Acid–base and electrolyte disorders are nearly ubiquitous in hospitalized patients and often originate from or manifest as neurological illness. Prompt recognition of the presence and degree of acid–base disorders through analysis of arterial blood gas can have a significant clinical impact in terms of diagnosis and treatment, particularly in the setting of increased intracranial pressure, respiratory muscle weakness, and seizures. Sodium abnormalities, such as diabetes insipidus, the syndrome of inappropriate antidiuretic hormone secretion, and cerebral salt wasting, are often encountered in neurological patients in association with their primary illness. Correction of hyponatremia and hypernatremia should be gradual to avoid central pontinemyelinolysis and cerebral edema. Abnormalities of potassium, calcium, and magnesium are also common and can cause serious neurologic and systemic abnormalities that warrant prompt recognition and correction.
The maintenance of acid–base homeostasis is essential for life and is strictly regulated by the body. Disruptions in the system outside of the physiologic range can have widespread and deleterious effects on human physiology, as acid–base status plays an integral role in protein and membrane functioning.1,2 Prompt and correct interpretation of acid–base derangements can be as challenging as clinically impactful.
The carbonic acid–bicarbonate buffer system is the main physiological determinant of acid–base status in the body and is represented by the following reaction:3
This depicts the bidirectional conversion of carbon dioxide and water to form carbonic acid, which rapidly dissociates to form bicarbonate and hydrogen ions. In vivo, the three main variables that can be altered are the partial pressure of CO2 (PaCO2), bicarbonate, and hydrogen ions. Changes in PaCO2 and/or bicarbonate ultimately affect the amount of hydrogen ions by driving the equation to the left or right. The pH, or negative log of the hydrogen-ion concentration, quantifies the acid–base status in a particular individual. The terms acidemia and alkalemia refer to abnormally low and high pH, respectively. Alterations in pH can be categorized according to the primary etiology—“respiratory” (characterized by changes in PaCO2 from alteration in minute ventilation) or “metabolic” (characterized by changes in bicarbonate) forming the four primary conditions affecting pH: respiratory acidosis, respiratory alkalosis, metabolic acidosis, and metabolic alkalosis. While clinical context can be extremely helpful in determining a patient’s acid–base status, the arterial blood gas (ABG) provides crucial information about the severity, etiology, and chronicity of acid–base abnormalities.
An ABG should be obtained whenever there is a suspicion of acid–base disturbance based on clinical context and symptomatology to confirm the presence and ascertain the type and degree of the acid–base disorder. The pH can be used first to delineate whether the patient has acidemia (pH <7.35) or alkalemia (pH >7.45). Next, the PaCO2 and bicarbonate levels should be integrated to determine the primary mechanism of the acid–base disturbance, to ascertain the presence and degree of compensation, and to establish if a mixed disturbance is present.
The primary mechanism of the acid–base disorder is usually denoted by the most abnormal value (PaCO2 or bicarbonate) congruent with the patient’s clinical picture. Compensation is a natural mechanism to maintain homeostasis. The compensatory mechanism is opposite to the primary disturbance, that is, metabolic compensation occurs for a primary respiratory disturbance and vice versa. Compensation is always in the same direction as the primary disturbance, that is, if an elevation in PaCO2 occurs as part of a respiratory acidosis, compensation occurs via an increase in bicarbonate in an attempt to maintain normal pH. A simple acid–base disorder is defined as a primary disturbance and its compensatory response. In contrast, a complex acid–base disorder consists of multiple primary disturbances. A metabolic acidosis can coexist with a metabolic alkalosis; however, only one respiratory disturbance is possible.3 Respiratory compensation for a metabolic disturbance is relatively rapid and can occur for hours. Conversely, metabolic compensation for a respiratory disturbance occurs more slowly and the degree of change in bicarbonate increases with chronicity. The identification of multiple metabolic disturbances can be identified with knowledge of the expected direction and magnitude of compensation. If the measured compensation is not equal to the calculated compensation, a mixed disorder is present. Table 12-12–4 provides definitions of the four types of primary acid–base disturbances and direction, magnitude, and timing of expected compensation.
Venous blood gases (VBG) are increasingly used as an estimate of systemic pH, PaO2, PaCO2, and bicarbonate due to patient comfort and their ease of acquisition in patients without arterial lines and in those where obtaining an arterial sample is difficult. They may also be obtained inadvertently from a presumed arterial stick. Due to tissue extraction of oxygen and expulsion of cellular metabolic waste products, VBGs generally have a lower PaO2, pH, and PaCO2 than their arterial counterparts. However, when compared with ABGs only the pH can be reliably estimated and is typically decreased by 0.03. Confidence intervals in comparing ABG and VBG values for PaO2, PaCO2 and bicarbonate are large and prohibit accurate estimation.5,6
One final feature of the ABG that is indirectly related to acid–base status is the PaO2. Alterations in PaO2 can be used to assist in determining the cause of a primary respiratory acid–base disorder, as hypoxemia may precipitate a respiratory alkalosis and a lactic acidosis. Furthermore, analysis of the PaO2 relative to ventilation can be estimated by calculating the difference between oxygen tension in the alveoli and arterial blood (known as alveolar–arterial oxygen gradient) and this estimate can help differentiate extrapulmonary from intrapulmonary causes of respiratory acid–base disturbances. The alveolar–arterial (A-a) gradient is estimated as follows:
where the barometric pressure is 760 mm Hg at sea level, the water vapor pressure is 47 mm Hg at 37°C, and the gas exchange ratio is approximately 0.8. Unless marked differences are present in the aforementioned physiologic parameters, the equation can be simplified to:
There is a normal difference in the A-a gradient in all individuals that increases with age because of physiologic ventilation/perfusion mismatch. The normal A-a gradient is 5–10 mm Hg and 15–20 mm Hg in the young and elderly, respectively. Increases beyond the normal values can indicate intrapulmonary pathology.7
The next section will further discuss more specific laboratory characteristics, symptoms, and etiologies of the four primary acid–base disorders.
Respiratory acidosis is defined as the presence of acidemia secondary to elevation in PaCO2 greater than 45mmHg. After establishing the presence of a respiratory acidosis, the next step is to ascertain its chronicity though analysis of the degree of metabolic compensation and clinical history. Compensation should occur through the elevation of bicarbonate: an increase in 1 mmol/L per 10 mmHg increase in the PaCO2 implicates an acute respiratory acidosis, while an increase of 4 to 5 mmol/L per 10 mmHg increase in PaCO2 implicates a chronic respiratory acidosis. A change in the bicarbonate level of less than 1 mmol/L or greater than 5 mmol/L per 10 mmHg increase in PaCO2 heralds the presence of a coexisting metabolic acidosis or alkalosis, respectively. Metabolic compensation occurs in less than 6 hours and between 2 and 5 days for acute and chronic conditions, respectively2,3 (Table 12-1).
Characteristics of Simple Acid–Base Disorders and Predicted Compensation
Disorder | Primary Acid–base Abnormality | Mode and Direction of Compensation | Magnitude of Compensation | Time to Completion | Mixed Disorder |
---|---|---|---|---|---|
Acute respiratory acidosis | PaCO2> 45 | ↑ HCO3 − | 1 mmol/L per 10 mm Hg increase in PaCO2 | <6 hours | Measured HCO3 − is < or > calculated |
Chronic respiratory acidosis | PaCO2> 45 | ↑ HCO3 − | 4–5 mmol/L per 10 mmHg increase in PaCO2 | 2–5 days | Measured HCO3 − is < or > calculated |
Acute respiratory alkalosis | PaCO2< 45 | ↓ HCO3 − | 2 mmol/L per 10 mmHg decrease in PaCO2 | <6 hours | Measured HCO3 − is < or > calculated |
Chronic respiratory alkalosis | PaCO2< 45 | ↓ HCO3 − | 4–5 mmol/L per 10 mmHg decrease in PaCO2 | 2–5 days | Measured HCO3 − is < or > calculated |
Metabolic acidosis | HCO3< 24 | ↓ CO2 | 1.5 × HCO3 − +8 | 12–24 hours | Measured PaCO2 is < or > calculated |
Metabolic alkalosis | HCO3>24 | ↑ CO2 | 0.9 × HCO3 −+9 | 24–36 hours | Measured PaCO2 is < or > calculated |
The physical manifestations of respiratory acidosis are primarily the result of the associated acidemia and manifest principally within the neurologic and cardiovascular organ systems, with the exception of hyperkalemia produced from associated extracellular extrusion. Patients are often asymptomatic until the pH drops below 7.2. CO2 diffuses rapidly across cellular membranes, including the blood–brain barrier, and therefore physical manifestations can occur in rapid succession with rises and falls in PaCO2. The presence and degree of symptoms are directly correlated with the magnitude and rapidity of change in PaCO2, and patients with compensated chronic respiratory acidosis pose the least risk of becoming symptomatic.8
Neurological symptoms and signs are seen more frequently in cases of respiratory acidosis than in cases of metabolic acidosis due to the ease at which CO2 diffuses across the blood–brain barrier. They include irritability, agitation, anxiety, hallucinations, cognitive dysfunction, confusion, and anorexia. However, progression to include delirium, somnolence, lethargy, and frank coma can occur, the latter typically being associated with PaCO2 levels exceeding 70–100 mmHg. On neurological examination, asterixis, myoclonus, tremor, and hyperreflexia progressing to hyporeflexia can be seen.8–10 Importantly, levels of pCO2 greater than 50 mmHg can produce cerebral vasodilatation and an increase in cerebral blood flow, resulting in a rise in intracranial pressure (ICP).11 Symptoms secondary to increased ICP include nocturnal or early morning headache, confusion, progressive obtundation, and visual disturbances ranging from blurred vision to blindness. Neurological signs of increased ICP include papilledema, optic atrophy, elevation of opening cerebrospinal fluid (CSF) pressure, or, in most severe cases, signs indicative of impending herniation syndromes, such as pupillary changes or upper motor neuron findings.12 The importance of avoiding respiratory acidosis in the patient with intracranial pathology placing them at risk for elevated ICP cannot be overstated, and careful clinical and laboratory monitoring is essential to avoid exacerbation of ICP from hypercapnia. In addition to confounding the clinical examination through sedation, opioid medications should be avoided in these patients because associated depression in the respiratory drive may provoke hypercapnia.
Cardiovascular manifestations begin with beta-adrenergic stimulation from catecholamine release associated with mild-to-moderate hypercapnia. However, with larger increases in PaCO2 inhibition of myocardial contractility, systemic vasodilation, and cardiovascular collapse can occur. Specifically, as the pH drops below 7.20 progressive attenuation of catecholamine effect occurs, limiting the efficacy of pressors. Flushing and cardiac arrhythmias may also occur.8,9 Respiratory acidosis should be considered in the differential diagnosis for PEA arrest.
Respiratory acidosis can be largely stratified as originating from central nervous system (CNS) or neuromuscular hypoventilation versus cardiopulmonary disease based on whether an A-a gradient is present on the ABG. Causes of respiratory acidosis are summarized in Table 12-2.2,8,10 In addition to the lethargic or comatose patient on opioids or with a concern for increased ICP, the neurologist should be particularly keen to look for the presence of a respiratory acidosis in patients with neuromuscular respiratory failure (as this may signal the need for intubation) and in seizure patients.
Common Medical Conditions Causing Respiratory Acidosis Characterized by Chronicity, A-a Gradient, and Localization
Acute Respiratory Acidosis | Chronic Respiratory Acidosis | ||
---|---|---|---|
With Normal A-a Gradient | With Abnormal A-a Gradient | With Normal A-a Gradient | With Abnormal A-a Gradient |
|
|
|
|
|
|
|
|
|
|
|
|
|
Treatment should focus on addressing the underlying cause for the respiratory acidosis and maximizing minute ventilation through jaw thrust maneuvers, nasal or oral airway, bag mask ventilation, noninvasive measures (eg, positive airway pressure devices), or endotracheal intubation depending on the clinical situation and the level of consciousness. Infusion of bicarbonate can be used as a secondary measure in emergency situations (such as during cardiopulmonary resuscitation) or in cases of complex refractory hypercarbia to assist in normalizing the pH. Symptoms and signs of respiratory acidosis typically improve quickly and in conjunction with correction of the hypercarbia. However, the decrease in PaCO2 should be gradual to prevent overcorrection in the case of metabolic compensation, as the CO2 will be eliminated more rapidly than the bicarbonate. The resulting metabolic alkalosis from overcorrection may be complicated by seizures.13
Respiratory alkalosis is the most common acid–base disorder, normally occurring in pregnant individuals and persons living at high altitude. It is defined as the presence of alkalemia secondary to a decline in PaCO2 to less than 35 mmHg. Similar to respiratory acidosis, after establishing the presence of a respiratory alkalosis, the next step is to determine its chronicity though clinical history and degree of metabolic compensation. Contrary to the case of respiratory acidosis, metabolic compensation occurs with a decrease in bicarbonate. A decrease of 2 mmol/L per 10 mmHg decrease in the PaCO2 occurs in an acute respiratory acidosis, while a decrease of 4 to 5 mmol/L per 10 mmHg decrease in PaCO2 points to a chronic respiratory acidosis. A change in the bicarbonate level of less than 2 mmol/L or greater than 5 mmol/L per 10 mmHg decrease in PaCO2 implies the presence of a coexisting metabolic alkalosis or acidosis, respectively. Metabolic compensation occurs in less than 6 hours and between 2 and 5 days for acute and chronic conditions, respectively2 (Table 12-1).
Symptoms associated with respiratory alkalosis most commonly include lightheadedness, perioral and limb paresthesias, headache, muscle cramps, and vertigo, but patients less commonly complain of tremor, tinnitus, blurred vision, ataxia, confusion, depression in level of alertness, chest pain, cardiac arrhythmias, seizure, or syncope.14,15 The presence and degree of symptoms correlate with the degree of hypocapnia, and patients with chronic respiratory alkalosis usually are asymptomatic. The mechanism behind the emergence of symptoms is alkalemia and, perhaps more importantly, the accompanying vasoconstriction. Purposeful hyperventilation to produce cerebral vasoconstriction can be used to decrease cerebral blood flow (CBF) and blood volume, thus reducing ICP by allowing additional space inside the skull in accordance with the Monro-Kellie doctrine. The response is rapid and significant (CBF decreases 40% approximately 30 minutes after reducing the PaCO2 by 20 mm Hg), but is not sustained—rapid buffering of bicarbonate in the CSF is responsible for the attenuated effect of hypocarbia on CBF after several hours.16 Furthermore, while transient hyperventilation can be an extremely useful method to reduce acute elevations in ICP, prolonged hypocarbia provides no benefit and a decrease in respiratory rate to target normocarbia after compensation is established can cause cerebral vasodilation and rebound increases in ICP with the resultant metabolic acidosis.17 The reduction in CBF with hyperventilation produces relative ischemia, and in susceptible individuals, can result in seizures. Likewise, the vasoconstriction associated with hypocarbia can also affect the coronary circulation and result in chest pain in patients with pre-existing coronary stenosis. Finally, hypokalemia, hypophosphatemia, and hypocalcemia can all occur as the result of the alkalemia from intracellular translocation and renal losses.15 With the exception of certain specific disorders such as inappropriate ventilator settings, some psychiatric conditions, and central neurogenic hyperventilation, the blood pH rarely exceeds 7.55, and therefore symptoms and signs tend to be less prominent than with metabolic alkalosis.14
Purposeful or accidental hyperventilation is the most common reason for respiratory alkalosis, although hyperventilation may be a harbinger of serious underlying pathology. Many patients with severe pain or significant brain injury may have increased respiratory drive. Additionally, patients with brainstem injury may develop central neurogenic hyperventilation, characterized by inability to breath-hold.18 Drug toxicities, including aspirin, cocaine, and amphetamines, should also be considered in the comatose patient without iatrogenic cause for respiratory alkalosis. Suspicion should be high for pulmonary embolus if an A-a gradient is present. A more complete list of potential causes of respiratory alkalosis is summarized in Table 12-3.2,14
Causes of Respiratory Alkalosis
Normal A-a Gradient | Abnormal A-a Gradient |
---|---|
Induced hyperventilation | Compensatory response to hypoxemia |
Postoperative pain | Pulmonary embolus |
Anxiety/Panic attack | Adult respiratory distress syndrome/Acute lung injury |
Early sepsis | Cyanotic heart disease |
Fever | Pneumothorax |
Central neurogenic hyperventilation | Hemothorax |
Intracranial mass | Pneumonia |
Pregnancy | Pulmonary edema |
Hyperthyroidism | Interstitial lung disease |
High altitude | |
Severe anemia | |
Mechanical ventilation | |
Drug overdose (stimulants, aspirin) |
Treatment should be focused on management of the underlying cause. If the patient is not on mechanical ventilation, intubation should be considered depending on the cause and severity of the respiratory alkalosis and the degree of fatigue. Mechanically ventilated patients can be sedated and/or tidal volume and respiratory rate can be reduced.19
Metabolic acidosis is defined as acidemia from depletion of serum bicarbonate to a concentration lower than 22 mmol/L either through bicarbonate elimination from the body or through reaction with excess hydrogen ions. Respiratory compensation through elimination of CO2 occurs relatively quickly (12–24 hours to completion) and can be predicted by using Winter’s formula (see Table 12-1):
If the observed PaCO2 on the ABG is lower than the expected PaCO2 calculated using Winter’s formula, then a superimposed respiratory alkalosis is present. Conversely, if the observed PaCO2 is higher than the expected PaCO2, then a superimposed respiratory acidosis is present.2
Understanding the concept of the anion gap is instrumental in the workup of a metabolic acidosis. The sum of all positive and negative ions must be equal in the body. The three ions with the highest concentrations and greatest contribution are sodium [Na+], chloride [Cl−], and bicarbonate [HCO3 −]. Numerous other ions (eg, magnesium, potassium, phosphorus, etc.) contribute to the net positive and negative charges, but their influence is essentially negligible, with the exception of albumin. The anion gap is defined as the difference between the net positive charges and the net negative charges:
The number of sodium ions in the serum always outnumbers the sum of the chloride and bicarbonate ions; as the body must maintain electroneutrality, the anion gap represents unmeasured anions in the serum that maintain the electrical homeostasis (Figure 12-1).3,10 Under physiologic conditions, much of the normal anion gap is accounted for by albumin, and therefore for every decrease of 1 g/dL in serum albumin, the anion gap should be increased by 2.5 mmol/L.20 Normal ranges for the anion gap vary among laboratories but the mostly commonly used normal values are 10–12 mmol/L.21
Figure 12-1
Normal Anion Gap and Anion Gap Metabolic Acidosis. The concept of the normal anion gap is illustrated in the figure to the left. Sodium essentially constitutes the entirety of positive charges present in the body. Chloride and bicarbonate largely represent the body’s negative charges with the anion gap, a conglomeration of albumin and other negatively charged ions contributing the remainder of negative charges necessary for electroneutrality. The addition of lactate, or other acid, reduces the bicarbonate concentration and increases the anion gap.

An anion gap metabolic acidosis represents the addition of nonvolatile acid to the serum. Dissociation of the acid produces a hydrogen ion, which binds to and neutralizes bicarbonate, and its respective anion, which contributes to the anion gap (Figure 12-1). A nonanion gap metabolic acidosis represents pure loss of bicarbonate from the serum (Figure 12-2).3,10 Therefore, in the context of the clinical history, the knowledge of the type of metabolic acidosis is invaluable in elucidating the cause and pathophysiology of the acid–base disorder. Table 12-4 lists frequently suspected causes of anion gap and nonanion gap acidosis.2,10 Additionally, the mnemonic “GOLD MARRK,” representing glycols (ethylene and propylene), 5-oxoproline (pyroglutamic acid), L-lactate, D-lactate, methanol, aspirin, renal failure, rhabdomyolysis, and ketoacidosis, is a helpful way to remember causes of anion gap metabolic acidosis.22 The neurologist will most commonly encounter an anion gap metabolic acidosis in patients with convulsive seizures, patients with lactic acidosis due to sepsis, and patients with diabetic ketoacidosis or renal failure. Rhabdomyolysis may also cause an anion gap metabolic acidosis in patients with prolonged convulsive status epilepticus, or in patients with ischemic stroke or intracerebral hemorrhage who are found hours after the event lying on their paralyzed limb(s). Finally, in a patient with unexplained altered mental status or coma with a high anion gap metabolic acidosis one should suspect ingestion of a toxic alcohol. Propylene glycol intoxication can also occur iatrogenically from high-dose lorazepam infusion. An elevated osmolar gap can signal the correct diagnosis in these situations, as all these toxins can present with an elevated osmolar gap (>10 mOsm/kg).23 In the case of hyperacute poisoning, the osmolar gap may be elevated prior to the anion gap. The osmolar gap is defined as:
Common Causes of Anion Gap and Non-Anion Gap Metabolic Acidosis
Anion Gap Metabolic Acidosis | Non-Anion Gap Metabolic Acidosis |
---|---|
|
|
|
|
|
|
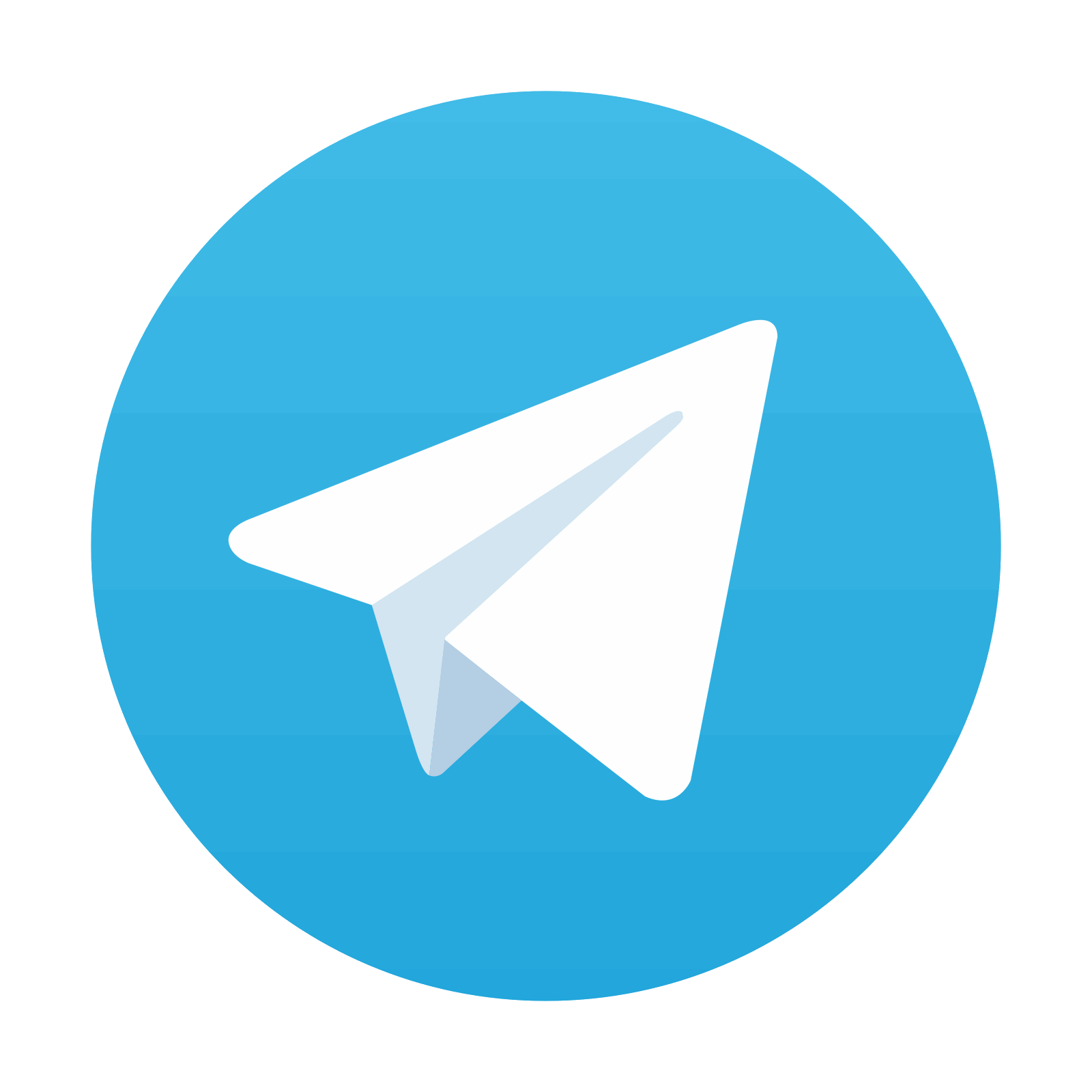
Stay updated, free articles. Join our Telegram channel

Full access? Get Clinical Tree
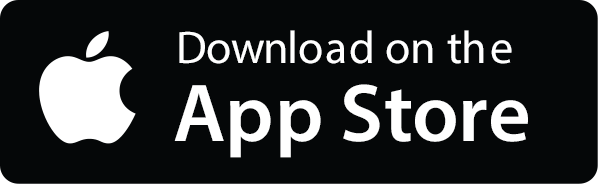
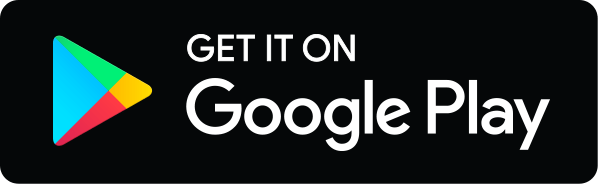
