Abstract
Peripheral nerve injuries and tumors are complex to manage, often requiring a multi-disciplinary approach. With an appropriate reconstructive strategy, in the optimal time window, using proper surgical technique, good outcomes can be achieved for patients with peripheral nerve injuries. For nerve sheath tumors, the most important early management is differentiating benign from malignant. This chapter highlights the evaluation and management of peripheral nerve injuries and reviews options for salvage in the case of failure of primary nerve surgery. The chapter also details the evaluation of peripheral nerve tumors, particularly focusing on differentiating benign from malignant and discusses what to do in cases when a malignant tumor is misdiagnosed as benign.
Keywords
brachial plexus injury, nerve transfer, free-functioning muscle transfer, nerve sheath tumor, schwannoma, neurofibroma
Highlights
- •
Prevention of reconstruction failure depends on choosing the appropriate reconstructive strategy for the right time frame and optimizing the surgical technique.
- •
For upper trunk injuries with failure of primary nerve reconstruction, additional options exist including free-functioning muscle transfer, tendon transfers, and shoulder fusion.
- •
For nerve sheath tumors, rapid growth, neurologic deficits, being fixed rather than mobile on palpation, and significant spontaneous pain warrant further evaluation for malignancy.
- •
When a nerve sheath tumor is unexpectedly diagnosed as a malignant nerve sheath tumor or other malignant tumor, a multidisciplinary sarcoma team should be employed to evaluate for metastatic disease and plan for definitive resection with or without chemotherapy and radiation.
Background—Nerve Injuries
Injury to the brachial plexus can occur secondary to penetrating trauma or trauma that induces stretch in the nerves comprising the brachial plexus. Brachial plexus injuries are reported to occur in approximately 1% of polytrauma patients, with motor vehicle accidents being the most common mechanism of injury. Among motor vehicle accidents, motorcycle and snowmobile accidents carry a substantially higher risk than automobile accidents. Supraclavicular brachial plexus injuries are significantly more common than infraclavicular brachial plexus injuries in the polytrauma setting.
Seddon classified nerve injuries into three groups: (1) neurapraxic, (2) axonotmetic, and (3) neurotmetic. Neurapraxic injury is the mildest form; the axon remains intact, as well as the epineurium, perineurium, and endoneurium. Wallerian degeneration does not occur. There is a focal physiologic conduction block with intact conduction distal to the site of injury and proximal to the site of injury but not across the injured segment. Spontaneous recovery is the norm and usually occurs within days or weeks. Axonotmetic injuries are characterized by partial or complete axon disruption with preservation of the epineurium and perineurium. Wallerian degeneration occurs distal to the injury, and after Wallerian degeneration occurs, conduction distal to the injury is lost. Spontaneous recovery can occur. Finally, neurotmetic injuries, the most severe form, are characterized by disruption of the axon and surrounding connective tissue, including the perineurium and epineurium. Wallerian degeneration occurs distal to the site of injury. Spontaneous recovery is not possible.
A common mechanism of injury involves a sudden increase in the angle between the neck and the shoulder, stretching the brachial plexus, particularly the upper brachial plexus. Options for management depend on the segment(s) of the brachial plexus that are injured, the severity of the injury, and the timing of presentation. Potential management options include conservative management allowing for spontaneous recovery; neurolysis, nerve graft repair, and nerve transfer (primary nerve surgery); and other soft tissue or bony reconstruction strategies, including tendon transfer, free muscle transfer, and joint fusion (secondary surgery).
Anatomic Insights
The brachial plexus is comprised of the C5–T1 spinal nerves. The C5 and C6 nerves join to form the upper trunk of the brachial plexus. With injury to the upper brachial plexus, there is loss of shoulder abduction (deltoid and supraspinatus), shoulder external rotation (infraspinatus), forearm supination (biceps), and elbow flexion (biceps, brachialis, and brachioradialis). Reconstructive strategies target restoration of these movements. Other nerves related to the upper brachial plexus include the phrenic, dorsal scapular, and long thoracic nerves. The phrenic nerve receives contributions from C3, C4, and C5 and innervates the diaphragm. The dorsal scapular nerve arises from the proximal aspect of the C5 nerve root to innervate the levator scapulae and rhomboids. The long thoracic nerve receives contributions from C5, C6, and C7, with the contributions arising proximally, ultimately innervating the serratus anterior.
Although the Seddon classification of nerve injuries is important in determining the likelihood of spontaneous recovery, an additional classification system is important for determining both the possibility of spontaneous recovery and the options for nerve reconstruction when necessary. This system dichotomizes nerve injuries based on the anatomic position of the injury relative to the dorsal root ganglion into preganglionic versus postganglionic injuries. Preganglionic injuries generally do not have a chance for spontaneous recovery, and in cases of nerve reconstruction, nerve graft repair is not an option because a viable nerve stump will not be present to graft into. Postganglionic injuries may or may not have a chance of spontaneous recovery, depending on the extent of the injury, but in cases of nerve reconstruction, a viable nerve stump will generally be present, allowing for nerve graft repair as an option.
In the setting of upper brachial plexus injuries, clinical, electrodiagnostic, and imaging features can be clues to pre- versus postganglionic status. Clinically, examination of the rhomboids and serratus anterior is important, since the takeoffs of the nerves or contributions to the nerves that innervate these muscles occur so proximally on C5 and C6. Loss of innervation to the rhomboids and/or serratus anterior suggests a very proximal injury. Although it does not confirm a preganglionic injury, it makes it more likely. Similarly, electromyography (EMG) can be used to determine whether the rhomboids and serratus anterior have been affected by the injury (i.e., presence of fibrillation potentials). An additional clue comes with sensory nerve action potential (SNAP) testing. In cases of postganglionic injury, both motor nerve action potentials and SNAPs are lost, whereas in cases of preganglionic injury, the motor nerve action potential is lost, but the SNAP is preserved. Similarly, an elevated hemidiaphragm on imaging (e.g., inspiration/expiration chest films, ultrasound, computed tomography [CT] scan) suggests involvement of the phrenic nerve. Although not diagnostic of a preganglionic injury, involvement of the phrenic nerve suggests a proximal and severe injury. Cervical radiographs showing spinal fractures are also associated with preganglionic injuries. Both CT and magnetic resonance (MR) myelography can also be used to look for signs of nerve root avulsion (preganglionic injury). The most common signs are the presence of a pseudomeningocele or pseudomeningocele with absent rootlets.
In cases of preganglionic injuries, nerve transfer is the procedure of choice. Because of the improved results with newer types of nerve transfers, nerve transfers are being increasingly used by many surgeons, in lieu of nerve grafting, in select cases of postganglionic injury or in cases where patients present late (i.e., after the typical window for nerve surgery). When performing nerve transfers, surgeons must understand the anatomy of the potential donor nerves to maximize outcomes and minimize donor morbidity. Probably the most common nerve transfers employed for upper brachial plexus injuries include spinal accessory nerve to suprascapular nerve and/or radial nerve triceps branch to axillary nerve for shoulder (C5) targets; and ulnar nerve fascicle to musculocutaneous nerve biceps branch (Oberlin transfer) with or without median nerve fascicle to musculocutaneous nerve brachialis branch for elbow flexion (C6) targets. In this situation, an ideal donor nerve is purely motor with a large number of motor axons, has sufficient length to allow coaptation to the target nerve in a tension-free manner without an intervening graft, has a redundant or expendable function, and has an action that is synergistic to the action of the target nerve.
The spinal accessory nerve comprises approximately 1500 motor axons, innervating both the sternocleidomastoid and trapezius muscles. The spinal accessory nerve emerges from beneath the sternocleidomastoid to run on the anterior surface of the trapezius, innervating the trapezius along its course. Transection of the spinal accessory nerve for use as a donor should occur distal to the first motor branch to the trapezius to allow continued partial innervation of the trapezius and to avoid the morbidity associated with complete denervation of the trapezius. Sufficient length of the spinal accessory nerve can typically be isolated to allow coaptation to the suprascapular nerve in a tension-free manner without an intervening graft. Debate exists as to whether the spinal accessory nerve should be used as a donor in this way or whether the trapezius muscle should be preserved and used instead for tendon transfer.
The radial nerve innervates all three heads of the triceps muscle. The redundant function of the heads of the triceps makes individual nerve branches to the triceps good candidates to be used as donor nerves. Furthermore, the synergistic function of the triceps and the deltoid muscles makes the triceps nerve branches good candidates for nerve transfer, specifically to the axillary nerve. Each of the branches to the three heads has theoretical advantages. The long head of the triceps is mechanically the least important for elbow extension, making use of the nerve branch to the long head theoretically the branch associated with the lowest donor morbidity. The branch to the long head also is the largest and contains the highest number of motor axons. The significant downside is that the length of the branch to the long head is typically short, making obtaining enough length for tension-free coaptation to the axillary nerve difficult. The branch to the medial head of the triceps is longer, making it significantly easier to achieve sufficient length. Care must be taken when isolating the specific triceps branch intended for nerve transfer to avoid damage to the main trunk of the radial nerve and weakening of wrist/finger extension.
A single fascicle of the ulnar nerve can be transferred to the biceps branch of the musculocutaneous nerve ( Fig. 63.1 ). The close proximity of the ulnar nerve to the biceps branch in the upper arm means that only a short length is needed for a tension-free coaptation. The ideal donor nerve fascicle supplies only, or at least predominantly, the flexor carpi ulnaris. By utilizing a fascicle innervating the flexor carpi ulnaris, which is redundantly innervated by multiple fascicles, donor morbidity is minimized (also, other median nerve innervated wrist flexors exist, minimizing donor morbidity). The fascicle innervating predominantly the flexor carpi ulnaris is typically located in the anterior-medial portion of the nerve.

Elbow flexion can be supplemented by reinnervating the brachialis via median nerve fascicle transfer to the brachialis branch of the musculocutaneous nerve. Similar to the ulnar fascicle transfer, the close proximity of the median nerve to the brachialis branch means that only a short length is needed for a direct tension-free repair and that the coaptation sits close to the motor end plate of the brachialis muscle, requiring only a short distance of nerve regeneration. The ideal fascicles for transfer innervate predominantly the flexor digitorum superficialis and flexor carpi radialis. Such a fascicle typically is located in the anterior-medial portion of the nerve. The fascicles ultimately giving rise to the anterior interosseous nerve are to be avoided as donors. These fascicles typically are located in the posterior-medial nerve. Understanding the fascicular topography and performing careful interfascicular dissection are critical to avoiding undue injury to the median nerve.
Although these are likely the most commonly employed nerve transfer strategies with upper brachial plexus injuries, a variety of other donor nerves and nerve transfer strategies have also been described and are available in the surgical armamentarium. Add to these nerve graft repair and secondary reconstructive strategies and there is a wide array of choices. Potential complications include injury to surrounding nerves or donor nerves, vascular injury because the brachial plexus lies in close proximity to major vascular structures, pneumothorax (the brachial plexus lies in close proximity to the apex of the lung, or during intercostal nerve isolation for transfer, if that strategy is employed), chyle leak or chylothorax (particularly on the left side due to the proximity of the thoracic duct), phrenic nerve injury with paralysis of a hemidiaphragm, and failure of the reconstruction. Failure of the reconstruction to achieve satisfactory restoration of function is the most common complication among the list. This complication will be our focus.
Debate continues regarding nerve graft repair versus nerve transfer as the optimal reconstructive strategy when both are options. To employ a nerve graft repair strategy requires a viable nerve stump to graft into. An injured, unhealthy nerve stump is a risk factor for failure. There is no way to completely reliably determine the health of the nerve stump. A combination of visual inspection, intraoperative electrophysiologic testing and, in some cases, intraoperative histologic analysis is used to determine whether a nerve stump is viable. To adequately prepare the nerve stump for grafting, the nerve must be resected back to healthy tissue with a normal fascicular pattern. Grafting into a damaged, unhealthy nerve is a risk factor for failure.
The length of nerve graft has also been associated with outcomes, with longer nerve graft length being associated with poorer outcome. The data, however, are mixed in this regard, with some studies showing no correlation between nerve graft length and outcome. Longer graft lengths may be a red flag, but this remains to be proven conclusively.
From the time of injury, it is a race against time to restore innervation before the muscle and neuromuscular junction are irreparably damaged. Prolonged delay from injury to reconstruction is a red flag for failure. Nerve transfers can be performed in a more delayed fashion than nerve graft repair due to the shorter distance of nerve regeneration required to reach the motor end plate, but prolonged delay, whether nerve graft or transfer is employed, is a risk factor for poor outcome. Although not a hard-and-fast rule, nerve graft repair beyond approximately 6 months after injury and nerve transfer beyond approximately 9 months after injury have progressively more risk of failure the further beyond these time points one gets.
Nerve transfers borrow function from another nerve. The success of this strategy depends on the integrity and health of the donor nerve. For example, the Oberlin nerve transfer borrows a nerve fascicle innervating the flexor carpi ulnaris from the ulnar nerve to reinnervate the biceps branch of the musculocutaneous nerve. If the flexor carpi ulnaris is weak on examination, is this still a viable option? How weak is too weak for the nerve still to be considered a viable donor? These questions remain to be answered. It is clear, however, that injury to the donor nerve is a risk factor for reconstruction failure.
Prevention
Prevention of reconstruction failure depends on choosing the appropriate reconstructive strategy, optimizing surgical technique, and appropriately timing surgical intervention. Time from injury to surgery should be minimized while still allowing sufficient time to determine whether spontaneous recovery will occur. For closed injuries, the optimal time window for surgical decision-making is between 3 and 6 months postinjury. A combination of clinical examination, electrodiagnostics, and imaging should be used to determine the need for surgery. In cases of delayed presentation, nerve transfer strategies, rather than nerve graft repair, should be strongly considered.
The reconstructive strategy should be optimized to lessen the risk of failure. Data suggest that in upper brachial plexus injury, the Oberlin transfer (ulnar fascicle to biceps branch) has better outcomes than any other nerve transfer strategy and also better outcomes compared with nerve graft repair. Double fascicular transfer utilizing a fascicle from the median nerve transferred to the brachialis branch plus the Oberlin nerve transfer, which intuitively would have improved outcomes compared with a single fascicular transfer, has been shown to have outcomes similar to those with single transfer using the Oberlin technique. If the ulnar nerve is partially injured and the flexor carpi ulnaris is weak, consideration should be given to an alternative strategy (though how weak is too weak remains unclear), so as to avoid further injury to the ulnar nerve or suboptimal recovery of elbow flexion.
For reconstruction of shoulder abduction and external rotation when nerve graft repair is possible, a number of potential permutations exist with combinations of nerve graft repair, spinal accessory nerve to suprascapular nerve transfer, radial nerve triceps branch to axillary nerve transfer, and trapezius tendon transfer (which requires not utilizing the spinal accessory nerve as a donor). Two recent systematic analyses suggest that dual nerve transfer yields improved results for shoulder abduction compared with single nerve transfer, nerve graft, or combined nerve transfer and nerve graft. The role of trapezius tendon transfer relative to spinal accessory nerve transfer remains unclear.
When nerve graft repair is utilized, surgical technique is important in preventing or minimizing the risk of failure. Intraoperative electrophysiologic testing with somatosensory motor evoked potentials and motor evoked potentials can be used to confirm that the nerve stump is in continuity with the spinal cord and is viable. Nerve action potentials also can be used to determine a preganglionic versus postganglionic injury and to document regeneration across a postganglionic injury (i.e., a neuroma-in-continuity). The nerve stump must be prepared adequately to receive a graft. The damaged end should be resected back to bleeding, healthy-appearing nerve with normal fascicular architecture. We confirm this visually, though confirmation with histologic analysis is performed at some centers. The nerve graft should be cabled to create a size match between the nerve stump and cabled graft. Each coaptation should be secured with several fine microsutures, often supplemented with fibrin glue. We also wrap the coaptation site with a collagen nerve wrap because there are some data in animal models indicating that this improves outcomes. Finally, the graft length should be minimized while still allowing for a tension-free repair.
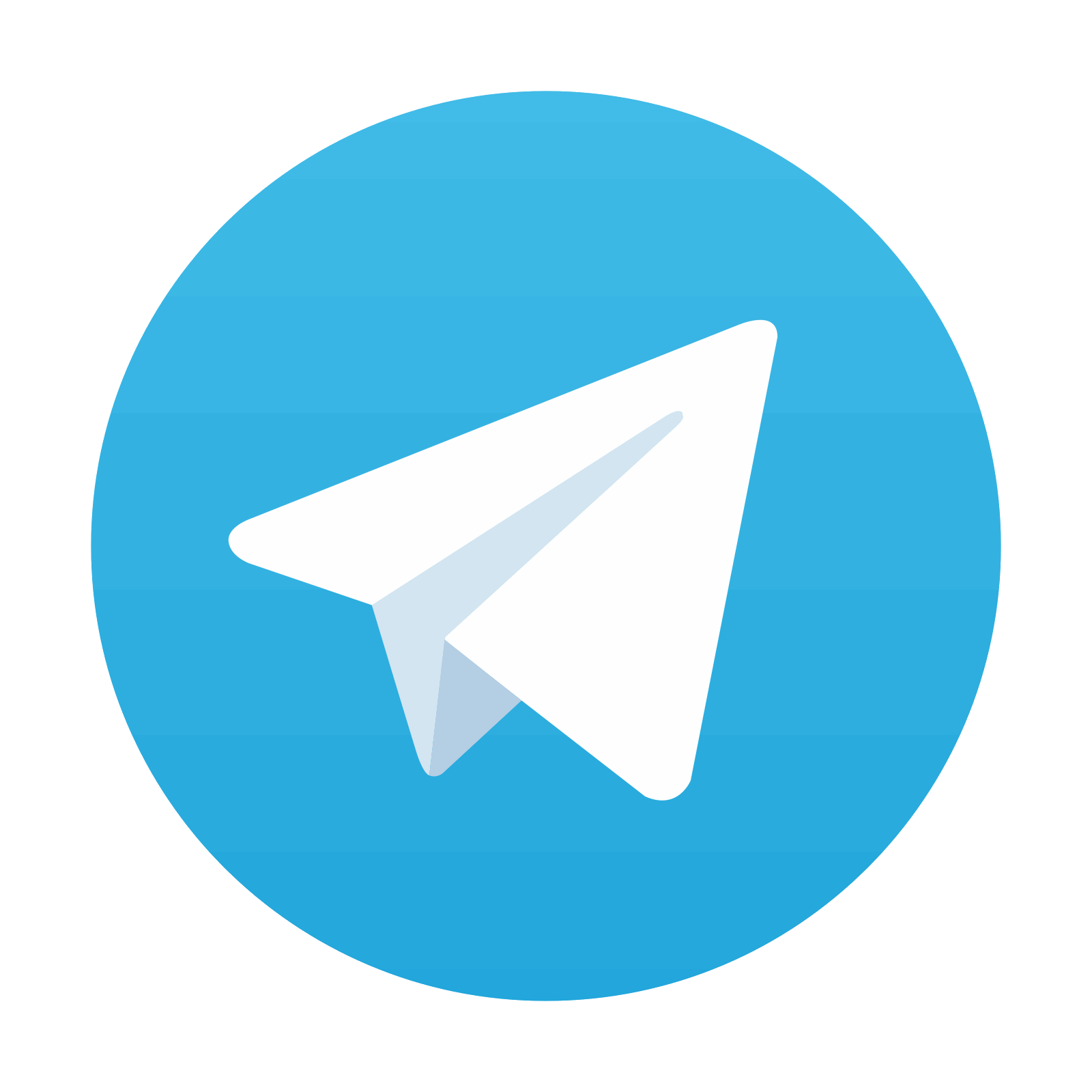
Stay updated, free articles. Join our Telegram channel

Full access? Get Clinical Tree
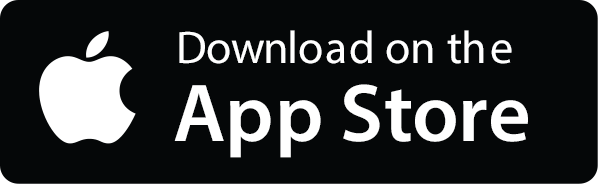
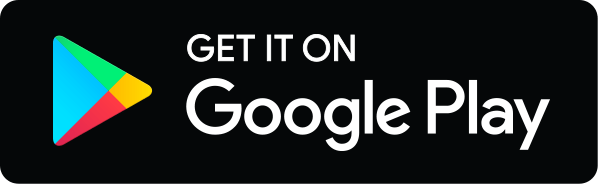