Case Report
A 63-year-old man presented on referral from a neurologist specializing in movement disorders, with medically intractable Parkinson disease (PD). The patient had levodopa-induced dyskinesias, rigidity, bradykinesia, freezing, and some rest tremor bilaterally. After neuropsychological, speech and swallowing, and on/off levodopa testing, the patient was considered an appropriate candidate for bilateral deep brain stimulation (DBS) electrode implantation. The target chosen by the neurologist was the subthalamic nucleus (STN). The patient underwent this image-guided procedure in two stages, both under general anesthesia, the first stage being the implantation of the electrodes, and the second stage, the implantation of the internal pulse generator (IPG). The patient did well after these procedures and 3 weeks postoperatively started stimulation with the goal to optimize his DBS system and drug management of his PD symptoms.
His initial response to DBS was encouraging, with resolution of his dyskinesias, more consistent “on time,” and a significant reduction in his dosage of Sinemet. However, at approximately 4 months postoperatively, the patient began to develop uncontrolled flailing movements of his left arm. This was concerning for hemiballismus, and the patient underwent high-resolution 1.5T magnetic resonance imaging (MRI) with contrast. This showed what appeared to be a small ring-enhancing lesion around his right STN electrode, with some minimal enhancement around the left STN electrode. Both distal electrodes also appeared to have a much wider surrounding area of T2 hyperintensity, interpreted as “edema.” At this time, the patient showed no systemic signs of infection. One week after the development of his hemiballismus, his entire DBS system, including the generator and extension leads, was removed. The patient was initially placed on broad-spectrum cerebrospinal fluid (CSF) penetrating antibiotics, but when the culture from the right electrode tip yielded Propionibacterium acnes , the regimen was simplified to just vancomycin. He was treated with a 3-week course of parenteral vancomycin and then observed off antibiotics for 6 weeks. His hemiballismus stabilized but did not improve, and he developed no additional neurologic deficits. Two months after the explant of his DBS system, his entire system was replaced, this time using a globus pallidus internus (GPI) target bilaterally. GPI DBS alleviated his left arm hemiballismus as well as his dyskinesias and other cardinal symptoms of PD. Over the course of two years, he has done well with DBS at this location and has had neither recurrence of his hemiballismus nor signs of recurring infection.
Discussion of the Case Report
This case is illustrative in several ways. First, it highlights what is an uncommon, but serious, complication of DBS implantation, namely infection. Most infections become apparent at the generator site, but rarely, the initial presentation may be at the brain electrode site, with attendant neurologic problems. MRI scanning is the definitive test in these cases because systemic or laboratory signs of infection are insensitive. As discussed below, the treatment for this complication is partial or complete removal of the system, parenteral antibiotics, followed by a short course of oral antibiotics. In this instance, GPI DBS was effectively used to treat a complication of STN damage from the infection: hemiballismus.
Complications of DBS
DBS has become an important therapy for a variety of neurologic conditions, including movement disorders, epilepsy, and potentially psychiatric conditions (reviewed by Holtzheimer and Mayberg ). For movement disorders in particular, DBS has been proven to be more effective than the best medical therapy at the highest level of evidence. Remarkably, complications of DBS surgery have diminished steadily over the past 25 years, yet the classification of DBS complications remains unstandardized. Most of the morbidity of DBS falls into three categories: procedure-related, hardware-related, and stimulation-related complications (adverse events). Surgical complications include intraoperative events and those arising within 30 days of the procedure: cerebrovascular events (strokes), postsurgical edema, seizures, electrode malposition, and miscellaneous complications associated with any surgery. Infections are best classified as hardware-related complications considering that the incidence of infections generally increases with implanted foreign bodies. As such, hardware-related complications include infections and erosions, lead migration, hardware fracture, and electrical malfunction. Stimulation-related complications revolve mainly around so-called “off-target” effects.
Utilizing the following categorization, this chapter will review the current evidence with regard to each of these complications and discuss best practices to avoid these complications. This chapter intentionally leaves aside patient selection and target selection, despite both being fundamentally important in the success of DBS therapy.
- 1.
Procedure-related complications:
- a.
Stroke
- i.
Intracerebral hemorrhage (ICH)
- ii.
Nonhemorrhagic transient or permanent neurologic deficit
- 1.
Ischemic infarction
- 2.
Venous infarction
- 3.
Postsurgical edema
- 4.
Seizures
- 1.
- i.
- b.
Electrode malposition
- a.
- 2.
Hardware-related complications:
- a.
Infection and skin erosion
- b.
Lead migration, lead fracture, and electrical malfunction
- a.
- 3.
Stimulation-related complications:
- a.
Off-target effects
- a.
Procedure-Related Complications
Stroke
Intracerebral Hemorrhage
Perhaps the most devastating complication of DBS electrode implantation is ICH resulting in permanent neurologic deficit(s). Fortunately, the incidence of symptomatic ICH is low, ranging from 0.7% to 3.9% per lead with a permanent neurologic morbidity of up to 1.1% and a very low mortality risk of 0.4% or less.
Systematic reviews of surgical risk for DBS have reported ICH rates of 3.2% to 5%. The risk of ICH is related to the use of microelectrode recording (MER), the number of MER penetrations, and a sulcal or transventricular course of the electrodes. The bulk of the evidence indicates that making multiple instrumented passes into the brain with a microelectrode for recording contributes to the risk of ICH, with the per-trajectory ICH rate estimated at 1.6%. Hypertension further increases the ICH risk by 2.5-fold.
ICH related to DBS further divides equally between asymptomatic and symptomatic, 1.9% and 2.1%, respectively. Hemorrhages resulting in permanent deficit or death are estimated at 1.1%. The incidence of hemorrhage in studies adopting an image-guided and image-verified approach without MER was significantly lower than reported with other operative techniques for total number of hemorrhages, asymptomatic and symptomatic hemorrhages, and hemorrhage leading to permanent deficit. Although the risk of MER-guided versus image-guided DBS implantation has not been subjected to a randomized controlled trial, multiple studies have shown that there is an associated risk of MER mapping. It is also worth emphasizing that MER guidance has never been demonstrated to be more effective at target localization than image guidance. Whether MER guidance adds substantively to the efficacy of DBS electrode localization and clinical outcome, to an extent that would justify the risk, remains an unanswered question.
Best Practice
Keeping the number of MER penetrations to a clinically indicated minimum and avoiding sulcal or transventricular electrode trajectories can mitigate the risk of ICH and intraventricular hemorrhage during DBS electrode implantation. Intraoperative hypertension (≥160 mm Hg systolic) has been associated with ICH, so blood pressure must be carefully monitored, particularly in procedures performed under local anesthetic. Postoperative computed tomography (CT) or MRI imaging may be warranted if the patient experiences an unexpected neurologic deficit after implantation.
Full anticoagulation must be reversed (international normalized ratio [INR] ≤1.4) before surgery. Although our practice has been to aim for an INR ≤1.2 for neurosurgical procedures, there is a lack of evidence to support this. Bridge anticoagulant treatment is often not required but may be appropriate in very high risk patients with thromboembolic disorders. So-called “bridging” is usually performed with low-molecular-weight heparin up to 24 hours before the DBS procedure. The timing of the discontinuance of oral anticoagulants must be appropriate to the therapeutic half-life of the drug, typically 4 to 5 days before the surgery. However, each individual case should be carefully assessed for proper timing of discontinuation. Importantly, before DBS implantation, aspirin (ASA) must be discontinued a minimum of 7 to 10 days before surgery. However, many institutions, including our own, still prefer to discontinue ASA and other antiplatelet agents 2 weeks before surgery. NSAIDs should be discontinued 3 to 4 days before DBS surgery.
Nonhemorrhagic Transient or Permanent Neurologic Deficit
Probably the most common reason for a postoperative neurologic deficit is ICH. However, some patients do experience temporary or permanent neurologic deterioration, either from cortical or subcortical ischemia, cortical venous infarct, or perielectrode “edema.” Ischemic infarcts are rarely reported, ranging from 0.3% to 0.9%. Cortical venous infarcts can occur in 1.3% of cases, usually becoming symptomatic only during postoperative day 1. A full recovery can be expected in nearly all cases. Most are avoidable by planning the electrode trajectory away from veins and cortical sulci visible on preoperative enhanced MRI.
Most neurologic deficits appear to be due to “edema” along the electrode trajectory or at the DBS target site. The origin(s) of this “edema,” revealed in some cases by T2 or FLAIR MRI, is not clear. Symptoms usually occur 1 to 2 days postoperatively, whereas the edema might take on average 1 month to resolve. The incidence of MRI signal changes along the electrode trajectory has been estimated around 6.3%, with the majority of cases being asymptomatic. Possibilities include mechanical tissue disruption from passing the insertion cannula(s), DBS electrodes, or microelectrodes. Rarely, this swelling can be indicative of cerebritis, or deep infection, although this complication is rare. Furthermore, if a patient exhibits a neurologic deficit in the immediate perioperative interval and no ICH can be demonstrated, it is highly unlikely that the deficit relates to an infectious etiology.
Seizures can happen around the time of electrode implantation. A metaanalysis has reported a rate of 2.4% occurring acutely within 24 to 48 hours and 0.5% chronically. An abnormal postoperative imaging study demonstrating a hemorrhage, ischemia, or edema increases the risk of seizures by 30- to 50-fold.
Best Practice
Cortical veins should be avoided especially if visualized during planning on preoperative MRI. In the face of limited understanding regarding the trajectory of swelling and infarction, it is difficult to recommend a best practice. Given the relative infrequency of unexpected neurologic deficits not related to ICH, it is tempting to argue that this problem is related to some intrinsic property of the patient’s brain, such as microvascular disease, gliosis, plaque formation, or amyloid angiopathy. It is certainly conceivable that preoperative corticosteroid administration might mitigate symptomatic edema, but this would need to be confirmed with a prospective study. Again, minimizing the number of penetrations may also minimize this complication, but this remains unproven. Clearly, further investigations of this phenomenon are imperative.
Malposition of Electrodes
The fundamental goal of DBS surgery is to accurately implant each electrode precisely at the desired target. In practice there is a fair degree of variance with regard to the “best target” for STN, GPI, and ventral intermediate nucleus (VIM). In the final analysis, the best electrode location is defined by the patient’s clinical outcome and tolerance for off-target effects.
Malpositioned electrodes are infrequently reported. Even a previous literature review encompassing large trials in PD revealed only a 1.6% rate of misplaced electrodes. Many factors can lead to electrode malposition: a misaligned stereotactic frame, instability of the frame, incorrect interpretation of MER, or intracranial air with intraoperative brain shift. Perhaps an underappreciated cause of lead misplacement is lead “deflection,” possibly due to variations in tissue density along the planned path of the electrode, the coronal angle of the trajectory, or superficial collision of the insertion cannula with either the burr hole edges or the dura. It is interesting that larger numbers of patients are being referred to specialized centers for second opinions after poor clinical DBS effects. Consequently, malposition rates may, in fact, be systematically underestimated in the literature. Indeed, a recent review of both CMS (Centers for Medicare & Medicaid Services), and NSQIP (National Surgical Quality Improvement Program) data revealed that somewhere between 15.2% and 34% of DBS electrode placements are removed or revised. The authors estimated that up to 48.5% of revisions may have been due to improper targeting, or to lack of therapeutic effect. Similar findings have also been shown on multiple occasions by another group. Together, these reports make the point that we need to understand the true prevalence and nature of such failures as they occur in the wider surgical community. If these statistics are representative of the degree to which electrodes are malpositioned, this complication alone dwarfs all others combined, by an order of magnitude.
Best Practice
The variance in target selection for DBS seems to be inherent in the field at present. No studies have convincingly demonstrated that minor variations in electrode position within a given nucleus result in a clinically significant difference, and opinions continue to dominate discussions. Proprietary targeting seems to be the norm. Electrodes that are clearly outside the target structure are suspect, although targeting the margin of a nucleus may exploit the fact that DBS recruits axons , not neurons , directly. Off-target effects also limit therapy, and the incidence of these phenomena is not consistently described in the literature.
Once a target has been planned, “off-target” can be defined as greater than a 1- to 2-mm malposition. By comparison, DBS electrodes measure 1.24 mm in diameter. If the average accuracy of DBS electrode placement is within this range, the method for placement can probably be considered “accurate.” In a recent study of image-guided DBS electrode placement, we determined the accuracy of placement was “past target” by 1.66 ± 0.76 mm, and “off plan” by 1.44 ± 0.73 mm, which we consider to be within the “acceptable” range of accuracy. Other authors have reached similar conclusions regarding accuracy. Whether image guidance or MER guidance offers superior placement accuracy is still being debated, although the value of intraoperative imaging and accurate DBS electrode placement verification before leaving the operating room (OR) seems increasingly to be recognized. In all instances, postimplant imaging confirmation of DBS electrode locations should be performed and correlated with improvement on clinical rating scales.
Hardware-Related Complications
Infection
An infection is the most pernicious of all complications after DBS system implantation. Reported infection rates with DBS range from 0% to 15%, which effectively eclipses all other reported postoperative adverse outcomes. It is difficult to determine an average infection rate that could be considered “standard of care.” Nevertheless, the largest available evidence comes from a random effects meta-analysis pooling 3550 patients from 35 studies, averaging 134 patients per study, published between 1997 and 2009 ; it reports a mean infection rate of 4.7% with a large prediction interval ranging from 0.9% to 22%. In comparison, the largest published population reported an infection rate of 0.4% within 30 days of implantation.
It is unlikely that a “true” infection rate, which could be used as standard of care, will ever be determined. With a binomial distribution, more than 1000 patients treated identically would be required to demonstrate that the “true” infection rate could be around 4.7% ± 1.2%, but the required sample size enlarges as the “true” infection rate decreases. With an infection rate of 0.4%, the sample size would be above 15,000. Similarly, with an average of 134 patients per study, the 95% confidence interval for a “true” infection rate spans from 1.7% to 10.5%, representing the reported infection rate for the majority of published studies and leaving the neurosurgical community with uncertainty as to the “true” infection rate.
Alternatively, an acceptable infection rate could be derived from literature in other specialties where similar devices are implanted. Orthopedic surgery is heavily involved with hardware implantation, and the rates of surgical site infections (SSIs) after hip or knee arthroplasties are around 2.18%. The rate of infections after primary implant for implantable cardiac electronic devices ranges from 0.5% to 0.8%, and 1% to 4% for revisions. One would expect that DBS infection rates closer to these should be achievable.
There is a somewhat artificial distinction being established in the neurosurgical literature regarding “infection” based on the timing of the infection. What is reported as an “infection” presents early (<6 months) with the classic signs and symptoms of SSI: erythema, warmth, pain, with or without purulence or drainage. Later surgical site complications (>6 months) are reported as “erosions” irrespective of a proven primary infection. Erosions are thought to be due to skin breakdown, typically over a prominent generator or connector in an elderly patient with thin and fragile skin. By the time these erosions present clinically, hardware is already exposed and there is de facto colonization of the underlying hardware by skin flora. These definitions are somewhat arbitrary. As such, we suggest that we recapitulate the definitions of SSI from the CDC and the American College of Surgeons, while incorporating the extensive neurosurgical experience with ventriculoperitoneal (VP) shunts.
The CDC National Healthcare Safety Network classifies SSI based on the depth of the infection and timing of onset. Depth is divided into superficial, deep, or involving an organ space. Timing of infection is used as a surrogate to the virulence of the infectious organisms and is divided into early (<3 months), delayed (3–24 months), and late (>24 months). However, these time frames do not fully represent the trend found in neurosurgical implants such as VP shunts. Two-thirds of VP shunt infections occur within 30 days, and nearly all infections occur by 12 months. In fact, shunt failures secondary to infection plateau by 3 months, and that plateau remains stable up to 6 months, the latest time point reported. With this in mind, we would suggest that early surgical site complications be composed of superficial infections occurring within 1 month and deep infections occurring within 3 months of surgery. Delayed surgical site complications involve the deep skin tissues and can occur between 3 and 12 months after surgery. Late surgical site complications occur beyond 12 months and are the result of either hematogenous spread of infectious organisms, trauma, or noninfectious erosion. Of note, the American College of Surgeons defines SSI specifically for implants as any infection involving the implanted hardware for the 12 months after implantation, a concept not consistently applied for neurosurgical implants.
Incision dehiscence is obligatorily deep and infected, and these commonly occur within 30 days. Pocket hematomas, which are fortunately rare, fall along an early deep surgical site complication and substantially raise the rate of infection. Erosion without a priori signs of infection are infrequent at 0.48% and most common at the connector site, followed by the pulse generator pocket, and occasionally at the skull-anchoring device.
This classification could provide guidance in managing surgical site complications. In superficial SSI, which occurs only within 30 days of surgery, hardware could be salvaged with an aggressive antibiotic regimen. Deep SSIs, early or delayed, require hardware explantation followed by an aggressive antibiotic regimen to salvage the distant hardware left in situ. Late complications, beyond 12 months, may not always be infectious. Certainly, many individuals who are implanted with DBS are older, often with thin and fragile skin along the scalp and chest wall, and many have lost subcutaneous fat. These patients may be prone to simple failure of the skin overlying the implants due to ischemia, pressure, or abrasion. However, once these later erosions present clinically, the distinction is moot because the wound and underlying hardware are now contaminated with skin flora.
Most DBS hardware infections occur at the pocket site, involving the pulse generator in one-third of cases or the lead extensions. Luckily, intracranial lead infections with possible intracerebral abscess formation are rare. Rates of infection with pulse generator replacement or revision have been reported to be increased by some but not others.
Best Practice
Implantation of DBS electrodes, extensions, and a generator can be performed in one or more stages. The infection risk does not increase with temporary externalized wires. The procedure should be performed after screening the patient for nasal methicillin-resistant Staphylococcus aureus (MRSA) and completing a decolonization, if timely possible. We recommend an antibacterial shower or cleansing the night before surgery, despite a possible lack of effect on SSI rates. All patients receive a dose of parenteral antibiotics within an hour before skin incision, at each stage. We remove hair with a clipper for technical and postoperative hygienic convenience. There is no statistical evidence that hair increases the infection rate, although no study has been specifically done for hardware implantation. Surgical sites should be prepped with an alcohol-based chlorhexidine or iodine solution. Although not proven, reopening a recent incision for wire connection is best avoided to reduce the infection rate. The use of water- based or alcohol-based surgical hand antiseptics does not affect the rate of infections. Double-gloving is done for all procedures. Iodinated-adhesive drapes are used only for stage one procedures to cover the fiducials, which get contaminated during the nonsterile registration. There is otherwise no evidence for the use of adhesive drapes.
Irrigation with bacitracin at 10,000 U/L is used to copiously irrigate the wound before closure. A mixture of 200,000 units of polymyxin B and 40 mg of neomycin diluted in saline 1 : 10 (v/v) is distributed in surgical sites after the deep sutures are tied and the wounds are watertight. No antibiotics are administered after surgery in concordance with the lack of evidence supporting a prolonged postoperative antibiotic administration.
Topical antibiotics are applied to incisions; the risk to benefit ratio is valuable in our opinion, despite the low level of evidence supporting a number needed to treat of 50. Occlusive dressings are placed over the incisions and not removed for at least 24 hours after surgery, although we prefer to keep the dressing on for 2 days. After this period, the principle of “clean and dry” is followed. We advise our patients to cover the incisions with a waterproof dressing while showering for 2 weeks postimplantation and to wait 4 weeks before submerging the surgical sites.
If the infection or erosion occurs over the generator site, often the DBS leads can be salvaged by first dividing the extensions just distal to the connectors, closing that wound completely, isolating that part of the field (usually behind the ear), and then removing the generator and extension lead segment. Cultures are performed at both the retroauricular connector and chest generator sites. If the retroauricular wound shows growth on culture, the DBS leads, skull anchors, and connectors are subsequently removed. If the culture at the retroauricular connector site shows no growth, the patient is treated with 4 to 6 weeks of parenteral antibiotic based on the bacterial sensitivities obtained from the chest wound. The patient is observed and monitored for recurrent infection for a month post cessation of antibiotics. The patient is then placed on a 10-day course of doxycycline 100 mg per oral twice a day starting on the day of reimplantation of new hardware. The same protocol is observed if the entire system is removed, replacing the entire DBS system only after the treatment regimen and a period of observation.
Lead Migration, Lead Fracture, and Electrical Malfunction
As long as hardware is being implanted, some will eventually break or malfunction. This is to be expected because hardware can be subjected to repetitive movements, stretching, and even trauma. A rough estimate would be 8% per lead-year, keeping in mind that most data has been retrieved from older hardware models and that this rate should substantially reduce with technologic advancements. Lead fractures have been estimated around 1.8% per lead. The majority of lead fractures are associated with the implantation of connectors at or below the mastoid, allowing the extensions to track down toward the chest and pull the leads along the way. Lead extensions can also occasionally become tethered (0.12%), leading to a phenomenon described as “bowstringing.”
Electrode migrations are very uncommon now with the advent of reliable skull-anchoring devices. Despite this, reported rates, irrespective of anchoring technology, average around 4.4% per lead. With improvement of skull-anchoring devices, rates of lead migration should plummet. Again, intraoperative imaging is of utmost assistance in confirming lead proper positioning until the lead is secured in place. Rare occurrence of “twiddling” syndrome has been linked to lead migration, mostly in children, and raises the necessity to anchor the pulse generator when there is a possibility of its moving in the pocket.
Electrical malfunctions, mainly short or open circuits, are intrinsic to DBS implantation, ranging between 0.9% and 9.9%. Most of the time, programming can be done around malfunctioning contacts, avoiding revising electrodes or extensions. With sturdier connector and electrode designs, these rates should also decrease over time. Pulse generator malfunctions are now uncommon, and these devices are highly reliable. MRI-compatible models have been designed to be free of magnetic interferences. At most, malfunctions are no more than 1%.
Best Practice
Lead fractures from migration of the lead extensions can be prevented by implanting the connectors posterior and superior to the ear. We implant our DBS systems in two stages, and during the first stage we bring the lead caps over the parietal convexity, above the ear, in the subgaleal space. This means that the DBS lead caps are sitting at the border of the temporalis muscle. During the second stage, the lead extension connectors are implanted above the upper third of the pinna, positioning the connectors above the nuchal fascia attachment to the skull. This prevents downward migration of the extensions.
“Bowstringing” extensions require opening the posterior auricular incision and the pocket incision and readjusting the extensions to release the tethering. Generally, extensions are replaced with new ones, keeping in mind a minimal risk of damaging the DBS leads when manipulating the connectors. Finally, pulse generators should be anchored to the fascia unless the pocket is well established and tight enough to prevent rotation or flipping of the generator. Rechargeable generators should always be anchored to the pocket wall.
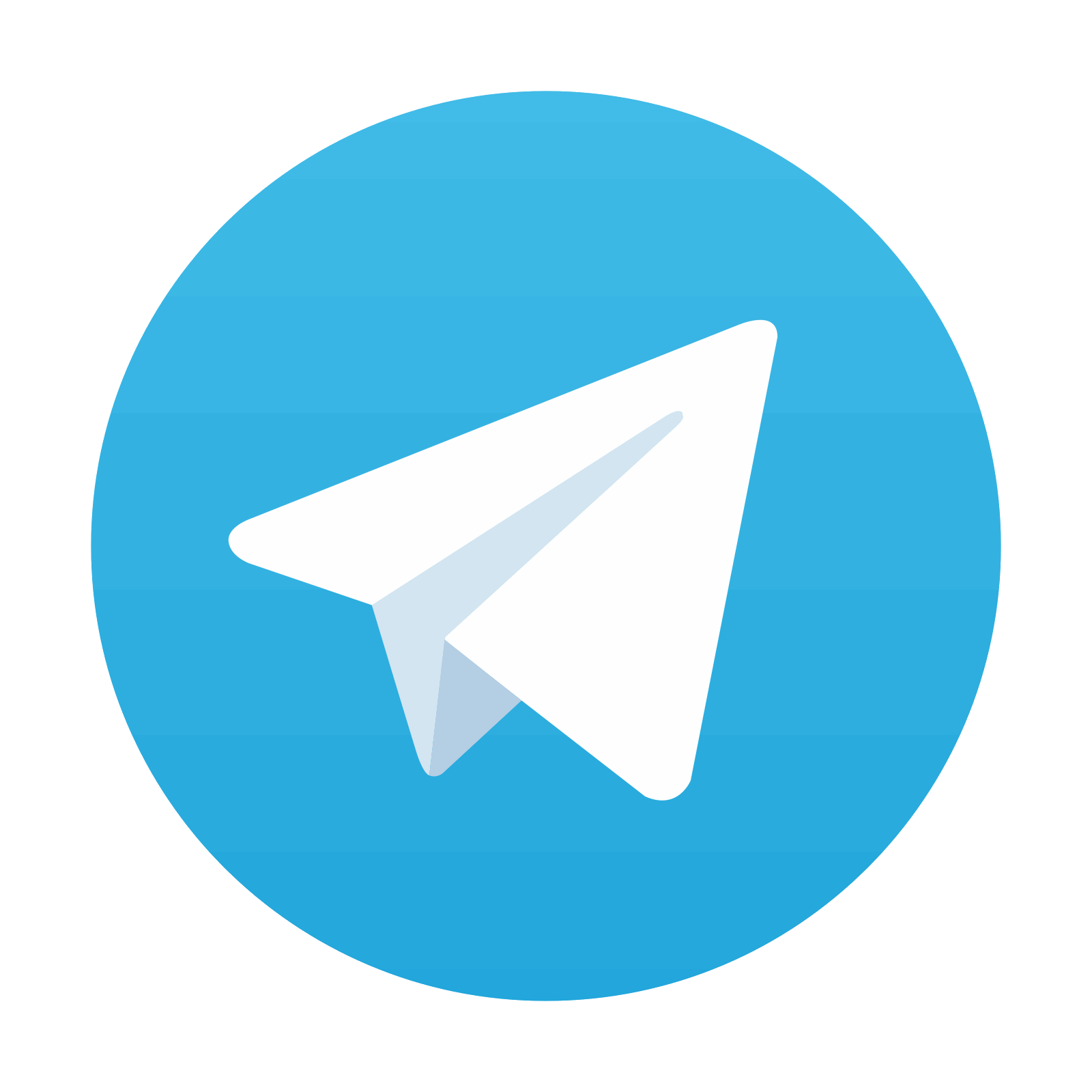
Stay updated, free articles. Join our Telegram channel

Full access? Get Clinical Tree
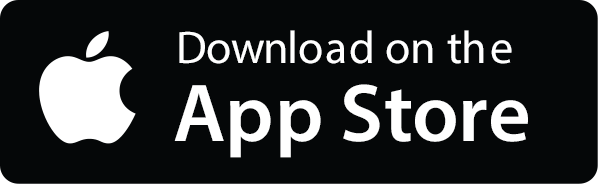
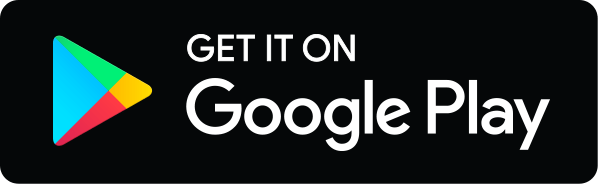