Fig. 12.1
Multifactorial etiology of cognitive dysfunction in cancer patients
As disruption in neurocognitive function can be a major cause of impaired quality of life, attention to these symptoms and consideration of possible therapeutic interventions directed to improve neurocognitive deficits have become increasingly important in clinical oncology and cancer survivorship. Importantly, a specific neurocognitive deficit (e.g., impaired short-term memory or executive dysfunction) might result in varying degrees of disability in a given patient, depending on the individual’s baseline cognitive function, cognitive reserve, and on how such deficits interfere with a patient’s ability to perform social and professional activities. Therefore, patient evaluation and choice of therapeutic intervention must be individually tailored to the patient’s perceived deficits and specific needs and evaluated in the context of the patient’s personal and professional life.
Tumor Effects on Neurocognitive Function
Impairment or change in neurocognitive function is usually dependent on the location, size, type, and biology of the underlying tumor [1–4]. Accounting for all of these factors, it has been hypothesized that cognitive dysfunction in brain tumor patients is the result of a whole brain network disturbance [5, 6]. Tumor-associated complications, such as cerebral edema, seizures, or use of anti-seizure medications are also known to influence neurocognitive performance [5, 7–10].
The current literature suggests that neurocognitive deficits are frequently evident prior to tumor directed therapies in brain tumor patients [4, 11]. For instance, neurocognitive dysfunction was present in 90% of patients with frontal or temporal gliomas prior to any therapeutic intervention, with impairment of memory and attention in 60% and executive dysfunction in 78% of patients [12].
The influence of tumor pathology and degree of malignancy on neurocognitive function remains poorly understood. Interestingly, impairment of attention and executive dysfunction are considered unfavorable prognostic markers in patients with high-grade gliomas [13]. It is conceivable that neurocognitive and behavioral deficits more easily result from rapidly growing malignant brain tumors than from low-grade or benign tumors, in which long-term compensatory mechanisms might play a role in the maintenance of cognitive function [3, 14–16]. However, other studies have not revealed a correlation between cognitive performance and degree of malignancy. For instance, Kayl and Meyers were unable to identify a significant difference in cognitive performance between glioblastoma and anaplastic astrocytoma patients, accounting for patient age, gender, education, tumor location, and tumor volume [17].
The development of specific neurocognitive deficits depends on the location of the tumor within specific brain regions. For example, tumors that affect the dominant hemisphere and fronto-temporal region are likely to cause disruption in language function, including verbal learning, memory, and speech [3]. Nondominant hemispheric tumors are more likely to result in impairment of nonverbal speech, visual-spatial, and visual-perceptual functions [1, 18].
Frontal lobe tumors commonly cause executive dysfunction and personality changes, including a lack of motivation, spontaneity, and impaired social judgment. Parietal lobe tumors commonly result in difficulties with spatial orientation, and tumors in the occipital lobes can cause visual-perceptive deficits. Neurocognitive and mood disorders have also been described in patients with cerebellar tumors [19–23] and brainstem lesions [24], supporting the notion that the cerebellum has important functions in neural network systems relevant to higher cognitive function.
Treatment Effects on Neurocognitive Function
Radiation Therapy
Cranial radiation therapy is well known to cause neurocognitive deficits [25–29]. Radiation can result in progressive cognitive decline in both children [30–33] and adults [25, 34–36]. The effects of cranial radiation have been best studied in patients treated with whole brain radiation therapy (WBRT) (e.g., [37]), though emerging data reveal that focal and stereotactic radiosurgery (SRS) can also result in structural brain changes and significant functional morbidity in a subset of patients [38, 39].
In general, risk factors for developing radiation related central nervous system toxicity include age (with neurotoxic complications more likely to occur at age <5 years and >60 years), a fractionation dose of >2 Gy, cumulative radiation dose, volume of brain irradiated, shorter overall treatment time, concurrent or subsequent use of chemotherapy, and preexisting cerebrovascular risk factors [25, 40–42]. In addition, genetic factors are likely to play a role in tissue vulnerability to radiation [43–45].
Cognitive dysfunction has been reported in up to 85% of patients treated with cranial radiation therapy [42]. A wide range of reported incidences is conceivably explained by differences in patient populations, treatment variables, and study methodology.
Radiation-induced neurotoxicity usually follows a progressive pattern, and long-term survivors are at particular risk to demonstrate cognitive decline, such as children treated with prophylactic cranial radiation for childhood leukemia [46]. The use of concurrent or sequential chemotherapy can increase the incidence and severity of radiation-induced toxicity [25]. For example, patients with primary central nervous system lymphoma (PCNSL) treated with whole brain radiation and subsequent chemotherapy are at particular risk for profound cognitive impairment; imaging findings can demonstrate brain volume loss and leukoencephalopathy [47]. Recent studies also revealed a surprisingly high degree of early onset and progressive brain atrophy in glioblastoma patients treated with combined standard temozolomide chemotherapy and focal brain radiation [39].
In general, radiation-induced neurotoxicity has been categorized in three phases: an acute, early delayed, and late-delayed reaction [42, 48].
In the acute phase, symptoms occur within days to weeks of therapy and are usually characterized by development of fatigue, subacute neurological decline, and worsening of preexisting deficits, which fortunately is reversible in most patients with supportive management. Symptoms are likely the result of inflammatory mechanisms, vascular toxicity, blood–brain barrier disruption, and cerebral edema [49, 50].
Early delayed neurotoxic symptoms occur within weeks to several months of treatment. Symptoms mainly consist of fatigue, inattention, and short-term memory impairment. Neurocognitive testing may demonstrate slowed information-processing speed, word and memory retrieval deficits, diminished executive function, and impaired fine motor dexterity [25, 51–53], suggesting impairment of orbito-frontal and fronto-temporal network systems.
Early delayed neurotoxic symptoms are thought to be related to radiation-induced transient demyelination [54].
Late-delayed side effects occur months to years after radiation and are mostly irreversible and progressive. Deficits in memory, attention, concentration, and executive dysfunction are frequently reported [55]. Most patients will demonstrate mild to moderate cognitive impairment, but only infrequently are these associated with abnormal imaging findings [56]. However, in more severe cases of late-delayed radiation toxicity, patients will develop progressive cognitive decline [27], and imaging and histopathological studies may demonstrate focal necrosis, leukoencephalopathy, and global brain atrophy [47, 57–62]. The current data suggest that nonspecific diffuse white matter changes can be seen on MRI in nearly all patients receiving doses of 20 Gy or higher who survive more than one year [63]. The risk of white matter changes following WBRT is higher in patients with baseline white matter changes [64].
Concerns regarding delayed radiation-induced neurotoxic adverse effects in long-term survivors have stimulated a contentious debate on the preferential use of SRS, WBRT, or a combination of both in patients with brain metastases [65]. One of the main questions is whether patients with a limited number of brain metastases should be treated with either WBRT or with SRS [66, 67]. With comparable survival data in patients treated with either modality, the benefit of WBRT has been challenged especially in patients with limited number of cerebral metastases and in light of more pronounced neurocognitive deficits (e.g., learning and memory decline) following WBRT [63, 67].
WBRT has also been shown to impact patient–reported quality of life. A large multi-institutional study of patients with one to three brain metastases revealed that WBRT, when added to SRS, was associated with more pronounced decline in patient-reported cognitive functioning at one year compared with patients that were treated with SRS alone [68]. Other studies using prophylactic cranial radiation in lung cancer patients revealed similar results [69].
Partial brain radiation may also have negative long-term effects on neurocognitive function, even though the risk of developing neurotoxicity is lower than with whole brain radiation therapy [70, 71]. Nevertheless, cognitive dysfunction, including impaired executive function, poor attention, and reduced information processing, can result in significant impairment of quality of life and overall performance in long-term survivors, such as in patients with low-grade gliomas [72, 73].
The precise mechanisms of chronic radiation toxicity are not entirely understood but likely include chronic inflammatory responses and damage to various neuroglial cell types, including neural progenitor cells and myelin producing cells [74–77]. Disruption of adult hippocampal neurogenesis has been identified as one of the relevant physiological processes driving cognitive decline following cranial irradiation [78].
There have been increasing efforts to minimize the negative impact of radiation on germinal zones of the brain. For instance, in a phase II multicenter study (RTOG 0933) Gondi and colleagues demonstrated preservation of cognitive function with conformal avoidance of the hippocampus during WBRT in patients with brain metastases [79]. In addition, pharmacological interventions have been explored to improve cognitive function in patients treated with cranial radiation [80]. For instance, the acetylcholinesterase inhibitor donezepil has shown promise to improve executive function and memory performance in both childhood brain tumor survivors [81], and cognitive function, quality of life, and mood in adult brain cancer patients treated with cranial irradiation [82]. Moreover, Brown and colleagues demonstrated in a recent randomized, double blind, placebo-controlled clinical trial that memantine, an NMDA receptor antagonist, was associated with delayed onset and improved cognitive function in adult patients treated with whole brain radiotherapy for brain metastases [83].
Chemotherapy
Chemotherapy can result in cognitive dysfunction, which has been most convincingly demonstrated in patients treated for non-CNS malignancies. The most frequently described neurocognitive problems include difficulties with memory, learning, attention, concentration, information-processing speed, organization, and executive function (Fig. 12.2).


Fig. 12.2
Cognitive domains commonly affected by cancer therapy
Interestingly, neurocognitive deficits may be of delayed onset and can be progressive even years after cessation of anticancer therapy. Neurocognitive adverse effects from chemotherapy have long been recognized as a disturbing problem in long-term survivors of childhood cancer [27–29, 84, 85]. Similarly, studies in adult cancer patients have demonstrated that chemotherapy-associated cognitive dysfunction is more common than previously anticipated [42, 86–97].
Incidences of chemotherapy-associated cognitive dysfunction range from 15 to 95% [89, 93, 98, 99]. This wide range of reported incidences has been attributed to the use of different neuropsychological tests with varying degrees of sensitivities to detect cognitive deficits, differences in study design (retrospective vs. prospective design, longitudinal versus cross-sectional analysis, etc.), and different patient populations studied.
Cognitive impairment has been demonstrated in patients treated for various solid cancer types [100–104]. The most compelling data exist in breast cancer patients, showing that approximately 20–40% of breast cancer patients have cognitive deficits on post-treatment evaluation [93, 97, 105–113]. Long-term evaluation of patients examined 1–2 years after treatment with high-dose chemotherapy demonstrated that cognitive impairment is notable in approximately 30–60% of patients [87, 107, 114].
While some patients experience neurocognitive deficits only transiently during and immediately after chemotherapy, symptoms may persist in other patients for years and can cause considerable decline in quality of life, preventing affected individuals to return to their previous level of academic, occupational, or social activities [115]. A recent study of nearly 200 breast cancer survivors who had undergone adjuvant chemotherapy more than 21 years prior found deficits in verbal memory, processing speed, executive functioning, and psychomotor speed when compared with a healthy reference group [116].
Neurocognitive adverse effects have been observed with virtually all categories of chemotherapeutic agents (Table 12.1) [26, 93, 117–119], including antimetabolites (e.g., cytosine arabinoside, 5-fluorouracil, and methotrexate), DNA cross-linking agents (e.g., nitrosureas and cisplatin), mitotic inhibitors (e.g., vincristine), and anti-hormonal agents (e.g., tamoxifen). Some agents, such as methotrexate and carmustine (BCNU), are well known to cause cognitive deficits and leukoencephalopathy, especially when administered at high doses, intrathecally, or in combination with cranial radiotherapy [120–123]. However, imaging abnormalities on MRI only infrequently correlate with the degree of cognitive deficits [124, 125].
Table 12.1
Chemotherapeutic agents associated with cognitive dysfunction in cancer patients
Chemotherapeutic agent |
---|
∙ ACNU/CCNU/BCNU (Nitrosureas) |
∙ Carmofur |
∙ Cisplatin |
∙ Corticosteroids |
∙ Cyclophosphamide |
∙ Cytosine arabinoside (Ara-C) |
∙ Dacarbazine |
∙ Fludarabine |
∙ 5-Fluorouracil (5-FU) |
∙ Ifosfamide |
∙ Interferon |
∙ Levamisole |
∙ Methotrexate |
Experimental studies have revealed insights into the underlying mechanisms of cognitive dysfunction in cancer patients. Chemotherapy-induced damage to neural progenitor cells required for neurogenesis, gliogenesis, and white matter integrity throughout life has been identified as an important etiologic factor [78, 126–132], and has offered a compelling explanation for delayed and progressive neurotoxic effects of chemotherapy.
Importantly, chemotherapeutic agents can affect brain function through direct cytotoxic effects or indirectly through vascular injury, pro-oxidative changes, and immune-mediated inflammation [29, 47, 133–138]. However, the etiology of cognitive dysfunction during and following chemotherapy is likely multifactorial. Risk factors of cognitive decline include timing of chemotherapy, combination of chemotherapy with radiation therapy, and patient age. Metabolic alterations, hormonal imbalance, fatigue, the level of cognitive function prior to treatment and other medical comorbidities have also been shown to influence the manifestation of cognitive symptoms [2, 42, 96]. In addition, certain genetic risk factors have been identified to modulate the degree of cognitive dysfunction. For instance, polymorphisms that influence the efficiency of DNA-repair mechanisms and drug efflux pump systems may expose certain individuals to a higher risk of developing central nervous system toxicity [139–149].
Diagnostic Considerations
The application of a comprehensive testing battery to capture the full range of neurocognitive and psychological function can be time consuming and may be difficult to administer in a demanding oncology practice. In general, assessment of neurocognitive function requires reliable, quantifiable, and valid measures sensitive to cognitive and quality of life deficits in cancer patients. Longitudinal assessment of the patient’s self-perception of cognitive function is equally important for patient management. Testing strategies that closely relate to real-life situations during which cognitive deficits readily manifest are considered most relevant. It has been an ongoing effort to develop guidelines regarding selection of neuropsychological tests for efficient and comprehensive assessment of cognitive function that can be uniformly applied to cancer patients and allow for comparable data analysis [97, 150–152].
Neuropsychological evaluations are conducted to assess a range of cognitive functions. The neuropsychologist chooses tests that are relevant to the cognitive concerns of the patient, family, or physician and interprets test performance, facilitating care for the patient’s cognitive and behavioral symptoms (Table 12.2). The application of a comprehensive testing battery to capture the full range of neurocognitive and psychological function can be time consuming and may be difficult to administer in a multi-site clinical trial. A brief neurocognitive testing battery conceptualized to evaluate memory, visual motor function, executive function, motor dexterity, verbal fluency, and functional independence has been successfully used in several clinical trials by the Radiation Therapy Oncology Group (RTOG) and North Central Cancer Treatment Group (NCCTG) . This testing battery has been shown to be sensitive to important cognitive symptoms, time efficient, and easy to administer [153]. Assessment of cognitive function, Quality of Life, and functional status has been shown to provide clinically relevant information in clinical trials assessment of novel treatment strategies [154, 155]. An improved understanding of neurocognitive sequelae is integral part of clinical management and allows clinicians, caregivers, and patients’ families to address important needs of cancer patients.
Table 12.2
Neurocognitive testing battery
Neurocognitive domain | Typical neuropsychological tests |
---|---|
Motor dexterity/speed | Lafayette grooved pegboard testa |
Visuospatial perception | Benton judgment of line orientation |
Block design subtest of the WAIS-IV | |
Language | Boston naming test |
Vocabulary subtest of the WAIS-IV | |
Category fluency test | |
Information processing speed | Trail making test Aa |
Digit symbol subtest of the WAIS-IV | |
Working memory/attention | Digit span subtest of the WAIS-IV |
Paced auditory serial addition test | |
Recent/episodic memory | Hopkins verbal learning testa |
Brief visuospatial memory test | |
Wechsler memory scales-IV | |
Executive function | Controlled oral word associationa |
Trail making test Ba | |
Wisconsin card sorting test |
Collectively, rigorous neurocognitive evaluation using standardized testing batteries will be important for optimal management of cancer patients. In addition, the identification of biomarkers to identify and monitor chemotherapy-associated cognitive changes remains an important objective of ongoing investigations. Recent translational imaging studies using structural MRI, functional MRI, and PET imaging have identified compelling evidence for chemotherapy-related brain damage [155, 156]. Advanced neuroimaging studies are therefore likely to increasingly complement future patient evaluation and neurocognitive assessment in cancer patients.
Treatment Considerations
There are currently no standard therapies for patients who experience cognitive symptoms following chemotherapy. While some agents have shown promising results in pilot studies, larger prospective and randomized clinical trials are missing and definite treatment guidelines have so far not been established. Increasing our understanding of the detailed mechanisms underlying cognitive dysfunction in cancer patients will be most relevant to develop neuroprotective therapies. Therapeutic interventions currently include strategies to either prevent side effects or to minimize the severity of symptoms.
Table 12.3 highlights some therapeutic interventions that have shown a potential clinical benefit in treatment of cancer therapy-associated neurocognitive side effects. For instance, psychostimulants such as methylphenidate [157–161] and modafinil [162, 163] are promising agents for treatment of cancer therapy-related cognitive dysfunction. In addition, donepezil—a reversible acetylcholinesterase inhibitor used to treat Alzheimer’s type dementia—has shown to improve cognitive function, mood, and quality of life in patients with brain and small cell lung cancer [82, 164].
Table 12.3
Treatment strategies for cancer therapy-associated cognitive dysfunction
Agent or intervention | References | Study population |
---|---|---|
Methylphenidate (Ritalin®, Novartis, Basel, Switzerland) | Breast cancer, ovarian cancer, childhood cancer, brain tumors, melanoma | |
Modafinil (Provigil®, Cephalon, Frazer, PA, USA) | Breast cancer | |
Donepezil (Aricept®) | Small cell lung cancer and brain cancer | |
Erythropoietin (Procrit®, Janssen Biotech, Horsham, PA, USA; Aranesp®, Amgen, Thousand Oaks, CA, USA) | Breast cancer, lung cancer, ovarian cancer, endometrial cancer, brain cancer and hematological cancer | |
Neurocognitive rehabilitation | [170] | Breast cancer |
Meditation | [172] | Various cancer types |
Exercise | Breast cancer and melanoma | |
Sleep regulation | [175] | Various cancer types |
Optimal management of fatigue, mood disorders, and other comorbidities (e.g., treatment of anemia) needs to be an integral part of patient management [115, 165–169]. Cognitive and behavioral intervention strategies used in patients with stroke and traumatic brain injury may also be employed in patients with chemotherapy-associated cognitive dysfunction [170]. These interventions often focus on compensatory strategies, stress management, energy conservation, and psycho-education. External memory aids (e.g., memory notebooks, pagers) have been among the most widely used interventions [171]. Other non-pharmacological interventions include meditation [172], exercise [160, 173, 174], and regulation of sleep [175].
Mood and Other Psychiatric Disorders in Cancer Patients
Introduction
Cancer patients have a high risk of developing mood alterations or other psychiatric illness [176]. Depression , anxiety, adjustment disorder, neurobehavioral, and neurocognitive changes are common and collectively affect the majority of cancer patients [177, 178].
Moreover, nonspecific emotional distress through the combined impact of social, psychological, and religious or spiritual stressors is highly prevalent in cancer patients, ranging from approximately 15–45% [179]. The National Comprehensive Cancer Network recommends screening for mood and psychiatric illness routinely in all cancer patients [180], and some guidelines for patient evaluation have been released (e.g., [181, 182]).
Psychiatric and Neurobehavioral Symptoms in Brain Tumor Patients
Among all cancer patients, brain tumor patients are considered at highest risk of developing neuropsychiatric symptoms during their course of disease [183, 184]. Quality of life studies have identified a high degree of depression, anxiety, and reduced family functioning in brain tumor patients [185].
Notably, changes in mood, cognition, behavior, and personality can be the initial sign or symptom of a tumor of the central nervous system [186]. In addition, neuropsychiatric conditions can occur as a consequence of cancer treatment, including cranial irradiation [187], chemotherapy [119, 188, 189], and various other medications (e.g., corticosteroids) [190–193]. Therefore, clinicians need to be aware of neuropsychiatric symptoms in brain tumor patients at time of diagnosis and throughout their course of the disease in order to recognize symptoms early and to initiate appropriate treatment as soon as possible. For instance, more than 90% of patients with high-grade gliomas reported symptoms of depression prior to and up to six months after surgery [194].
Neuropsychiatric symptoms are usually nonspecific in terms of tumor localization, and also do not strictly correlate with the type or the degree of malignancy [194, 195]. Brain tumors in frontal and temporal brain regions may produce varying degrees of neurobehavioral changes, including mood alterations, impaired judgment, inattentiveness, irritability, memory deficits, and frank dementia [196–198]. Patients with tumors affecting the corpus callosum, cingulate gyrus and deep and midline brain areas frequently demonstrate neurobehavioral symptoms, personality changes and affective disorders, reported in up to 90% of patients [199]. Psychotic symptoms (e.g., paranoid delusions) can be part of the presentation [198]. Tumors affecting the cerebellum and neural pathways within the cerebellar network system may also produce neurocognitive deficits, psychotic symptoms, and mood alterations [200].
Psychiatric and Neurobehavioral Symptoms in Patients with Cancer Outside the Nervous System
The prevalence of mood alterations is significantly higher in cancer patients when compared to the general population. Approximately 10–50% of cancer patients are considered to have some emotional distress or depression [176, 201, 202].
Cancer patients frequently report some degree of anxiety. Anxiety can have several etiologies in cancer patients and can occur alongside or independent of a mood alteration. As physical, psychological, or cognitive symptoms can be the lead manifestation of anxiety, the diagnosis can be challenging [182, 203]. Depression and anxiety are significantly more common in patients with advanced illness, poorly controlled pain, physical deficits, younger cancer patients, and prior history of affective disorders [202, 204–206].
Interestingly, the presence of mood alterations has been associated with poor survival in cancer patients [207–209]. Studying the connection between mood and emotional state on the one hand and the immune system and tumor behavior on the other hand has been an increasingly relevant topic in the field of psychoneuroimmunology [209, 210].
Making matters even more complex, there appears to be a high level of discordance between patient and physician-reported affective disorders, such as depression [194, 211], posing a challenge to the optimal management of cancer patients. In general, a patient’s perception of mood alterations is considered higher when compared with the clinician’s perception. This discordance and underestimation from side of the health care professionals may result in insufficient treatment of patients with such symptoms.
Treatment of cancer patients with adjustment disorder, anxiety, depression, or other psychiatric illness is similar to management of non-cancer patients. Pharmacological and non-pharmacological interventions, such as cognitive-behavioral therapy, supportive psychotherapy, psychosocial interventions, and support groups have been used successfully in cancer patients [212–214].
The choice of a specific intervention or medication will depend on several factors, including the predominant symptoms, comorbid medical conditions, and potential side effect profiles. For instance, a psychostimulant (e.g., methylphenidate or modafinil) may be a reasonable choice as a monotherapy, or in combination with a less sedating antidepressant (e.g., SSRI) for a fatigued cancer patient with depression.
Fatigue in Cancer Patients
Introduction
Fatigue is a major reason of impaired quality of life in cancer patients [217–219]. Fatigue can be associated with the cancer itself [220], or specific kinds of treatment, such as radiation or chemotherapy [221]. Fatigue is usually described as a permanent and distressing sense of tiredness that is not significantly relieved by rest or sleep. Patients may perceive fatigue as physical (e.g., generalized muscle weakness), or mental (e.g., decreased motivation and lack of energy), or a combination of both.
Prevalence and Epidemiology
The majority of cancer patients experience fatigue during their course of disease [222]. The current literature suggests a prevalence of fatigue in over 60% of patients [223, 224]. In patients with a diverse group of underlying cancers, fatigue was present in 50–75% of patients at time of diagnosis, but was even more common throughout their course of treatment, including chemotherapy or radiation, affecting up to 93–95% of patients [224]. While the majority of patients report significant fatigue during active therapy [225], fatigue can persist for years after cancer treatment completion and can be a major cause of chronic morbidity in long-term survivors [220]. Notably, chronic fatigue in cancer survivors has been increasingly recognized and occurs at a prevalence ranging from 15 to 50% [226–235].
Etiology and Mechanisms
Given the complexity of potential causes, fatigue is considered multifactorial in origin in most cancer patients (Table 12.4). For instance, it is well known that specific comorbidities, such as anemia, liver, or renal disease, cause fatigue [236]. In addition, many medications, mood-related issues, pain, sleep dysfunction, lifestyle patterns, cognitive deficits, and psychological stressors are associated with fatigue [237–239].
Table 12.4
Possible factors to cause or contribute to fatigue in cancer patients
Cancer and cancer therapy related factors | ∙ Tumor and brain area involved |
∙ Surgery | |
∙ Radiation therapy | |
∙ Chemotherapy | |
∙ Biologics and molecular-targeted therapies | |
∙ Hormonal therapy | |
Comorbidities and cancer associated conditions | ∙ Sleep dysregulation |
∙ Mood disorder | |
∙ Anxiety | |
∙ Pain | |
∙ Electrolyte imbalance | |
∙ Liver dysfunction | |
∙ Renal disease | |
∙ Thyroid dysfunction | |
∙ Diabetes mellitus | |
∙ Cardiopulmonary disease | |
∙ Anemia | |
∙ Infections | |
∙ Rheumatological disease | |
∙ Neurological disease | |
Psychosocial factors | ∙ Personal and emotional stressors |
Medications | ∙ Antiepileptic drugs |
∙ Opiates | |
∙ Anxiolytics | |
∙ Cardiac medications | |
∙ Hypnotics | |
∙ Analgesics | |
Constitutional factors | ∙ Behavioral factors (eating behavior, lack of exercise, alcohol and other substance abuse) |
∙ Obesity or anorexia |
This issue is particularly relevant in the management of brain tumor patients, in whom mood dysfunction, cognitive impairment, sleep alterations, and fatigue appear to coexist and correlate with each other, causing significant impairment of quality of life in affected patients [240].
The pathophysiological mechanisms that induce chronic fatigue remain poorly understood. Possible causal factors include physical conditions, mood and cognitive dysfunction, metabolic and neuroendocrine factors, and an increase in circulatory inflammatory cytokines [241]. A chronic inflammatory state has been recognized as one major etiological factor in the manifestation of chronic fatigue during cancer treatment or in the post-treatment phase [220]. Specifically, TNF-α signaling has been identified as a likely contributor to chemotherapy-induced fatigue in breast cancer patients [238]. In addition, alterations of sympathetic and parasympathetic nervous system activity have been identified as a possible cause of cancer-related fatigue [242, 243]. However, given a considerable variability of fatigue in cancer patients, various other factors unrelated to treatment, or the underlying disease, appear to influence the degree of fatigue in individual patients. Modulators of fatigue include genetic factors [241], metabolic, behavioral, and lifestyle factors [244, 245], mood-related issues [231], or psychological factors [232, 246] (Table 12.4).
Patient Evaluation
Every patient managed for cancer during the active treatment or post-treatment phase should be evaluated for symptoms of fatigue [247]. Several questionnaires are used and have been validated to assess symptoms and severity of fatigue in cancer patients [237, 248–250]. With the increasing awareness of the high prevalence of fatigue among cancer patients, evaluation and management of fatigue has become an important aspect of supportive care in oncology and survivorship. Several guidelines have been published from expert groups, including the American Society of Clinical Oncology and the National Comprehensive Cancer Network, recommending screening for fatigue at the initial visit, during active treatment, and in the post-treatment setting [251, 252]. A multidisciplinary team approach is necessary in most patients to rule out and treat conditions that might be unrelated to cancer but possibly to treatment, such as endocrine dysfunction (e.g., thyroid disease), medical comorbidities (such as anemia), untreated depression, or sleep disorders [206].
Treatment and Management
Any comorbidity or treatable condition possibly contributing to the manifestation of fatigue will need to be addressed and treated as the initial step in patient management (Table 12.4). For instance, correction of an underlying anemia or thyroid dysfunction [253] and treatment of depression or sleep disorder [254] need to be integral part of patient management.
A variety of therapeutic interventions, evaluated in randomized controlled clinical trials, have resulted in improvement of chronic fatigue in cancer patients. Treatment interventions shown to have clinical benefit include pharmacological interventions (e.g., psychostimulants such as methylphenidate and modafinil) [161, 255], and non-pharmacological interventions [252, 256], such as relaxation techniques [257–259], acupuncture [260], meditation [172, 261, 262], lifestyle interventions [263–266], and physical exercise [217, 252, 267–271]. In addition, strategies aiming at a reduction of psychosocial stressors may involve lifestyle modifications and can improve symptoms of fatigue [252, 272–275].
Collectively, management of fatigue requires identification and treatment of comorbidities and several conditions known to cause fatigue [276]. The complexity of potential etiological factors in cancer-related fatigue underscores the importance of an individualized and multi-disciplinary team approach in patient management. Both pharmacological and non-pharmacological interventions have shown promise to improve fatigue-related symptoms and increase quality of life in cancer patients.
Acknowledgements
J.D. has been supported by a Paul Calabresi Career Development Award for Clinical Oncology, the Stephen E. and Catherine Pappas Cancer Research Foundation Award, the American Academy of Neurology Foundation, the American Cancer Society, and the National Institute of Health. JD received generous philanthropic support from the Sheila McPhee and Ronald Tawil family foundations.
References
1.
Wefel JS, Kayl AE, Meyers CA. Neuropsychological dysfunction associated with cancer and cancer therapies: a conceptual review of an emerging target. Br J Cancer. 2004;90(9):1691–6.PubMedPubMedCentral
2.
Wefel JS, Witgert ME, Meyers CA. Neuropsychological sequelae of non-central nervous system cancer and cancer therapy. Neuropsychol Rev. 2008;18(2):121–31.PubMed
3.
Noll KR, Sullaway C, Ziu M, Weinberg JS, Wefel JS. Relationships between tumor grade and neurocognitive functioning in patients with glioma of the left temporal lobe prior to surgical resection. Neuro Oncol. 2015;17(4):580–7.PubMed
4.
Wu AS, Witgert ME, Lang FF, Xiao L, Bekele BN, Meyers CA, et al. Neurocognitive function before and after surgery for insular gliomas. J Neurosurg. 2011;115(6):1115–25.PubMed
5.
Heimans JJ, Reijneveld JC. Factors affecting the cerebral network in brain tumor patients. J Neurooncol. 2012;108(2):231–7.PubMedPubMedCentral
6.
Derks J, Reijneveld JC, Douw L. Neural network alterations underlie cognitive deficits in brain tumor patients. Curr Opin Oncol. 2014;26(6):627–33.PubMed
7.
Shields LB, Choucair AK. Management of low-grade gliomas: a review of patient-perceived quality of life and neurocognitive outcome. World Neurosurg. 2014;82(1–2):e299–309.PubMed
8.
Guerrini R, Rosati A, Giordano F, Genitori L, Barba C. The medical and surgical treatment of tumoral seizures: current and future perspectives. Epilepsia. 2013;54(Suppl 9):84–90.PubMed
9.
van Breemen MS, Wilms EB, Vecht CJ. Epilepsy in patients with brain tumours: epidemiology, mechanisms, and management. Lancet Neurol. 2007;6(5):421–30.PubMed
10.
Bosma I, Vos MJ, Heimans JJ, Taphoorn MJ, Aaronson NK, Postma TJ, et al. The course of neurocognitive functioning in high-grade glioma patients. Neuro Oncol. 2007;9(1):53–62.PubMedPubMedCentral
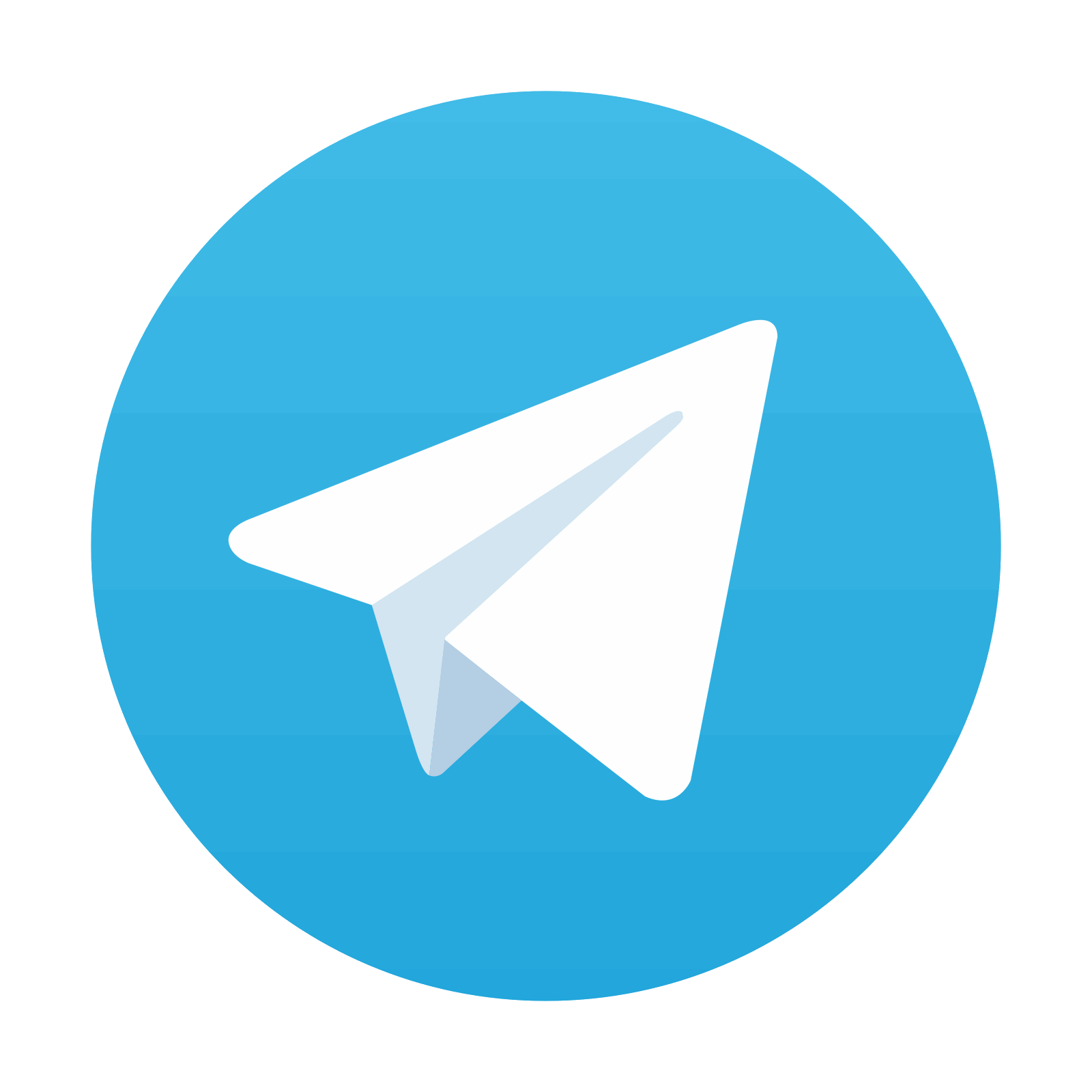
Stay updated, free articles. Join our Telegram channel

Full access? Get Clinical Tree
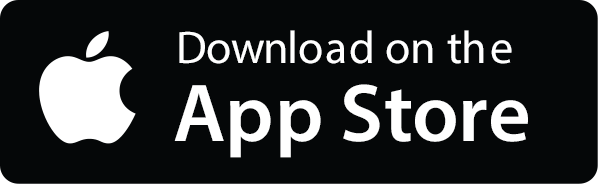
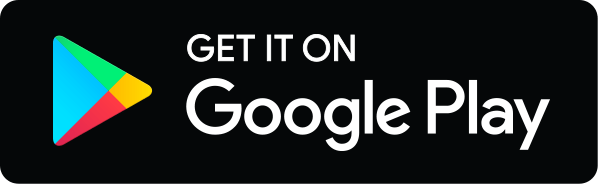