Tumors of the nervous system are the group of neoplasms that arise from or metastasize to brain, spinal cord, meninges, or nerves. They include a wide variety of histological types and grades of malignancy as well as genetic heterogeneity. This creates a diagnostic complexity that requires a combined approach to incorporate imaging, histopathology, and genomic methods. Treatment is based on morphologic and, increasingly, molecular data, and often involves a multidisciplinary approach with surgery, irradiation, and chemotherapy as well as targeted therapies. In spite of the recent advances in diagnosis and treatment, prognosis of the most common primary neoplasms—high-grade gliomas—remains poor.
Tumors of the nervous system define a large group of neoplasms that arise from the different cell types constituting the central nervous system (CNS; neuroepithelial tumors, pituitary tumors), its covering (meningeal tumors), the peripheral nervous system (nerve sheath tumors), lymphatic or hematopoietic cells, and developmental remnants (germ cell tumors). These primary tumors are outnumbered by nervous system metastases. Tumors are classified by location (supratentorial, infratentorial, and spinal), cell of origin (astrocytoma, oligodendroglioma, ependymoma, etc), pathological behavior (high grade, low grade), relation to neural tissue (intra-axial, extra-axial, intradural–extramedullary, intramedullary, etc), and radiological (contrast enhancing, cystic, etc). A combination of classification methods can be used in a comprehensive way of analyzing a CNS tumor and making a decision on how to manage them.
Throughout this chapter, we will be mainly using the World Health Organization (WHO) pathological classification system, which is primarily based on histopathologic appearance, cell types of origin, and growth patterns, but also with constant references to other methods to achieve this comprehensive approach. The WHO grading system by incorporating cell density, infiltrative capacity, number of mitoses, vascular proliferation, and necrosis, creates a scale from I to IV by which the degree of malignancy can be quantified and behavior and therapeutic response can be predicted.1
CASE 44-1
A 69-year-old woman presents with a generalized tonicclonic seizure on a background of significant vascular risk factors and no known epilepsy history. The patient arrives in the emergency department (ED) and is placed in a resuscitation bay. She has one further episode of seizure. An urgent EEG shows generalized suppression as would be expected postictally but also some sharp wave activity in temporal lead derivations. An urgent CT with contrast is performed, which shows a large enhancing lesion in the parieto-temporal junction. She is loaded with phenytoin while waiting for a neurology bed.
Her visibly distressed daughter approaches you at the computer. She has been told that her mother has a tumor in her head. She wants more information regarding what options are available. You explain that after her stabilization she will be transferred to a tertiary referral center with neurosurgical service where therapeutic decision will be made, but you indicate your willingness to give her some general information.
Surgery
Radiation
Chemotherapy
Other more novel modalities
For most patients with brain tumors, surgery is the primary treatment modality. It serves a multitude of purposes:
provides diagnostic information
alleviates mass effect
reduces seizure activity
may offer cure
Tumor resection is preferred, but when not feasible, a stereotactic biopsy is performed. A stereotactic frame is placed on the patient’s head assuring appropriate sampling. Functional MRI and diffusion-tensor imaging define the relationship between tumor and contiguous, eloquent areas of the brain, and white matter tracts. These data are used to choose a target and trajectory, minimizing morbidity and maximizing diagnostic yield. The probe is then passed through a small drill hole. Cylindrical samples 1 cm in length and 1–2 mm in diameter are retrieved. The procedure is safe and associated with less than a 2% risk of seizure, hemorrhage, or infection. The most relevant shortcoming of the procedure is sampling error, that is, acquisition of tissue that may not be representative of the tumor in its entirety or not diagnostic at all.
Complete surgical resection is curative for noninfiltrative gliomas, pituitary adenoma, or meningioma. Less clear is the benefit of resecting infiltrating neuroepithelial neoplasms. Widely accepted indications are complete resection of astrocytomas and oligodendrogliomas, debulking of large masses causing neurologic dysfunction by compression or increased intracranial pressure, intratumoral hemorrhage giving rise to sudden neurologic decline, or uncontrollable seizures. Intraoperative tools aiding tumor resection include MRI, cortical stimulation mapping, and monitoring of somatosensory evoked potentials. Postoperative MRI should be obtained within 48 hours whenever possible in order to document the extent of resection before adjuvant therapies are initiated.
A Food and Drug Administration (FDA)-approved device for intraoperative MRI-guided laser interstitial thermocoagulation therapy (LITT) can be used for the treatment of brain metastases and primary brain tumors when conventional treatment strategies have been exhausted.2
Radiation therapy (RT) is commonly used to consolidate the success of tumor resection or to address the infiltrative nature of cerebral neoplasms. RT may be curative for germinomas and substantially prolongs the survival of patients afflicted with a variety of glial and nonglial tumors. In addition, RT provides symptomatic relief (headaches, seizures).
Radiation causes breaks in the DNA double strand either by ionization of the target atom or by production of free radicals. The effect of radiation depends on the dose applied, frequency of administration, time intervals between exposures (ie, time available for damage repair), and phase of the cell cycle the exposed cell is in (most susceptible in M- and G2 phases). Photons are the most commonly used particles for brain tumor therapy. Examples of nonphoton irradiation modalities include neutrons, protons, and heavy ions (carbon, argon, neon).
Radiation therapy is usually delivered by a linear accelerator (LINAC), which uses high-frequency electromagnetic waves to accelerate electrons to high energies. For superficial tumors, the electron beam is used directly. Deep-seated lesions are treated indirectly with x-rays produced by the electron beam. Individually fit shielding blocks restrict the beam to the target volume. For infiltrative tumors, radiation is prescribed to the volume of enhancement or T2 abnormality on MRI and a safety margin of 1–3 cm. For noninfiltrative lesions, the margin is narrower. Whole-brain radiation therapy (WBRT) is used for extensive gliomatosis or chemorefractory CNS lymphoma.
What are some of the strategies used to maximize cell killing while limiting exposure of skin and normal brain tissue?
Conventional 3D-conformal radiation therapy is provided in daily fractions of 1.8–2.0Gy. The total dose depends on tumor grade (60 Gy for glioblastoma). Fractionation strategies require immobilization devices such as bite blocks and thermoplast molds that allow reproducible positioning of the patient with each treatment. The use of multiple radiation fields or threedimensional conformal irradiation limits the exposure of overlying skin and normal brain tissue.
In brachytherapy, radiation is delivered by implanting the irradiation source close to or into the target tissue. This technique is rarely used for CNS tumors nowadays. A balloon catheter through which radionuclide can be administered is available for temporary intracavitary placement.
Multiple agents or strategies have been used in clinical trials in an attempt to increase radiation-induced cell killing. These include nitroimidazoles, hypoxic cytotoxins, hyperbaric oxygen, agents that alter the hemoglobin-oxygen dissociation curve, and nonhypoxic radiosensitizers. Unfortunately, none of these radiosensitizers have shown to be of any benefit to brain tumor patients.
Radiosurgery delivers large doses of radiation to well-circumscribed tumor sites while minimizing exposure to normal tissue in a single (stereotactic radiosurgery [SRS]) or multiple fractions (stereotactic radiation therapy [SRT]). Various devices are available. A modified linear accelerator and gamma knife use photon beams. Gamma knife provides irradiation using 200 separate and collimated cobalt-60 sources in a hemispherical array aimed at the target. Proton beam radiosurgery offer optimal physical characteristics for stereotactic applications as it penetrates to relatively finite depths beam reflecting the energy imparted to the particle (Bragg peak). These radiosurgery techniques require a more robust fixation of the patient’s head in space than conventional radiation (rigid frames affixed to the patient’s skull or fitted mouthpieces). New devices designed for robotic frameless SRS are now available (Cyberknife, NovalisTx). Target localization is based on an image-to-image correlation algorithm utilizing plain radiographs.
Chemotherapy is provided to most patients with malignant brain tumors at some point in their disease course. For some tumors such as primary CNS lymphoma, it is the primary treatment modality, while for other neoplasms, it is used in an adjuvant fashion.
Alkylating agents (temozolomide, lomustine, carmustine, procarbazine, cyclophosphamide) are the most commonly used class of chemotherapy drugs against brain tumors. They covalently bind alkyl groups to DNA resulting in the formation of intra- and interstrand crosslinks interfering with transcription. Mechanisms of tumor resistance include decreased drug uptake and elimination of alkylated nucleosides by the repair enzyme O6-alkylguanine alkyltransferase (AGAT or MGMT). The most common toxicities encountered with these agents are myelosuppression, nausea, and infertility. Secondary malignancies occur in up to 5–10% of oncology patients with a peak incidence 5–7 years after exposure but this is a rare complication in the brain tumor population.
Antifolates are inhibitors of tetrahydrofolate synthesis, a key component in pyrimidine and purine metabolism. The dihydrofolate reductase (DHFR) antagonist methotrexate has to be given intravenously in gram-equivalent doses in order to achieve therapeutic concentrations within the nervous system and the eye. This requires alkaline diuresis, avoidance of concomitant use of drugs competing for excretion in the proximal tubule, and initiation of leukovorin rescue 24 hours after starting the methotrexate infusion. Pemetrexed inhibits at least 3 enzymes involved in folate metabolism and DNA synthesis: thymidylate synthase, dihydrofolate reductase, and glycinamide ribonucleotide formyltransferase. Both agents are used for the treatment of primary CNS lymphoma.
The deoxycytidine analogue cytosine arabinoside is a competitive inhibitor of DNA polymerase A. It is a “false” nucleoside that, after incorporation into the nascent DNA strand, causes inhibition of chain elongation.
Agents interfering with polymerization and disassembly of microtubules inhibit cell division, intracellular transport, and secretion. These include vinca alkaloids, naturally found in Catharanthus roseus, and taxanes (paclitaxel, docetaxel).
Platinum compounds produce intrastrand adducts linking 2 nucleotides by forming bifunctional bonds to DNA. Recognition of platinum adducts and initiation of apoptosis are dependent on the DNA mismatch repair system.
Topoisomerase inhibitors catalyze the temporary uncoiling and unlinking of the DNA double strand during replication. Camptothecin derivatives (irinotecan, topotecan) inhibit topoisomerase I, an enzyme that introduces single-strand breaks into the DNA molecule. Etoposide and teniposide, semisynthetic derivatives of podophyllotoxin, a substance found in mayapple extracts, antagonize the effects of topoisomerase II (introduction of double strand breaks) by interfering with re-ligation of DNA from the cleavage complex.
Autologous peripheral blood stem cell transplantation after myeloablative chemotherapy is increasingly used for consolidation therapy of primary CNS lymphoma. The technique failed to produce higher response rates in malignant gliomas when compared with conventional adjuvant chemotherapy.3,4
For medications not able to cross the blood–brain barrier, how is the medication delivered to the site of action?—
The blood–brain barrier (BBB) limits access of a variety of chemotherapeutic agents to the brain and CSF. It is formed by the endothelial cell layer of cerebral capillaries sealed by intercellular tight junctions, the vascular basal membrane, and astrocytic foot processes. A variety of delivery strategies have been developed to circumvent the barrier for the medications that do not cross the BBB. Chemotherapeutic agents (methotrexate, thio-TEPA, cytosine-arabinoside) are injected intrathecally for the treatment of leptomeningeal metastases. Hypertonic solutions of osmotic diuretics (25% mannitol or 15% glycerol) are infused intrarterially to produce temporary opening of the BBB. The technology requires general anesthesia and serial cerebral angiographic procedures. Biodegradable dimesized polymer wafers (p-carboxyphenoxy [poly bis] propane and sebacic acid) impregnated with BCNU are available for placement in the neurosurgical resection cavity. The drug is released over 7–10 days and diffuses over a short distance into the wall of the resection cavity.5 Complications include infection, wound healing impairment, brain necrosis, and CSF leak.
A large spectrum of novel treatment strategies are under investigation. Some of these treatments have been approved for systemic cancers but only very few for nervous system neoplasms. Techniques fall into several categories:
to enhance the effect of classical chemotherapeutic agents
to interfere with the tumor’s ability to form new blood vessels
to target specific somatic mutations within tumors
to enable the patient’s immune system to eliminate neoplastic cells
What are some of the ways being explored to enhance the effectiveness of classical chemotherapeutic agents?—
Several methods have been developed to overcome resistance to alkylating agents. O6-benzylguanine is a potent inhibitor of AGAT that has been co-administered with alkylating agents.6 Inhibitors of poly(adenosine diphosphate ribose) polymerase (PARP), cell signaling enzymes implicated in cellular responses to DNA injury, potentiate the effect of various chemotherapeutic agents.
Cancer is a disorder of cell growth control. Mutations or epigenetic alterations in the cancer cell genome affect different components of this complex regulatory network: growth factors, growth factor receptors, intracellular signal transducers, nuclear transcription factors, and cell-cycle control proteins. Various strategies have been developed that interfere with the transcription/translation of the mutated genes or the protein they encode at each level. Modified peptides or peptidomimetics are designed to bind to the active sites of altered proteins such as the tyrosine kinase domain of growth factor receptors. Antisense oligonucleotides hybridize with transcripts of growth control genes and inhibit their translation. Ribozymes degrade transcripts with high specificity. Monoclonal antibodies directly target growth control proteins. Gene therapy is designed to restore the function of mutated cell-cycle control proteins.
Growth factors:
Epidermal growth factor receptor (EGFR), commonly overexpressed or mutated in malignant gliomas, is a logical target. However, monotherapy with agents targeting this receptor (gefitinib, erlotinib, and tyrphostin) have invariably failed.7 Predictors of the rare responses have been identified.8
Inhibition of platelet-derived growth factor receptor (PDGFR) with imatinib has likewise been unsuccessful.
This has led to the development of small-molecule inhibitors with a broader spectrum, “dual pathway” inhibitors, and combination regimens using growth factor receptor- and downstream signal transduction inhibitors.
Intracellular signal transduction:
Intracellular signal transduction can be blocked targeting molecules such as phosphoinositide-3-kinase/protein kinase B (PI3K/Akt), protein kinase C (PKC), Ras, and the mitogen activated protein kinase pathway Raf/MEK/ERK. These agents have been studied as monotherapy and in combination with classical cytotoxic agents or growth factor receptor inhibitors.
An example of successful therapy of a CNS neoplasm by targeting signal transduction is the treatment of subependymal giant cell astrocytoma with inhibitors of mTOR, a downstream constituent of the Pi3K/Akt pathway.9
Proteasome inhibition:
Inhibition of the proteasome, a cellular protein degradation complex that recognizes and degrades polyubiquitinated substrates such as cell-cycle control proteins, induces apoptosis.
Bortezomib, a dipeptidylboronic acid derivative, has proven effective in multiple myeloma but remains investigational for primary brain tumors.
Histone deacetylase:
Histone deacetylases (HDAC) induce hyperacetylation of histones resulting in chromatin relaxation and transcriptional activation. HDAC inhibitors exert antineoplastic activity, likely as a result of activation of differentiation programs, cell-cycle inhibition, and induction of apoptosis in cancer cells.10
Suberoylanilide hydroxamic acid (SAHA) and the fungal tetrapeptide depsipeptide are currently undergoing clinical evaluation in malignant gliomas.
IDH1/2:
One of the most fundamental discoveries of the Cancer Genome Atlas Project has been the identification of early somatic mutations in IDH1 and IDH2, which encode two isoforms of isocitrate dehydrogenase, in infiltrative gliomas.11 Mutant IDH1/2 produce 2-hydroxyglutaric acid, a molecule that alters gene expression and appears to be promoting malignant degeneration by inhibiting histone- and DNA-modifying enzymes.12,13
Inhibition of these mutant enzymes may result in normalization of gene expression and reversal of malignant degeneration.14
Anti-angiogenic mechanisms:
The rapid growth of malignant gliomas and other neoplasms is dependent on sufficient stimulation of new blood vessel formation. This reflects the intricate interplay between promoters including vascular endothelial growth factor (VEGF), basic fibroblast growth factor (bFGF), platelet-derived growth factor (PDGF), transforming growth factor (TGF), and tenascin, as well as endogenous inhibitors such as angiostatin, endostatin, thrombospondin, and heparin. Treatment strategies have been developed that aim to inhibit angiogenesis:
Bevacizumab, a humanized monoclonal antibody binding VEGF, is now approved for use in patients with relapsed glioblastoma.
Several “small-molecule” inhibitors of VEGF receptors are at various stages of development.
Sunitinib has been used in relapsed atypical and malignant meningiomas with modest success.15
Cediranib failed to demonstrate survival benefit in a phase III trial for patients with relapsed glioblastoma.16
The addition of cilengitide, a cyclic pentapeptide inducing apoptosis of growing endothelial cells through inhibition of their αVβ3 integrin interaction with the matrix proteins vitronectin and tenascin, did not improve outcome in patients with newly diagnosed glioblastoma.17
Therapies based on immune-mediated strategies aim to increase immune responses to the tumor.
Tumor vaccination makes use of immunogenic peptides, attenuated autologous tumor cells, or dendritic cells loaded with tumor antigens.18 Cytotoxic T-cells infiltrate the injection site and, by exposure to the antigens, are “primed” to attack tumor cells in the brain. The immune reaction can be enhanced by irradiation, transfection with cytokine genes, or transfection with major histocompatibility complex (MHC) class II genes. A “one-fits-all” immunization strategy using an oligopeptide resembling a somatic mutant of EGFR (EGFRvIII) has been tested in a phase II study with promising results,19 and a registration trial is ongoing.
Regulators of T-cell response: The T-cell response to an antigen presented on major histocompatibility complex molecules expressed by antigen-presenting cells (APCs) is modulated by numerous inhibitory and activating molecules on APCs, for example B7.2 (binding to cytotoxic T-lymphocyte–associated antigen 4 [CTLA-4]) and B7-H1 (PD-L1, binding to programmed death 1 [PD-1]).20 Upregulation of inhibitory molecules by cancer cells, stromal cells, and immune cells in the cancer microenvironment enables tumor cells to evade an immune attack. Antibodies blocking CTLA-4 and PD-1 have recently been shown to have activity in a variety of cancers,21,22 and a registration trial is underway for patients with relapsed glioblastoma.
Other treatment concepts have been designed to overcome various mechanisms by which gliomas evade recognition by the immune system including cytokines (TGF-β, prostaglandin E, IL-10), defective cytokine receptors on tumor-infiltrating T lymphocytes, and inhibitory molecules expressed on tumor cells, stromal cells, and immune cells. Examples for this category of investigational therapies are the transfection with antisense TGF-β23 or decorin, a TGF-β–binding and TGF-β–inhibiting proteoglycan.
Adapted microorganism-related function:
Cytokines fused to bacterial toxins have been designed to enter tumor cells via binding to selectively expressed cytokine receptors and then kill them after release of the toxin. A phase III clinical trial of cintredekin besudotox (a fusion protein of IL-13 and pseudomonas exotoxin) administered intracerebrally through convection-enhanced delivery failed to demonstrate a survival benefit.24
Viruses have been genetically modified to preferentially replicate in, and destroy, cancer cells. For example, ONYX-015 is replication competent but lacks E1B, a viral protein that binds and inactivates p53, and thus can only replicate in cells with loss of TP53 function, an early event in the pathogenesis of gliomas.25 The safety of oncolytic viruses has been demonstrated but delivery systems have thus far proven insufficient. Viruses with enhanced tumor cell selectivity and killing are under development.26,27
Novel delivery strategies are designed to enhance tumor cells exposure by circumventing the BBB.
Intraoperative injection of resection margins with viral vectors or oncolytic viruses has proven inefficient.
Convection-enhanced delivery via intraoperatively or stereotactically placed infusion catheters achieves a wider distribution of therapeutic agents.
Therapeutic compounds (cytotoxic chemotherapy agent, vector, antibody etc) can be packaged into polymer nanoparticles from where they are released over a modifiable period of time. The nanoparticles are coated with various surface molecules in order to enhance selectivity for the targeted cell population.28,29
Neuroprogenitor cells have been used in animal experiments to deliver vectors or therapeutic genes into tumors. They home to brain tumors even when injected into the contralateral cerebral hemisphere, raising hope that they may be able to track down migratory brain tumor cells.30
Gene therapy of brain tumors encompasses a wide spectrum of strategies.31,32
Viral vectors are used to express transgenes activating cytokines or chemotherapies. Therapeutic success depends on transfection efficiency and the “bystander effect”—killing of cells lacking the transgene by the cellular reaction to successfully transfected adjacent tumor cells. Tumor selectivity can be enhanced by various means: incorporation of ligands or antibodies targeting tumor-specific surface proteins into the capsid of adenoviral vectors; use of replication-conditional viral vectors or retroviruses; and placement of genes essential to virus replication under the control of promoters that are selectively active in gliomas (such as the nestin or human telomerase reverse transcriptase [hTERT] promoter).
Delivery strategies for viral or nonviral vectors (naked DNA, polycationic polymers, and liposomes) include stereotactic injection into the tumor or intraoperative insertion into the wall of the resection cavity, convection-enhanced delivery, and intra-arterial or intraventricular application.
Neuroepithelial tumors
Astrocytomas
Oligodendrogliomas
Ependymomas
Choroid plexus tumors
Embryonal tumors
Neuronal and neuroglial tumors
CNS lymphoma
Schwannomas
Meningiomas/Hemangiopericytomas
Masses in the sellar region
Masses in and around the spinal cord
Metastatic tumors
CASE 44-2
A 19-year-old man presents with several episodes of altered level of consciousness. During these episodes, the patient has stereotypies and lip smacking. After the episodes, he is exhausted and requires half an hour to return to his baseline. When he presents to the ED, an urgent CT is done, which shows postsurgical changes in the left temporal lobe. The family says that the patient had an astrocytoma that was resected in his early teens. You are consulted to help with this patient.
Tumors composed of cells of astrocytic differentiation are classified into 4 different grades. The well-circumscribed noninfiltrative astrocytomas (WHO grade I), which include pilocytic astrocytoma, pleomorphic xanthoastrocytoma, and subependymal giant cell astrocytoma, may be curable depending on location, and rarely ever, if at all, undergo malignant transformation. On the other side, diffuse astrocytomas (WHO grade II), anaplastic astrocytoma (WHO grade III), and glioblastoma multiforme (WHO grade IV) are characterized by diffusely infiltrative growth pattern. They are considered incurable, and grade II or III neoplasms are at risk of malignant transformation.33
As a rule of thumb, the noninfiltrative astrocytomas present in children and adolescents and the infiltrating types in the adults.
The common noninfiltrative astrocytomas include:
Pilocytic astrocytoma
Pilomyxoid astrocytoma
Pleomorphic xanthoastrocytoma
Subependymal giant cell astrocytoma
Pilocytic astrocytomas (WHO grade I) are slow-growing, well-circumscribed glial tumors of childhood and young adulthood. They represent approximately 6% of all intracranial tumors.33–35
The cerebellar hemispheres are the most commonly affected site. Less commonly, they are encountered in optic nerve, chiasm, cerebral hemispheres, brainstem, infundibulum, hypothalamus, 3rd ventricular region, and spinal cord.
On neuroimaging studies, these tumors have nodular enhancing and cystic components.
Histopathologically, tumor cells stain for glial fibrillar acidic protein (GFAP) and contain eosinophilic granular bodies and Rosenthal fibers (intermediate filaments with entrapped cytosolic proteins).
Vascular proliferation is responsible for the contrast enhancement seen on preoperative MRI studies.
It can occasionally cause a diagnostic confusion with a high-grade glioma, especially when the biopsy sample is small.
Surgical resection of pilocytic astrocytoma when feasible has the potential to be curative.36
Prognosis quoadvitam is favorable (overall survival at 5 years [OS-5] nearly 100% and OS-10 95%). Functional outcome is dependent the tumor’s location.35
What are the more aggressive forms of pilocytic astrocytoma called, and where are they typically found?
Pilomyxoid astrocytomas (WHO grade II) are clinically more aggressive variants of pilocytic astrocytomas.
They occur most commonly in the hypothalamic/chiasmatic region.
Distinctive histologic features include a monomorphic population of neoplastic pilocytes in a prominent myxoid background stroma.
Unlike most pilocytic astrocytomas, in pilomyxoid astrocytoma, Rosenthal fibers are either found in small amounts or completely absent.36
Infants and children (median age 10 months) are the main affected groups. Local recurrences and spread through cerebrospinal fluid do occur in these lesions.1
What are pleomorphic xanthoastrocytomas, and in what anatomical distributions are they typically found?
Pleomorphic xanthoastrocytomas (PXA; WHO grade II) account for fewer than 1% of glial neoplasms and typically manifest in children and young adults.
Patients often have a long-standing history of seizures.
Most commonly, they are located in the temporal lobe, close to the cortical surface.
Characteristic histologic features are multinucleated and lipidized giant cells with bizarre nuclei, which tend to express the astrocytic marker GFAP.
There is low proliferative activity. Imaging studies reveal well-demarcated lesions, but there may be invasion of adjacent brain, perivascular spaces and meninges. This phenomenon does not affect prognosis negatively.
Many PXAs follow a benign clinical course (long-term survival after tumor resection).
Whether malignant transformation occurs in classical PXA remains a matter of debate.1,36
Subependymal giant cell astrocytoma (SEGA; WHO grade I) is a benign tumor composed of large gemistocytes or ganglion-like cells.
It uniquely occurs in patients with tuberous sclerosis complex.
These individuals often present with signs of obstructive hydrocephalus due to the proximity of the tumor to the foramen of Monro.
Surgical resection is curative.36
Everolimus, an mTOR inhibitor, has been shown to shrink SEGA, creating the possibility of chemotherapy where surgery is not feasible or fails, or when tumors recur.9,37
CASE 44-2 (continued)
You are not sure whether seizures in this patient represent tumor recurrence or are due to postsurgical changes. You locate the patient’s oncologist who informs you that the patient had a pleomorphic xanthoastrocytoma resected with curative intent. She wants you to start him on an antiepileptic drug (AED) and to do a contrast-enhanced MRI of the brain. She will follow up within 2 weeks.
CASE 44-3
A 65-year-old was admitted to hospital with new-onset neurological symptoms. The patient was in her normal state of health until about 3 months ago when she began to exhibit changes in her personality. She appeared puerile and insensitive. She was having problems organizing herself at home. Over the next few weeks, she started developing left-sided weakness, which affected her arm predominantly. You were consulted to assess her and found her to have headaches, papilledema, left upper motor neuron pattern of weakness, and some behavioral oddities. You suspect a brain tumor in this patient.
Infiltrative astrocytomas are the most common primary neoplasms of the brain in adults and constitute more than 60% of all neuroepithelial tumors. The histologic grade shows a direct correlation with prognosis. Low-grade diffuse astrocytoma tend to occur in a younger population (4th and 5th decades), whereas Grade IV astrocytoma affects a more elderly population (6th decade). Men are at a 1.5 times higher risk.35
Astrocytomas can be divided into four grades:
Grade I astrocytomas: see above
Grade II astrocytomas: mostly diffuse astrocytomas
Grade III astrocytomas: anaplastic astrocytoma
Grade IV astrocytomas: better known as glioblastoma
Diffuse astrocytomas (WHO grade II) are characterized by slow growth, low mitotic activity, but a high tendency to infiltrate and spread into adjacent brain structures.
Mutations of isocitrate dehydrogenase (IDH) gene occur early in their pathogenesis. This mutation changes the molecular substrate of the IDH enzyme, which normally acts to convert isocitrate into α-ketoglutarate. When mutated, it catalyzes the conversion of α-ketoglutarate to 2-hydroxyglutarate (2HG). 2HG is an oncometabolite whose accumulation is linked to the formation and malignant progression of gliomas.11–13
Diffuse astrocytomas are most commonly located in the frontotemporal lobes; in children, however, there is a predilection for the brainstem.
They present with a biphasic age distribution with the first peak in childhood (age 6–12 years) and a second peak in early adulthood (age 30–50 years).38
More than half of the patients present with seizures.38,39
Other clinical signs are secondary to mass effect in the specific location of the brain where they originate or secondary to increased intracranial pressure.40
Imaging findings typical of diffuse astrocytomas are hypo- to isodense masses on CT and hypoto- isointense on T1W images and hyper-intense on T2W images of MRI. There is no enhancement after administration of contrast dye. Cystic components are frequently encountered.40
A patient diagnosed with diffuse astrocytoma has a median overall survival in the range of 6–8 years.41 Prognostic factors that are associated with improved survival are age less than 40 years, presence of seizures, and a high Karnofsky performance score (KPS) (>70).39,41,42
The optimal treatment for diffuse astrocytoma remains a topic of debate.
Surgery: Tumor resection, to the largest extent possible without neurologic injury to the patient, is generally performed, although randomized controlled trials have not been performed to clarify the role of extent of surgical removal. A biopsy is recommended in unresectable tumors as it provides tissue for morphologic and molecular typing.43 After surgery, patients, in whom complete resection was accomplished, are followed expectantly. Individuals with residual tumor or neoplasms with high-risk features (see above; IDH wild type) are increasingly provided with adjuvant therapies.44
Traditionally radiation therapy is chosen as first-line therapy for astrocytic tumors. However, this paradigm has been challenged. An approach stratified by molecular features (MGMT promoter methylation, IDH status), tumor location, and size appears logical but awaits prospective evaluation.
Radiation therapy is an effective therapy for patients with symptomatic and progressive low-grade astrocytomas. Adverse effects of normal brain exposure to ionizing radiation have to be carefully weighed against the benefits of therapy. Early treatment of an asymptomatic patient after complete tumor removal is of no benefit.45 Dose escalation does not improve outcome.46
Alkylating agents (temozolomide (TMZ), lomustine, or procarbazine) have shown activity.44,47 MGMT expression in tumors, as measured by promoter methylation assays, has been used as a predictor of chemotherapeutic response to TMZ.48
Anaplastic astrocytomas (AA, WHO grade III) arise from low-grade diffuse astrocytomas through malignant degeneration, although patients are frequently diagnosed without indication of a less malignant precursor lesion.
On a microscopic level, AAs are characterised by increased cellularity, hyperchromatic and polymorphic nuclei, as well as an increased mitotic index. Early signs of vascular proliferation may be seen, but necrosis is absent.1,33
The mean age of patients with AAs is in the early 40s.
Gross total resection is performed whenever feasible. AAs require adjuvant therapies.
Radiation therapy and chemotherapy (temozolomide, PCV) are of comparable efficacy.49
Molecular predictors of response to treatment await prospective validation (MGMT promoter methylation, IDH mutation).
Concomitant radiochemoytherapy (Stupp protocol) may be superior to sequential treatment in selected patients.50
Prognosis is much less favorable than for low-grade astrocytomas.
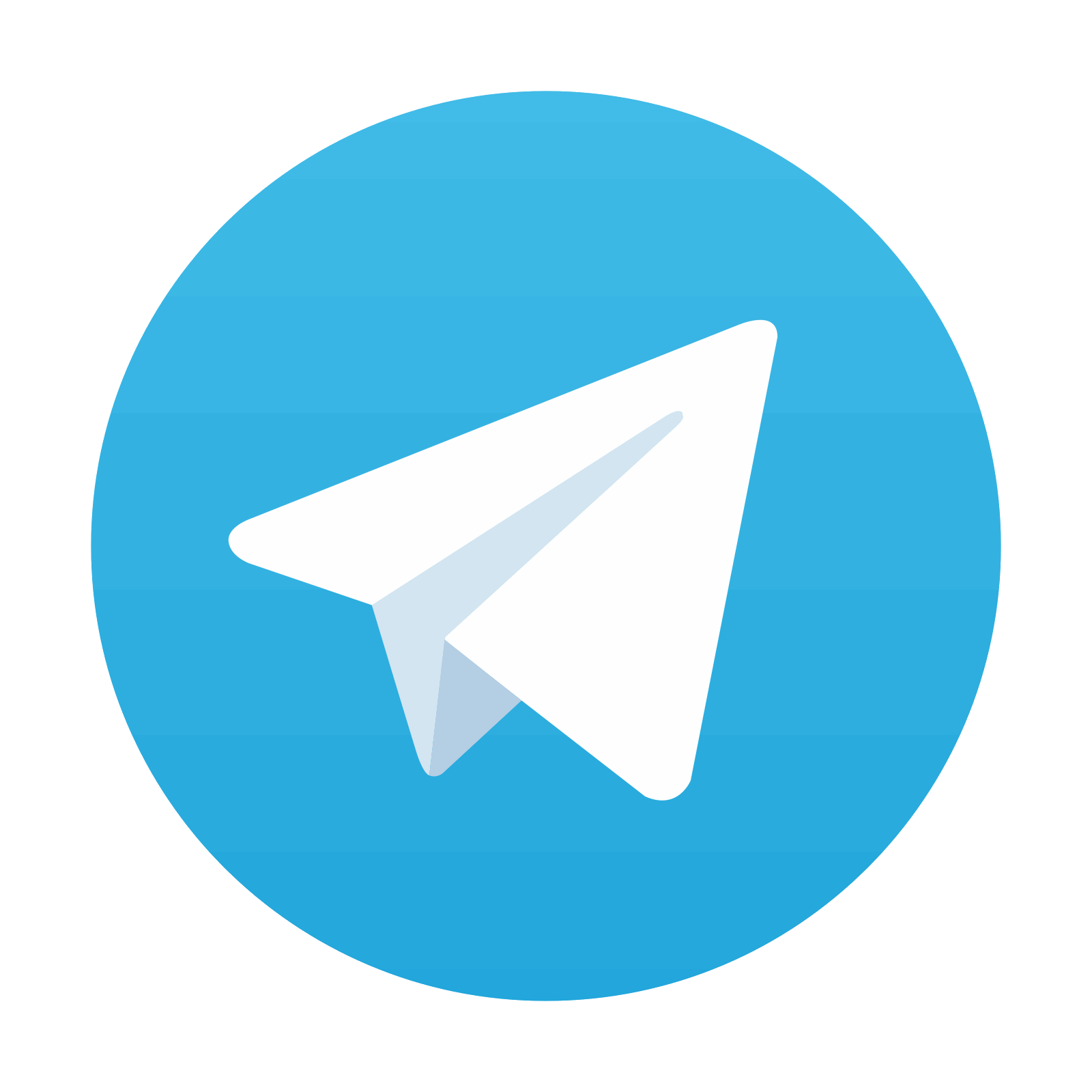
Stay updated, free articles. Join our Telegram channel

Full access? Get Clinical Tree
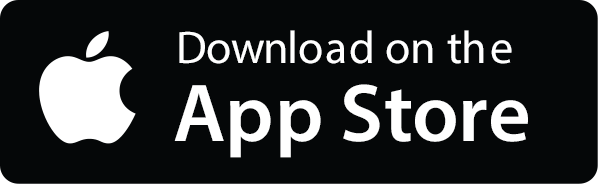
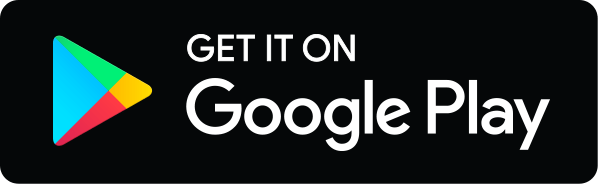