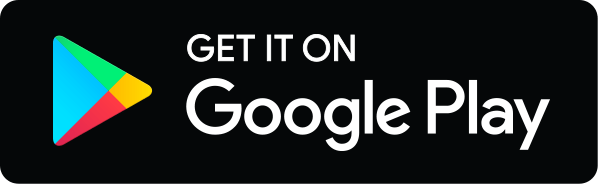