Fig. 23.1
Example of an nTMS mapping, performed on a 63 year-old female patient with a left hemisphere brain tumor, suffering from a mild hemiparesis on her right side. In the left panel, all spots stimulated on the left hemisphere are displayed. In the right panel, the image displays only the spots where a muscle response was observed (MEP > 50 μV peak-to-peak amplitude). Three different hand muscles (abductor pollicis brevis, abductor digiti minimi, first dorsal interosseus) and one leg muscle (tibialis anterior) were recorded in this case. The color coding corresponds to the intensity of the response, whereby red indicates small responses (MEP 50–500 μV), yellow indicates medium responses (MEP 500–1,000 μV), and white indicates large responses (MEP > 1,000 μV)
Overview of the Safety and Risks of Transcranial Magnetic Stimulation
When thinking about the safety and risks of transcranial magnetic stimulation, we should recognize that there are different types of TMS: single-pulse TMS, paired-pulse TMS, and repetitive TMS (rTMS). The influence of the magnetic field from these three different types of TMS procedures differs greatly, and thus they have different safety profiles. The modality used for mapping the motor cortex in patients with brain tumors is single-pulse TMS, in which the electro-magnetic influence is the lowest of the three different types of TMS. Generally, single-pulse TMS is considered to have no significant risk from its more than 20 years of clinical experience (Rossi et al. 2009; Groppa et al. 2012). Nonetheless, it is recommended to use a short safety checklist such as a questionnaire developed by “The Safety of TMS Consensus Group” (Rossi et al. 2011) to identify patients with increased risk for performing TMS such as patients with a history of loss of consciousness due to seizures or syncope, brain diseases or medications associated with increased seizure risk, the presence of implanted metallic devices, and pregnancy (Groppa et al. 2012).
In general, TMS has some direct safety concerns such as heating and magnetic field exposure (Rossi et al. 2009). The heating effects to the brain induced by a single-pulse TMS is estimated to be less than 0.1° Celsius (Ruohonen and Ilmoniemi 2002). As for magnetic field exposure, its exposure to both patients and operators should be taken into account. Magnetic field exposure induced by single-pulse TMS to patients seems not to cause a significant risk, since the total time of exposure is so short, but the potential risk of long-term adverse consequences for TMS operators has not yet been adequately studied (Rossi et al. 2009).
TMS has also several known potential adverse events (Rossi et al. 2009). The adverse events can be divided into two subgroups: (1) adverse events reported both in single-pulse TMS and paired-pulse or rTMS and (2) adverse events reported only in paired-pulse and/or rTMS but not in single-pulse TMS. The former subgroup includes events such as seizure induction, syncope, transient headache, local pain, neck pain, toothache, paresthesia, and transient auditory threshold changes. The latter subgroup contains events such as transient cognitive/neuropsychological changes, induced currents in electrical circuits of medical devices, structural brain changes, histotoxicity, and other transient biological effects such as hormonal change (Rossi et al. 2009). The latter set of adverse events only from paired-pulse or rTMS will not be discussed further here in this chapter about single-pulse TMS.
Induction of seizures is the most severe acute adverse effect for TMS, but most TMS-associated seizures were induced during repetitive TMS (Rossi et al. 2009). Less than 5 % of all the reported TMS-related seizures occurred during single-pulse TMS (Groppa et al. 2012), but this only provides a rough approximation, since it remains unknown what percent of all TMS usage is repetitive TMS. The exact incidence of seizures after single-pulse TMS is not known. Of course this rate should not be underestimated, but it seems to be very low. The question of “what percentage of single-pulse TMS sessions result in a seizure”, is a very important issue for patient counseling and safety. Since the literature lacks firm answers, it should be investigated with large-scale multi-institutional studies in the near future. In most cases, single-pulse TMS-related seizures occurred in patients with known structural brain pathology or patients under medication such as amphetamines, lithium, and chlorpromazine, which can lower the seizure threshold (Groppa et al. 2012). Nonetheless, seizures can occur in patients without known risk factors. For example, Kratz et al. (2011) reported on a healthy subject who developed seizure after single-pulse TMS during motor threshold estimation. The risk and benefit balance must always be fully discussed with the patient before TMS is used.
Patients with rolandic tumors frequently suffer from symptomatic seizures and therefore may seem to be a patient group at high-risk of TMS induced seizures. Hufnagel et al. applied TMS to 13 patients with medically intractable complex partial seizures. They found that the epileptic focus was activated by TMS in 12 out of 13 patients, but clinical seizure was induced only in one patient (Hufnagel et al. 1990). Based on a literature review (Schrader et al. 2004), the risk of single-pulse TMS-induced seizure in patients with epilepsy ranges from 0.0 % to 2.8 %. In any case, the TMS examiner should prepare space and medications for managing a seizure, should it occur.
Although TMS-associated syncope is also a rare adverse event, it is more likely to occur than seizure. Because of the lack of systematic studies, the incidence of TMS-associated syncope remains unknown, but many laboratories have experienced it (Groppa et al. 2012). This is an area deserving more attention, and until better reviews have been published, TMS users should be aware of the risk of syncope and proceed with caution in this regards.
As for pain, single-pulse TMS seems to be generally well-tolerated and experienced by most participants as painless (Rossi et al. 2009), but the literature specifically addressing this point also remains scant. As mentioned above though, transient headache, local pain, neck pain, and toothache, as well as paresthesia have all been reported from single-pulse TMS (Rossi et al. 2009). We would suppose though that these pains were at least transient, if not also mild. As for transient auditory threshold changes, TMS produces a loud clicking sound from the coil, up to 120–130 dB, and all patients should be required to wear earplugs during the procedure to prevent transient auditory threshold changes, so called “noise induced temporary threshold shift” (Groppa et al. 2012).
Three reports have mentioned adverse events from nTMS in patients with rolandic tumors (Paiva et al. 2012; Krieg et al. 2012b; Forster et al. 2011). To summarize these reports, there were two adverse events (unpleasantness in one patient, and headache in one patient) out of 31 nTMS sessions in 30 patients. Although this is too small a sample size to support reliable estimates, provisionally it suggests that about 1 in 15 patients will experience such adverse events. Larger multi-institutional registries would help to better elucidate the frequency of these events, as well as monitor the occurrence of other possible rare adverse events.
Furthermore, we must keep in mind that some neurosurgical patients harbor metals such as titanium skull plates for craniotomy closure or DBS electrodes inside the cranium, both of which present risks in the presence of an electromagnetic field such as TMS. Titanium skull plates may be of greatest concern, since TMS is performed not only pre-operatively, but also post-operatively on these patient populations. Rotenberg et al. assessed the safety of applying rTMS (which has greater electromagnetic influence than single-pulse TMS) to patients with titanium skull plates. They found that small titanium skull plates are not likely to heat sufficiently to injure the surrounding brain tissue during conventional low-frequency rTMS protocols, and they concluded that low-frequency rTMS may be safe for patients with small titanium skull plates that are in the stimulation site (Rotenberg et al. 2007). Besides the problem of heating, one must consider the possibility that the induced currents might displace the titanium skull plates (“Lorentz interaction”). Yet only minimal displacement of loose titanium plates during simulated rTMS has been observed (Rotenberg et al. 2007). As for DBS electrodes, two ex vivo studies have shown that the current and voltages induced by TMS in the deep brain electrodes are smaller than those induced by DBS itself and seemed to be a safe level (Kumar et al. 1999; Kuhn et al. 2004). nTMS is still a quite new technology and further monitoring of its safety is needed from the broader community of clinical users. Clinical users should report any incidents of adverse events that they observe in clinical usage, ideally be sending a brief letter describing the incident to a scientific journal indexed by PubMed.
Review of Studies on the Accuracy of Navigated Transcranial Magnetic Stimulation
To establish the validity of any new method of non-invasive brain mapping, its accuracy must be assessed relative to the gold standard of DES. Several studies have assessed the ability of nTMS to identify the motor cortex and delineate the cortical representation of individual muscles, in order to evaluate its reliability and accuracy for motor mapping. We conducted a review of the literature up to June 2012. The search terms we used on PubMed were: “transcranial magnetic stimulation”, “TMS”, “direct cortical stimulation”, “direct electrical stimulation”, “DCS”, “DES”, “motor cortex”, “M1” and “brain tumo(u)r”. We reviewed the abstracts of those reports, and if they reported on evaluating patients with rolandic tumors with both nTMS and DES, then we extracted information from the report. A total of eight studies meeting these criteria were identified (Table 23.1).
Table 23.1
Summary of eight studies comparing nTMS to DCS in terms of motor cortex identification
Reference | N patients with TMS (n comparisons of nTMS and DES) | Histology | Output muscles of nTMS | Method of measurement | Distance between muscle representations identified by nTMS and DCS (mm) | Other modalities compared | Study limitations |
---|---|---|---|---|---|---|---|
Krings et al. (1997) | 2 | Brain tumors | FCR | Compare single hotspots | <10 | None | Small sample size; hotspot method; semi-quantitative |
(2) | Oligoastrocytoma III 3 | FDI | |||||
Oligodendroglioma 1 | GG | ||||||
Picht et al. (2009) | 10 | Brain tumors | TM | Compare single hotspots | Mean (SD) | None | Hotspot method; semi-quantitative |
(10) | Glioblastoma 3 | 3.4 (3.0) | |||||
Astrocytoma III 3 | |||||||
Astrocytoma I 1 | |||||||
Metastasis 3 | |||||||
Kantelhardt et al. (2010) | 1 | Brain tumor | BR, APB | Compare single hotspots | <5 | fMRI (n = 6) | Single case; hotspot method; semi-quantitative |
(1) | Meningioma 1 | ADM | Unquantitative | ||||
Picht et al. (2011a) | 20 | Brain tumors | APB n = 15 | Compare single hotspots | Mean (SE) | None | Hotspot method |
(17: APB n = 15 TA n = 8 ) | Glioblastoma 5 | TA n = 8 | APB 7.83 (1.18) | ||||
Astrocytoma III 3 | TA 7.07 (0.88) | ||||||
Metastasis 7 | |||||||
Meningioma 3 | |||||||
Lymphoma I 1 | |||||||
Forster et al. (2011) | 10 | Brain tumors | TM n = 5 | Compare single hotspots | Overall mean (SD) | fMRI (n = 10) | Hotspot method |
(11 sessions in 10 patients) | Glioblastoma 3 | TA n = 4 | 10.49 (5.67) | Mean (SD) versus DCS | |||
Astrocytoma III 2 | FDI, AHB n = 3 | Median[range] | 15.03 (7.59) | ||||
Astrocytoma II 3 | BC, QC n = 2 | APB | |||||
Astrocytoma I 1 | ADM, OO n = 1 | 12.8 [2.6–27.6] | |||||
Metastasis 1 | ED: 10.6 [7.8–16.4] | ||||||
TA: 11.1 [5.9–15.9] | |||||||
Krieg et al. (2012b) | 26 | Brain tumors | APB | Compare borders between positive and negative stimulation points | Mean (SD) | fMRI(n = 24) | Complicated idiosyncratic measurement method |
(14) | Glioblastoma 13 | ADM | 4.4 (3.4) | Mean (SD) versus nTMS – upper extremity | |||
Astrocytoma III 3 | FCR | ||||||
Astrocytoma II 2 | BC | ||||||
Astrocytoma I 1 | TA, GN | 9.8 (8.5) | |||||
Metastasis 7 | Lower extremity | ||||||
14.7 (12.4) | |||||||
Paiva et al. (2012) | 6 | LGG | Hand and forearm (not specified) | Center-of-gravity approach | Mean | None | Small sample size; statistical methods were not explained sufficiently |
(6) | Astrocytoma II 6 | 4.16 | |||||
Tarapore et al. (2012) | 24 | Gliomas | ADM | Compare single hotspots | Median (SE) | MEG (n = 23) | Small sample size; |
(8 points in 5 patients) | Glioblastoma 7 | APB | 2.13 (0.19) | Median (SE) versus nTMS – ADM: 8.98 (1.41) | Hotspot method | ||
Astrocytoma III 8 | OO | ||||||
Astrocytoma II 8 | APB: 6.16 (0.73) | ||||||
Treatment effect 1 |
The first study to compare nTMS to DES for evaluating the motor cortex was by Krings et al. (1997). A mechanical stereotactic arm was used for TMS navigation. They compared areas of motor responses identified by both nTMS and DES in two patients with rolandic tumors. The discrepancy between nTMS and DES maps was never more than 1 cm. The major limitations of this study are that it reported on only two cases, and it used a homemade system that is not commercially available.
The next study was published more than a decade later (Picht et al. 2009). In this study, the motor cortex of 10 patients with rolandic tumors were evaluated using a homemade nTMS system in which an electromagnetic navigation system was integrated with TMS for the purpose of positioning the TMS coil. The mean (SD) [range] distance between hotspots of the two modalities was 3.4 (3.0) mm [0–7] mm. The limitation of this study was that the preoperative and intraoperative mappings were performed in the same predefined 5-mm raster, so the resulting comparative data were semiquantitative (Picht et al. 2011a). This system is not commercially available, so the applicability of the findings is limited. The next study (Kantelhardt et al. 2010) evaluated hotspots determined by nTMS and DES in two patients with brain tumors. In one patient the distance between hotspots was estimated as less than 5 mm, but in the other case the comparison referred to post-op nTMS. This study is unreliable because of the very small sample size and inadequate reporting.
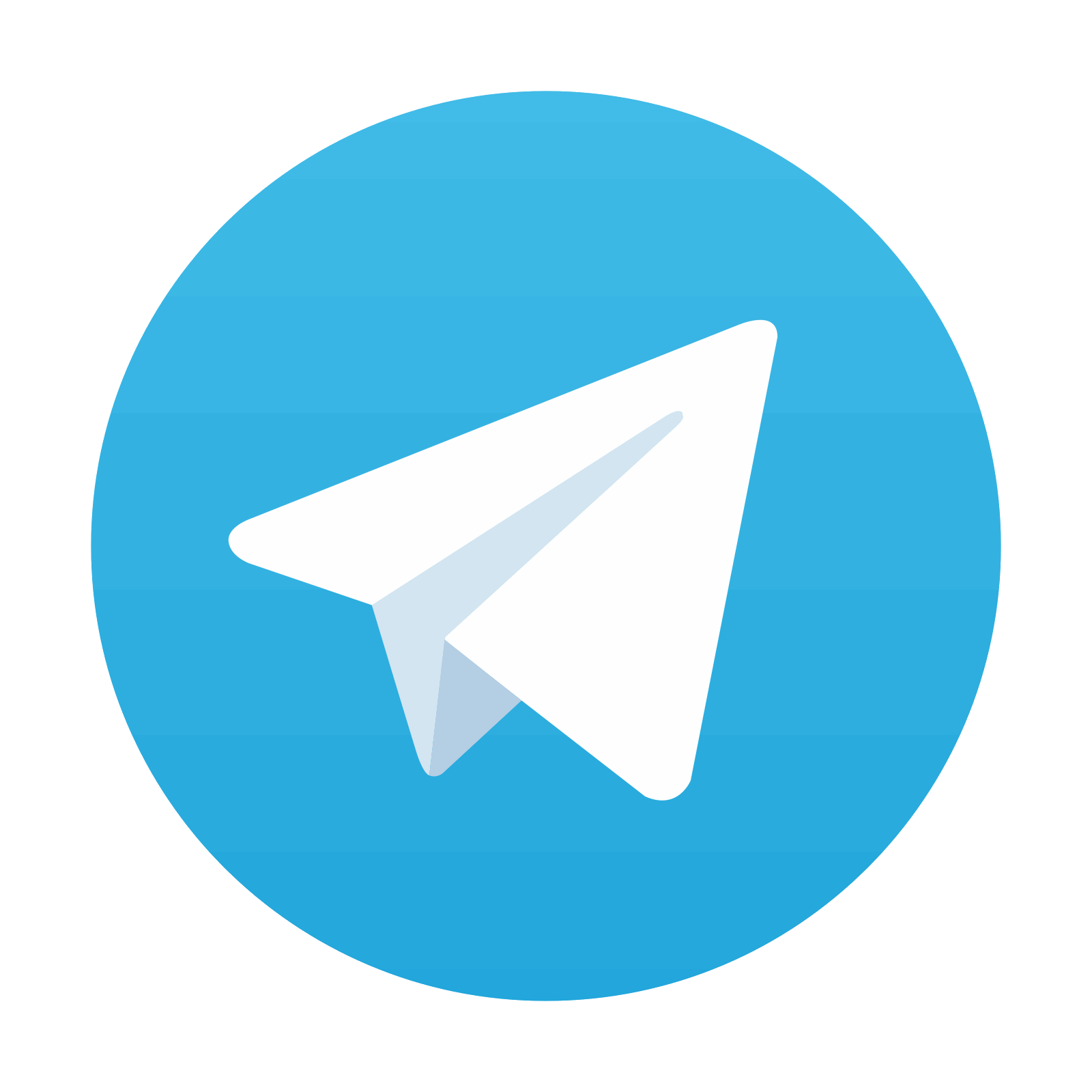
Stay updated, free articles. Join our Telegram channel

Full access? Get Clinical Tree
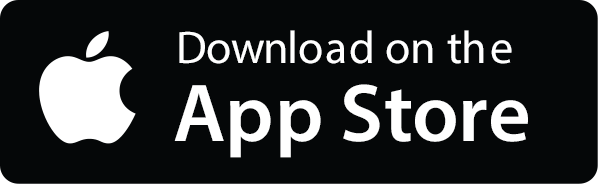
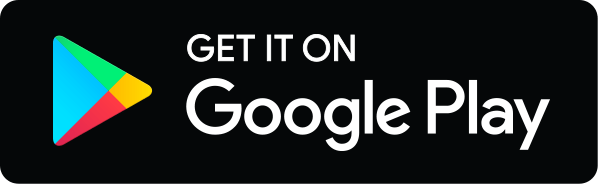