
This type of valve may be of benefit in patients with siphoning and over-drainage problems or in those with a high risk of developing slit-ventricle syndrome. Current data have shown a benefit in improving symptoms of low ICP but no difference in their revision rates compared to other types of valves. In fact, these valves tend to have a smaller orifice, which may act as a site for obstruction.
6.3.2 Differential Pressure Valves
This type of valve utilises a very simple concept – opening and closing pressures. When the ICP is above the valve’s opening pressure, CSF will flow, and vice versa. However, due to the valve’s mechanisms, the closing pressure may be lower than the quoted opening pressure and symptoms of over-drainage may occur without siphoning. A large variety of these valves exist in the market, examples include the Codman® Precision Valve and the Sophysa Pulsar® Valve, and each one is subdivided according to their pressure range (high, medium and low). They can be roughly placed into four groups according to their mechanism of actions: diaphragm valve, ball-in-cone valve, mitre valve and slit valve.
Diaphragm valves are the most common type and include the Sophysa Pulsar® Valve. They consist of a silicon membrane which deflects in response to ICP changes, thus controlling the flow rate. These devices differ from one another in terms of the position of the membrane (proximal or distal to reservoir) and therefore would affect one’s ability to utilise the valve for examination and intrathecal drug delivery.


Ball-in-cone valves include the Codman® Hakim system where a ball sits in the middle of a cone or a spring. The opening pressure is determined by the properties of the spring whilst the flow rate is then determined by the diameter of the outflow aperture, the diameter of the ball and the cone angle. As ICP increases, the ball is displaced away from the cone and therefore flow increases. These valves seem to be less prone to the ageing effects of materials compared to the mitre and slit valves but may be more prone to obstruction (e.g. in post-intraventricular haemorrhage neonates).


The mitre valves include the IntegraTM Mischler Valve which is essentially a system with a direct flow path to resist occlusion and may therefore be useful in those with high CSF protein levels. The main determinants for their control of CSF flow include the size, shape, length and thickness of the silicone leaflets.
Slit valves are made of silicone rubber material with cuts or slits within them [27]. These slits are malleable and will open in response to rising ICP. The number of slits and the stiffness of the membrane will determine the opening pressure and flow rate. An example would be the Sophysa Phoenix system. They can be placed either proximally (e.g. Holter-Hausner® valve) or distally (e.g. Codman® Unishunt) – the more distal the placement, the lower the resistance to flow.


6.3.3 Gravitational Valves
This type of valve has been designed to reduce the siphoning effects of change in patient’s posture by altering the opening pressure, using gravity. They are basically ball-in-cone differential pressure valves but with an additional gravitational unit. This is composed of a ball which would displace into the cone when the patient is upright, thus increasing resistance and reducing flow. This results in the valve having two different opening pressures depending on the patient’s posture [21]. As with any differential pressure valve, they come in a range of different opening pressures and given that this is dependent on the height of the column (see hydrodynamics above), the choice should be based on the height of the patient. Examples of gravitational valves include the IntegraTM H-V valve and the Miethke DualSwitchTM valve.
6.3.4 Adjustable or ‘Programmable’ Valves
These are differential pressure valves which have a mechanism that allows adjustment of the opening pressure by the user externally, but otherwise the design is similar to a normal differential pressure valve as described above. The obvious benefit is the ability to alter the opening pressure according to the patient’s symptoms and hence negating the need for revision surgery. They may therefore be useful in normal pressure hydrocephalus patients to allow a gradual reduction in ventricular size without causing subdural haematomas [15]. However, there are no class I data to compare their efficacy to other types of valves. In addition, due to the magnetic design of these valves, a strong magnetic field such as an MRI may alter the shunt setting. This has been attempted to be overcome, such as in the Polaris and proGav valves [2, 1]
A number of adjustable valves are available in the market, including Codman® Medos valve, Strata® adjustable pressure valve and Miethke proSATM valve [9, 31]. These are, in the main, indicated in the management of idiopathic Normal Pressure Hydrocephalus and have been shown to reduce post-operative over-drainage and subdural formation in these patients [10] However, a surgeon may choose to use these more expensive valves in younger adults with very large ventricles or perhaps in children primarily, as a way of allowing gradual upgrading of valve pressure as the child grows taller [5, 38]. Whether this lowers the risk of long-term over-drainage complications or blockage in the individual child is not yet clear from longitudinal studies [6].


6.4 Catheter Characteristics
It is now well recognised that delayed shunt complications (defined here as greater than 5 years) are often a consequence of catheter failure [45]. The entity of catheter occlusion by in-growing choroid plexus, ependyma and glia is covered elsewhere in this book. Here we are interested in the details of catheter design and material that may dispose it to degrade, calcify and become brittle over time [12]. This may lead to catheter fracture, migration and cause subsequent shunt dysfunction as well as possible visceral injury, depending on the site of the distal catheter [3, 4, 11, 18, 23]. Catheter technology has developed with greater understanding of the resistance associated with the tube itself and how this affects CSF drainage. Tissue response to the catheter itself has changed the materials used in shunt manufacture [13, 14].
6.4.1 Material Properties
Modern shunt catheter tubing is made of plain silicone, and initial concerns about silicone allergy in shunted patients have largely been clinically unfounded [30]. Initial shunt catheters sometimes contained a spiral wire re-enforcement in order to reduce the chance of kinking but were associated with a high rate of abdominal complications. There are many reports of catheter migration and visceral perforation (usually of the rectum or vagina) associated with the Raimondi wired shunt in the literature, so they are not used in modern neurosurgical practice for these reasons [36, 39, 40, 42, 43, 46, 49].
Catheter material tends to degrade and calcify over time. This makes the catheter more brittle and likely to fracture during patient movement. Calcified or degraded catheter tubing can become fixed to subcutaneous tissue. If this fixation takes place before the patient stops growing, there is an increased risk of fracture or migration. It also makes removal of a catheter at revision surgery much more difficult and it is far more likely that shunt products are retained within the patient. This is associated with developing later abdominal complications such as intestinal obstruction and perforation (Fig. 6.1) [48].


Fig. 6.1
Delayed rectal extrusion of a distal shunt catheter in an infant shown on plain radiography caused by colonic erosion without clinical evidence of peritonism or bowel perforation
Calcification in shunt catheters occurs in vivo by dystrophic calcification, which is characterised by a normal concentration of serum calcium but altered cellular metabolism caused by cell death or damage. This type of calcification seen in silicone implants is thought to occur due to the cellular debris that accumulates next to the implant. This could be the result of direct trauma or tissue reaction to the catheter itself. Dystrophic calcification is augmented by the presence of surface irregularities on the catheter caused by catheter degradation and by barium.
Biopolymers deteriorate in four ways: (1) the structure is altered by hydration; (2) covalent bonds of the chains are weakened; (3) these bonds are broken, which reduces the molecular weight of the polymer; and (4) certain soluble fragments are digested by macrophages, which causes host-cell-mediated inflammation, and can accelerate calcification. In the human body, silicones have to resist chemical and mechanical insults for a number of years.
Interestingly, the cerebral parenchyma and peritoneal cavity do not degrade silicone catheters, suggesting that it is the subcutaneous environment that promotes polymer breakdown. However, this cannot be completely the case as ventriculo-atrial catheters have also been shown to degrade with time. Mechanical forces applied during movement can facilitate the breaking down of non-covalent bonds in a silicone polymer, which may explain the differential degradation of catheters along its length. Catheter fracture is often observed at the level of the neck; this is the part of the catheter that is under most dynamic stress during the lifetime of the shunt system and several reports have indicated that the most common site of shunt fracture was 2–4 cm above the clavicle.
The presence of barium coating of shunt catheters is associated with an increased rate of catheter calcification. Both platinum- and barium-coated silicon catheters have been reported to have an early calcification and fracture rate. For these reasons, it is generally recommended that they are no longer used in clinical practice. One may hypothesise that the coating is more likely to form microscopic cracks with time that may cause local micro-cellular damage and hence activate local host inflammation and accelerate dystrophic calcification as described above. The development of plain silicon-coated shunt catheters has increased the longevity of shunt catheters, but they are still susceptible to calcification and subsequent fracture in the long term. Improvements in shunt longevity will be dictated by research in biomaterials that do not interact with the host environment and do not calcify or degrade over time.
The impregnation of catheters with antibiotics (e.g. Bactiseal), or coating with substances that reduce bacterial adhesion (Silverline, or Bioglide), has been developed to reduce the rate of shunt failure from infection and have variable amounts of evidence to support their efficacy. Some surgeons with historically high shunt infection rates have chosen to use these catheters routinely for all patients at considerably increased expense to their department, whereas others with low infection rates may only use them selectively on those patients with specific risk factors (e.g. infants under the age of 6 months, patients with poor skin condition, previously infected patients). These anti-microbial catheters do not completely eliminate the risk of infection in such patients, but provide some defence against the more common pathogens causing shunt infections. A UK multi-centre randomised trial (BASICS) is underway to assess the efficacy of these different products [7].
6.4.2 Physical Properties and Interplay with the Valve Complex
As previously described, a variety of valves are commercially available, which differ not only in the way they regulate CSF dynamics but also in the way they are manufactured. The profile of the valve and the mechanism by which it connects to the catheter is of relevance to late mechanical complications. A low-profile tubular valve in the catheter line, such as the Hakim valve (Codman) is often chosen in paediatric practice to minimise tension in the overlying thin skin, but has a tendency to migrate downwards because it becomes pulled by a fixed subcutaneous calcified distal catheter as the child grows older. By comparison, the round-bodied valves (Orbis-Sigma), or a flushing burr-hole valve cannot migrate distally with traction caused by growth. In these systems, disconnection or fracture of the distal catheter is more likely to result from the traction applied to the aging degraded material. Rarely, a low-profile tubular valve system can migrate proximally and sometimes fully including the valve (Fig. 6.2).


Fig. 6.2
Migration of whole of a low-profile shunt system upwards into grossly enlarged cerebral ventricles. Most migrations of low-profile systems occur distally into the peritoneal cavity
Physical design of shunt systems will determine how frequently catheters become disconnected and displaced. Unitised systems were introduced in an attempt to lower the risk of disconnections by avoiding the need to attach the tubing to connectors and fix it with a silk or nylon ligature. However, they are more difficult to revise than systems with separate components and overall the longevity of the shunt has not been shown to be any better for these systems. Some type of valves have connectors that need considerable effort and manipulation to attach a catheter securely (‘fiddly’) and would seem more likely to be associated with complications such as disconnections or leakage from iatrogenic holes in the catheter. A surgeon should become familiar with the valve shape and characteristic of the connectors to lower the risk of these types of mechanical complications.
The outflow mechanism from a distal catheter influences shunt function. The presence of drainage slits (not valves) at the end of the distal catheters is associated with a higher incidence of distal shunt failure, and in our practice these are always removed prior to shunting into the peritoneum or pleura [17]. Catheter length has an impact on shunt function [16]. As described above the flow is dependant on resistance – as defined by Poiseuille’s law. The resistance will increase as the length of the catheter increases. Shorter catheters will have a higher flow rate and will, therefore, more likely over-drain. Conversely, very long distal catheters occasionally under-drain in premature babies. This has an obvious implication when shunting very small children. This will be discussed further below.
6.5 Patient Factors
The choice of the most appropriate shunt components for an individual patient can become a complicated science and a full understanding of the aforementioned mechanical characteristics is important in order to minimise complications. Obviously, the various patient characteristics including the underlying reason for the need for shunting are vital in the decision-making process too. These are covered in more detail in the separate chapters in this book and a brief description will be given here.
The underlying diagnosis often determines an individual’s CSF dynamics, as does the patient’s age. It is well documented that CSF production declines with age whilst the resistance to CSF outflow increases with age [20]. This means that older patients are more likely to suffer from low-pressure symptoms secondary to shunt over-drainage [32, 37]. This effect is further amplified by the increased siphoning effect of a long distal catheter, compared to a shorter catheter in a child or baby. Therefore, the treating physician may consider avoiding low-pressure valves in older patients (e.g. those with normal-pressure hydrocephalus, NPH), or in the case of a programmable shunt, to start at a higher setting and gradually reduce it according to symptoms [51]. This approach will also allow for the increased risk of subdural haematoma secondary to over-drainage in the setting of an atrophic brain with reduced compliance [24, 34, 44]. The treating physician may also consider the use of an anti-siphon adjunct which may either be built into the valve (as in the case of a gravitational valve) or as an addition (e.g. Delta chamber, ‘shunt assistant’ or SiphonGuard) [25, 29, 35, 41, 50].
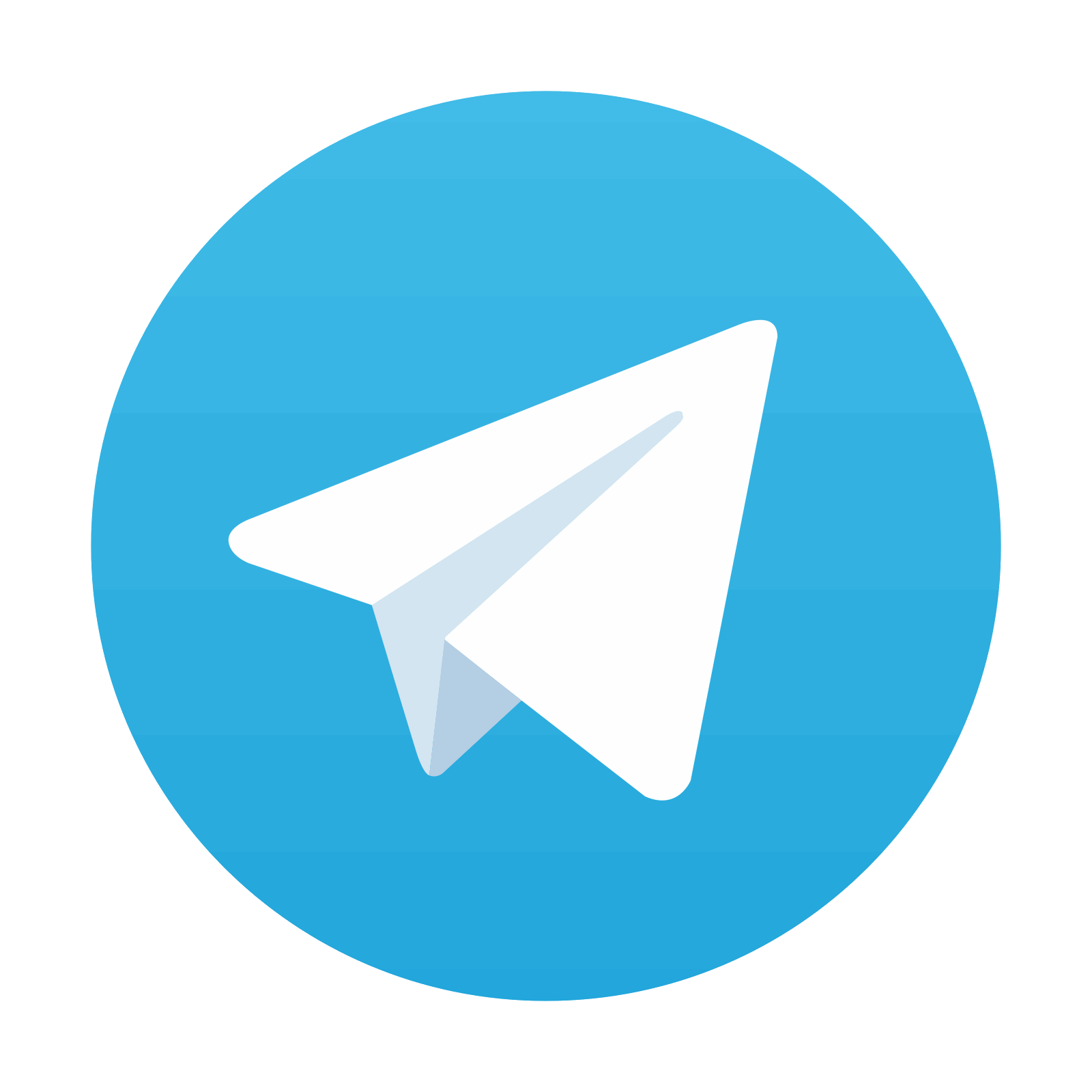
Stay updated, free articles. Join our Telegram channel

Full access? Get Clinical Tree
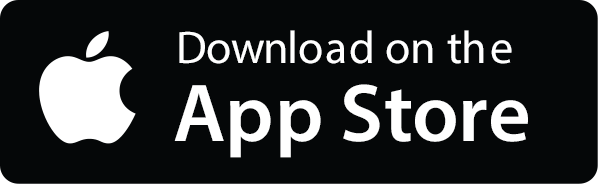
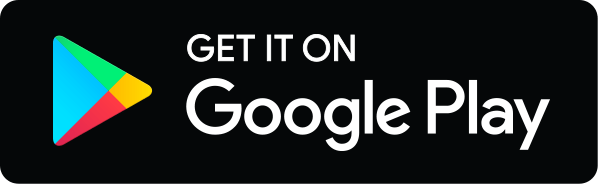