Abstract
Congenital heart disease (CHD) is the most common malformation in humans. The psychomotor development of children with CHD has been shown to be delayed in many aspects throughout adulthood. Many of these delays in brain maturation are evident as early as in the fetal period and often result in brain injury and developmental delays consistent with children born prematurely. Anatomic abnormalities in the most severe CHD disorders, transposition of the great arteries and hypoplastic left heart syndrome, require early surgical interventions to improve the cardiac function, thus subsequently improving blood flow to the brain. Despite improvements in intraoperative monitoring and postoperative care, stroke, white matter injury, cerebral sinovenous thrombosis, subdural hemorrhage, and symptomatic seizures associated with acute brain injury remain common. With increased use of neuroimaging in infants with CHD, we are gaining a greater understanding of the factors that contribute to the developmental impairments seen later in childhood. Furthermore, advanced neuroimaging techniques with diffusion tensor imaging, functional magnetic resonance imaging, and magnetic resonance spectroscopic imaging provide avenues for future investigation of the brain in children with CHD. Although many factors contribute to the outcomes of children with CHD, we continue to search for more neuroprotective and neural recovery treatments that improve the lifelong functioning of these children.
Keywords
advanced MRI technique, amplitude-integrated electroencephalogram, brain development, brain injury, congenital heart disease, continuous electroencephalogram, diffusion tensor imaging, dysmaturation, fetus, functional magnetic resonance imaging, hypoplastic left heart syndrome, infant, long-term outcome, magnetic resonance imaging, magnetic resonance spectroscopy, near-infrared spectroscopy, neonate, neurodevelopment, neuromonitoring, neuroprotection, newborn, sinous venous thrombosis, stroke, subdural hemorrhage, transposition of the great vessels, white matter injury
Fetal Brain Injury and Maturation
In the fetus with CHD, brain maturation at term appears similar to that of preterm infants, as shown by the frequency of white matter injury (WMI) and white matter maturational delays. Several theories have been proposed to explain this interesting feature of infants with CHD, with particular attention paid to blood flow measurements and brain oxygenation. In a study comparing fetal MRIs of confirmed CHD fetuses with controls, CHD fetuses had reduced arterial oxygen saturation (Sa o 2 ) in the aorta without difference in cerebral blood flow. This was found to be associated in a reduced cerebral oxygen consumption of 32% and a 13% overall reduction in brain volume. Fetuses with single-ventricle physiology had a greater reduction in Sa o 2 than those with biventricular physiology. Reductions in head circumference in fetuses with CHD were observed with lower cerebral-to-placental resistance ratios as measured by Doppler ultrasound, suggesting that fetuses with CHD have potentially decreased cerebrovascular resistance compared with controls. This appears to have a global effect on brain growth, with total brain volumes, gray matter, and subcortical brain volumes all being affected. These effects are most notable in the third trimester of the growing fetus with slower brain volume growth and lower N -acetylaspartate (NAA)-to-choline ratios on MR spectroscopy reflecting a white matter maturation delay. Routine ultrasonography of fetuses with CHD can identify brain volume and abdominal circumference growth abnormalities that are independent indicators of delayed brain growth and development. Identification of reduced cerebral fissure depths in fetuses with CHD has been shown to be correlated with lower neurodevelopmental scores at 6 months of age. Fetuses with hypoplastic left heart syndrome (HLHS), transposition of the great arteries (TGA), hypoplastic aortic arch, and other congenital abnormalities resulting in lower placental blood delivery to the brain are at greatest risk of decreased brain maturation and growth delays evident on fetal MRI.
Fetal MRI investigation of the fetus with CHD has revealed that subsequent brain abnormalities are commonly found ( Table 15.1 ), whether as a result of underlying genetic syndromes, in utero events, or a result of volume loss from chronic hypoxia. The most common brain abnormality on fetal MRI is abnormal width of the cerebrospinal fluid spaces identified in 43% of affected patients, followed by malformations in 33%, and acquired lesions in 24%. These findings support the significance of the multifactorial determinants of development of the brain in the fetus with CHD and the role of fetal MR in assessing the neurodevelopmental prognosis preoperatively. The investigation of the fetus with CHD with MRI and Doppler ultrasound is of particular importance to the cardiologist in determining the ideal early hemodynamic and surgical approach, yet is equally important to the neonatologist and pediatrician in optimizing brain development.
Study: Author (Year) | Study Design | Neuroimaging Modality | Brain Abnormalities |
---|---|---|---|
Ruiz et al. (2017) | Prospective cohort CHD (n = 119) | Fetal ultrasound | Smaller biparietal diameter z score (−1.32) and head circumference z -score (−0.79), which remained small throughout gestation |
Sun et al. (2015) | Case-control CHD (30/60) | Fetal MRI | Smaller total brain volume ( P < .001) with reduced cerebral oxygen consumption ( P < .001) |
Zeng et al. (2015) | Case-control CHD (73/241) | 3D Fetal ultrasound | Smaller total brain volumes in CHD from 28 weeks onwards ( P < .05), largest in the frontal lobes. The differences were largest in the HLHS, aortic hypoplasia, and TGA populations. |
Andescavage et al. (2015) | Case-control fetal CHD (41/135) | Fetal MRI | Smaller total brain and cerebral volumes in CHD group ( P < .001), with larger brainstem volumes ( P < .001). |
Schellen et al. (2015) | Case-control tetralogy of Fallot (24/48) | Fetal MRI | Smaller total brain ( P < .001), gray matter ( P = .003), and subcortical brain ( P = 0.001) volumes with increased external CSF spaces ( P < .001) in TOF cases |
Masoller et al. (2016) | Case-control CHD (58/116) | Fetal MRI, MRSI, and fetoplacental Doppler ultrasound | Lower biparietal diameter, head circumference and cerebral blood flow (all P < .05). Lower NAA/choline values in basal ganglia and frontal lobe ( P < .05) Lower brain volumes in CHD infants ( P < .05) |
Brossard-Racine et al. (2014) | Case-control fetal CHD (144/338) | Fetal MRI | Higher risk of brain abnormalities (23% vs. 1.5% in controls, P < .001). Unilateral ventriculomegaly and increased extraaxial spaces most common abnormalities. |
Al-Nafasi et al. (2013) | Case-control fetal CHD (22/34) | Fetal MRI | Lower ventricular output in CHD fetuses ( P < .01). CHD fetuses had lower brain weights. |
Mlczoch et al. (2013) | Prospective cohort CHD (n = 53) | Fetal MRI | Congenital brain disease 39% |
Clouchoux et al. (2012) | Case-control fetal HLHS (18/48) | Fetal MRI | Progressively lower subcortical gray ( P < .05) and white matter ( P < .001) volumes in third trimester. Delays in cortical gyrification ( P < .001) |
Berman et al. (2011) | Case-control fetal CHD (3/31) | Fetal MRI, DWI | ADC values were higher in CHD fetuses in the thalamus ( P < .05) with strong trend in the parietal white matter ( P = .071) |
Limperopoulos et al. (2010) | Case-control CHD (55/105) | Fetal MRI, MRSI | Total brain volumes lower ( P < .001) NAA/choline lower in CHD infants ( P < .001) |
Prenatal Diagnosis
Rates of prenatal detection of major CHD vary considerably across centers. The best quoted European rate is 47%. However, better training in fetal echocardiography may help improve clinicians’ diagnostic skills. Prenatal diagnosis of TGA and HLHS is associated with decreased perioperative morbidity and mortality. This positive impact on the development of the brain likely is a result of earlier prostaglandin treatment and earlier monitoring of the hemodynamic state of newborns with CHD. Prenatally diagnosed infants are more likely to be transported while receiving prostaglandin E 1 , will have a more physiologic pH on admission to a tertiary care center, and have a lower early mortality. Prenatal diagnosis also results in less WMI and/or stroke and allows for better white and grey matter maturation as evidenced by advanced MRI techniques. There is also a reduced likelihood of seizures following prenatal diagnosis. These observations support the importance of prenatal CHD screening and early prostaglandin treatment in the care of the newborn with CHD.
Postnatal Diagnosis
Delayed or missed diagnosis of critical CHD accounts for 0.4 to 2.0 deaths per 10,000 live births in a U.K. series. These infants typically present with serious and life-threatening symptoms, such as cyanosis, tachycardia, pulmonary edema, and cardiogenic shock. The timing of presentation depends on the type of cardiac malformation and its dependence on a patent ductus arteriosus to maintain oxygenated circulation to the brain and other major organs. Before pulse oximetry screening was standard practice in neonatology, 30% of infants with CHD were undiagnosed at the time of discharge from the nursery, especially if they appeared normal physically during their first few days of life. In these undiagnosed newborns, the risk of mortality reaches 30%.
Malformation Grouping
Severe congenital cardiac malformations are typically categorized as either single-ventricle or biventricular lesions, which can be associated with or without aortic arch obstructions such as coarctation, atresia, or stenosis. Any of these lesions can be further complicated by intracardiac (e.g., atrial septal defect or ventricular septal defect) or extracardiac shunts (e.g., patent ductus arteriosus). The presence of shunts, malformed valves, or obstructed blood flow may cause reduced oxygen delivery owing to the mixing of venous and arterial blood. Two common forms of CHD, accounting for more than 80% of surgeries required during the first week of life, are TGA and HLHS.
CHD can be caused by a number of factors, ranging from inherited or spontaneous genetic mutations to environmental factors. The most common genetic syndromes associated with CHD include Down syndrome (trisomy 21), Edward syndrome (trisomy 18), Patau syndrome (trisomy 13), DiGeorge syndrome (22q11.2 deletion), Williams syndrome, and Turner syndrome. Antenatal exposure to certain infections (e.g., rubella), teratogens (e.g., retinoic acid, phenytoin, lithium), maternal diseases (e.g., diabetes mellitus, systemic lupus erythematosus, phenylketonuria), and air pollution are also considered significant contributors.
Transposition of Great Vessels
TGA ( Fig. 15.1 ) results from a ventriculoarterial discordance when the aorta arises from the right ventricle and the pulmonary artery from the left ventricle. Dextro-looped TGA (D-TGA) is the most common type and is characterized by the right ventricle being positioned to the right of the left ventricle and the origin of the aorta being anterior and rightward to the origin of the pulmonary artery. This malformation leads to two parallel circulatory systems and causes the affected infant to be cyanotic. One of these systems carries deoxygenated venous blood from the body to the right atrium and then returns it to the systemic circulation through the right ventricle and aorta. The other system brings oxygenated venous blood from the lungs to the left atrium and sends it back to the lungs via the left ventricle and pulmonary artery. D-TGA can be associated with other cardiac abnormalities, such as ventricular septal defects, left ventricular outflow tract obstruction, mitral and tricuspid valve abnormalities, and coronary artery variations. In this situation, the TGA defect is said to be complex. D-TGA can be difficult to diagnose antenatally with ultrasound. Postnatally, affected infants can present with cyanosis, tachypnea, heart murmurs, and diminished femoral pulses. The severity of symptoms is inversely related to the degree of mixing between the two parallel circulations and the presence of other cardiac anomalies.

The initial management of D-TGA is to stabilize the infant’s condition until the corrective surgery is performed. This stabilization first consists of a continuous intravenous prostaglandin E 1 infusion to maintain the patency of the ductus arteriosus to optimize the intercirculatory mixing between the two systems. Another procedure that is used to promote mixing of the two parallel circulations is balloon atrial septostomy (BAS). In this intervention, during cardiac catheterization a balloon is pulled vigorously across the atrial septum through the foramen ovale or an existing defect, leading to increased atrial mixing. This procedure dramatically improves the survival of infants with D-TGA and has recently been performed in a series of fetuses. The arterial switch, optimally done during the first week of life, is now the standard corrective procedure. An alternative surgical approach, called the Rastelli procedure, is preferred in neonates with significant pulmonary stenosis.
Levo-looped TGA (L-TGA) is much rarer, accounting for less than 1% of CHD. L-TGA is best described as congenitally corrected transposition of great arteries (CCTGA) in which there are discordant atrioventricular and ventriculo-arterial connections. It is due to abnormal leftward looping of the primitive heart. In this cardiac malformation, the heart is physiologically corrected such that the systemic deoxygenated venous blood returns to the pulmonary circulation and oxgenated pulmonary venous blood returns to the systemic circulation. In more than 90% of cases, L-TGA is associated with other cardiac abnormalities such as ventricular septal defect, pulmonary outflow tract obstruction, or tricuspid or mitral valve abnormalities. Even if isolated, L-TGA is associated with systemic ventricular failure in the long term.
Hypoplastic Left Heart Syndrome
HLHS (see Fig. 15.1 ) accounts for 2% to 3% of all CHD and is characterized by an underdevelopment of the left heart with normally connected great vessels, leaving the sole right ventricle to perfuse both the pulmonary and systemic circulations. In this syndrome, the left ventricle is significantly hypoplastic and can be associated either with atresia, stenosis, and hypoplasia of the aortic or mitral valves; with hypoplasia of the ascending aorta and arch; or both. Survival is dependent on ductus arteriosus patency to ensure adequate mixing of oxygenated and deoxygenated blood. Affected infants are relatively asymptomatic initially; however, when the ductus arteriosus closes and the pulmonary vascular resistances decrease, as expected shortly after birth, these infants rapidly go into cardiogenic shock and respiratory insufficiency. Infants with a restrictive or absent atrium septal defect at birth do not benefit from this “honeymoon period” and present with severe cyanosis and respiratory distress at birth because of inadequate interatrial blood mixing. Fortunately, prenatal diagnosis with routine obstetrical ultrasound is often possible, usually between 18 and 24 weeks’ gestation.
As with TGA, the initial management of infants with HLHS is focused on providing sufficient mixing of oxygenated and deoxygenated blood with the use of continuous intravenous prostaglandin E 1 , which maintains the ductus arteriosus patent and allows vital flow from the right ventricle to the systemic circulation, as well as BAS. The surgical palliative repair typically consists of a three-staged approach, with initial surgery performed in the neonatal period (Norwood procedure), then at 3 to 6 months of age (bidirectional Glenn procedure), and at 18 to 30 months of age (Fontan procedure). In the Norwood procedure, a neoaorta is created by using the proximal pulmonary artery and homograft material, then connected to the native ascending aorta. A source of pulmonary blood flow is established by connecting the innominate artery with the proximal right pulmonary artery via a Gore-Tex shunt L-TGA is best described as congenitally corrected transposition of great arteries (CCTGA) in which there are discordant atrioventricular and ventriculo-arterial connections, in a procedure known as a modified Blalock-Taussig shunt. An alternative to BT shunt is the use of a right ventricle to pulmonary artery shunt. When the child reaches 3 to 6 months of age, pulmonary vascular resistance has dropped sufficiently to allow substitution of the arterial shunt to the pulmonary circulation with a venous shunt, thus unloading the ventricle. During the bidirectional Glenn procedure (or cavopulmonary shunt), the original shunt is removed and the superior vena cava is anastomosed end-to-side to the right pulmonary artery. As the child grows further, the Fontan procedure is performed, usually between 2 and 3 years of life. In this third stage, the inferior vena cava is connected to the pulmonary arteries, allowing the entire systemic venous return to flow passively to the lungs.
Brain Injury and Brain Maturation
Table 15.2 summarizes a selected list of studies associating CHD and brain abnormalities in neonates. Fig. 15.2 shows examples of brain injuries found in infants with CHD.
Study: First Author (Year) | Study Design | Neuroimaging Modality | Risk Factors | Brain Abnormalities |
---|---|---|---|---|
Fogel et al. (2017) | Prospective cohort SVP (n = 168) | MRI | Presurgical, bidirectional Glenn (BDG), Fontan procedures | WMI and PVL most common in BDG group; more WMI and brain atrophy seen postoperatively than preoperatively |
Knirsch et al. (2016) | Prospective cross-sectional SVP (n = 47) | MRI | Fontan procedure | Abnormal MRI in 36% Enlarged CSF spaces, small gray, and white matter injuries were seen; half of the individuals had combined lesions. |
Birca et al. (2016) | Prospective cohort CHD (n = 33) | MRI, DTI cEEG | — | WMI and stroke in 25% Brain injury and dysmaturation were both associated with increased high-frequency EEG connectivity and greater discontinuity |
Peyvandi et al. (2016) | Prospective cohort TGA and SVP (n = 153) | MRI, DTI | Postnatal diagnosis of CHD | Brain injury rates higher in postnatal diagnosis ( P = .003) Faster white matter ( P = .04) and gray matter development ( P = .02) in infants with prenatal diagnosis of CHD |
Knirsch et al. (2016) | Prospective cohort SVP (n = 47) | MRI | — | Abnormal MRI in 36% |
Von Rhein et al. (2015) | Case-control Surgical CHD (19/38) | MRI | — | Reduced regional (8%-28%) and total (21%) brain volumes ( P < .001) |
Naim et al. (2015) | Retrospective cohort Surgical CHD patients (n = 161) | cEEG | — | Electrographic seizures in 8% (85% subclinical), beginning at a median of 20 hours after return to ICU. Higher mortality in those with seizures ( P < .001) |
Kelly et al. (2014) | Prospective cohort Surgical CHD (n = 152) | MRI | — | Subdural hemorrhage 43% |
Bertholdt et al. (2014) | Case-control Surgical CHD with CPB (30/50) | MRI | — | WMI and stroke 23% vs. 0% in controls Neuromotor scores 15 days postoperatively median 2.5 (95% CI 0–7) |
Glass et al. (2011) | Prospective cohort Surgical CHD | MRI | Acquired infection (23/127) | No significant increase in WMI was seen with acquired infection |
Block et al. (2010) | Prospective cohort TGA and SVP (n = 92) | MRI | BAS ( P = .003) Lowest arterial oxygen saturation ( P = .007) | WMI and stroke (preoperative) 43% |
Ter Horst et al. (2010) | Prospective cohort Cyanotic and acyanotic CHD post-PGE (n = 62) | aEEG | — | Abnormal background in 45% of infants, severe in 14%. Electrographic seizure activity in 19%, more commonly in acyanotic CHD ( P = .039) |
Shadeed et al. (2011) | Case-control Cyanotic CHD (38/76) | MRSI, DTI | — | Poor brain development on MRSI ( P < .001) and DTI ( P < .001) |
Andropoulos et al. (2010) | Prospective cohort Surgical CHD with CPB | MRI | Single-ventricle physiology (32/68) | Increased WMI and stroke postoperatively Single-ventricle ( P = .02) |
Beca et al. (2009) | Prospective cohort TGA | MRI | BAS (33/44) | WMI and stroke Both NS |
Miller et al. (2007) | Case-control TGA and SVP (41/57) | MRI, DTI, MRSI | — | WMI and stroke (32% CHD vs. 0% controls) Poor brain development on MRSI ( P < .001), DTI ( P < .001) |
McQuillen et al. (2006) | Prospective cohort TGA | MRI | BAS (19/29) | WMI, stroke, and IVH P = .001 (41%–85% risk difference) |
Gaynor et al. (2005) | Prospective cohort Surgical CHD patients (n = 178) | cEEG | Duration of DHCA | Seizures were identified in 11%, with higher likelihood in increased duration of DHCA ( P = .001) |
Tavani et al. (2003) | Prospective cohort Surgical CHD (n = 24) | MRI | — | Intracranial hemorrhage Preoperatively 62%; postoperatively 88% with increased size in 43% |
Mahle et al. (2002) | Prospective cohort Surgical CHD (n = 24) | MRI, MRSI | — | WMI and stroke: 24% preoperatively; 67% new lesions postoperatively Elevated lactate preoperatively 53% ( P < .02) Atrophy late post-operatively 12% |

Cranial Ultrasound Versus MRI
Cranial ultrasound in the neonate is a low-cost and noninvasive bedside procedure that provides an assessment of cerebral volumes and ventricle size and can often identify large hemorrhages, infarcts, and cystic WMI. Advanced ultrasound with Doppler measurements is also informative of the flow in the largest venous sinuses. Unfortunately, the reliability of cranial ultrasound for the detection of ischemic lesions is poor, with up to one-third of infarcts being missed. MRI is increasingly recognized as an important addition to the early investigation of neonates with CHD, given the high burden of radiation associated with computed tomography (CT) and the poor reliability of cranial ultrasound. In the investigation of the infant with CHD, it is important to recognize that the majority of injuries are clinically silent and can be present in well-appearing infants. MR diffusion-weighted imaging (DWI), in combination with T1- and T2-weighted imaging, is an early and sensitive test for brain ischemia and acute injury. Owing to the postinjury pseudonormalization of the DWI signal beyond 5 to 7 days in the neonate, MR DWI is best performed within the first week of life to assess for silent infarcts. Appropriate investigation of the neonate with CHD is necessary to aid determine the medical treatments, neurodevelopmental prognosis, and subsequent follow-up procedures for those at greatest risk of developmental deficits.
Cerebral Ischemia and Stroke
Up to 40% of infants with CHD will have preoperative brain injuries, with an acute cerebral infarct or stroke the most predominant early injury types. Neonates with cyanotic CHD have been shown to have ischemic lesions in up to 60% of cases. In the preoperative period, low oxygen saturation is associated with increased risk of stroke and WMI. Several groups have speculated that BAS is a cause of acute infarcts and WMI in TGA, showing an increased incidence of embolic strokes in patients treated with BAS; however, this remains controversial. As clinically silent infarcts and WMI are common in CHD and have a low risk of progressing with surgery, in most cases medically necessary surgical procedures should not be delayed in the event of brain injury findings.
White Matter Injury
WMI, the predominant injury of the premature neonate, is a well-recognized complication of CHD in the term neonate. Infants with CHD are particularly prone to WMI as a result of fluctuations in the cerebral blood flow both preoperatively and postoperatively. Although stroke is the most common preoperative complication, WMI is the most common lesion acquired after surgery, with the greatest risk in neonates with single-ventricle physiology and aortic arch obstruction. Intraoperatively, during bidirectional Glenn and Fontan procedures it has been shown that higher levels of cerebral blood flow are associated with reduced risk of WMI, suggesting the ischemic nature of this complication. The timing of the injury appears most frequently in the neonatal period, as 1- to 3-month-old infants have a significantly reduced risk of postoperative WMI (4% compared with 54% in neonates). This may reflect the relative immaturity of the cerebral autoregulation systems in the brain of the infant with CHD, much like that of the preterm neonate. In those neonates with known preoperative WMI, worsening of white matter lesions was identified in 19%, with new lesions occurring in 48%. In neonates with follow-up beyond 3 months, MRI scans will reveal that many of the existing lesions have resolved though nearly 30% of patients show new lesions. Similar to cohorts of preterm infants, the incidence of WMI in neonates with CHD appears influenced by nosocomial infections the incidence of WMI in neonates with CHD appears influenced by nosocomial infections. In the assessment of WMI and stroke it is important to remember that their pathophysiologies and subsequent outcomes are shared, and optimizing oxygenation and hemodynamic monitoring provides the best neuroprotection.
Cerebral Sinovenous Thrombosis
Cerebral sinovenous thrombosis (CSVT), a serious hemostatic complication in the ill neonate, is a common finding in autopsies of infants with cyanotic CHD. Yet in a study of several European pediatric registries, patients with CHD represented only 4% of the cases with confirmed CSVT. In an adult study, a greater incidence of thrombosis and bleeding was found in subjects with hypoxemia compared with a nonhypoxemic group (12% vs. 2% and 14% vs. 2%, respectively). The etiology of these abnormalities in hemostasis remains incompletely understood, although chronic endothelial damage, increased blood volume and viscosity, low pulmonary artery velocity, biventricular dysfunction, and chronic right-to-left shunting all likely contribute. The investigation for suspected CSVT should include either unenhanced CT and/or MRI T1- and T2-weighted imaging, in addition to venography (CT venography or MR venography) in investigating for clots within the venous sinuses as well as parenchymal injury with infarction or hemorrhage. An experienced neuroradiologist familiar with neonatal vascular imaging should be involved because of the difficult interpretation of the imaging. In a study comparing CSVT and arterial infarct presentation, parietal involvement and lack of caudate infarction were more suggestive of CSVT.
Subdural Hemorrhage
Serial MRI assessments of neurologically asymptomatic infants with CHD both preoperatively and postoperatively revealed that 43% had subdural hemorrhage (SDH) on at least a single scan, with many infants having more than one focus of hemorrhage. Infants with CHD born by vaginal delivery had a higher incidence compared with those delivered by cesarean section (50% vs. 27%, respectively). These rates are altogether higher than in infants without CHD, normally ranging from 6% to 26% and are highest with assisted vaginal delivery. In neonates with CHD, 61% of SDH were small (≤2 mm deep), and only 10% were 5 mm or more deep; the locations were supratentorial in 43% and infratentorial in 57%. There was no significant difference in the location of the hemorrhages between infants with CHD and those without CHD. In monitoring SDH lesions over time, 24% of foci resolved within the first 2 to 4 weeks and 95% resolved by 3 months of age, with persistent hemorrhages generally observed in the posterior fossa. Postoperatively 9% of infants with CHD showed new foci of hemorrhage; increased size of SDH was observed in 48% in one study. The prevalence of recent preoperative SDH in neonates with chronic hypoxia did not differ significantly from nonhypoxic neonates. Although SDH appears to occur more frequently at birth and postoperatively in infants with CHD, SDHs are generally asymptomatic and resolve over time without treatment. The impact of SDH on the neurodevelopmental outcome of the infant with CHD remains unknown.
Brain Dysmaturation
Brain development during the fetal period requires a high energy supply from the placenta. Failure to meet these demands may disrupt brain growth and maturation, especially in fetuses in whom CHD determines decreased cerebral blood flow and hypoxemia. In infants with HLHS, for example, more than half have microcephaly (<10th percentile) at birth. The head growth seems to be directly linked to the degree of ascending aortic flow. Brain maturation delay and cortical malformations are frequently seen on brain MRI and autopsy. Other prospective studies performed antenatally or postnatally in newborns with CHD demonstrate impairment of brain growth and metabolism in the third trimester, decreased pyramidal tract maturation, or widespread metabolic and microstructural brain abnormalities preoperatively, similar to preterm infants. Together, these studies support the concept that brain dysmaturation in CHD originates in utero.
Brain injury in neonates with CHD shares mechanisms common to preterm infants. WMI in neonates with CHD is linked to ischemia (e.g., hypotension) and inflammation. Based on experimental data, Buser et al. described how the primary mechanism of myelination failure in the human preterm neonate involves dysmaturation rather than cell loss, through a disrupted cellular response whereby pre-oligodendrocytes fail to differentiate into mature oligodendrocytes (i.e., oligodendrocyte progenitor maturation arrest). Similar findings were recently confirmed in a rodent model of CHD-related WMI. During the third trimester of gestation, early lineage oligodendroglia are vulnerable to insults that do not affect mature myelinating oligodendroglia. This results in a preponderance of WMI in neonates born before term, rather than the gray matter vulnerability seen in term neonates. Surprisingly, WMI is the characteristic brain injury pattern in term neonates with CHD and is indistinguishable from that seen in preterm neonates. Thus brain vulnerability in CHD appears to be a consequence of dysmaturation.
In human newborns, recent works have led to the recognition of abnormal brain maturation in utero and the importance of impaired brain maturation as a substrate for postnatal injury. In similar studies of neonates born preterm term, brain dysmaturation, rather than brain injury, is the major driver of adverse neurodevelopment. As the primary issue appears to be brain maturation arrest rather than cell loss, these data highlight the potential for brain recovery from injury.
Neuromonitoring
Intraoperative Monitoring
The modern surgical approach to the most complex cardiac lesions includes cardiopulmonary bypass (CPB) and deep hypothermic circulatory arrest (DHCA). Both procedures carry a risk of injury for the neonatal brain and are frequently associated with postoperative lesions. Infants 3 months of age or younger who undergo CHD surgery are at greater risk of transient cerebral ischemia from hypotension than older children, likely as a result of reduced cerebral autoregulation. As neuromonitor usage has increased in recent decades, improvements in neurodevelopmental outcomes of the infant with CHD have similarly been seen. Transcranial Doppler ultrasound is one method that has shown effectiveness in determining adequate cerebral perfusion and arterial saturation in real time during the low-flow CPB period. Similarly, somatosensory evoked potentials used intraoperatively are very specific and sensitive for neurological complications from hemodynamic disturbances. A study using multimodal intraoperative neuromonitoring combining electroencephalography (EEG), transcranial Doppler ultrasound, and near-infrared spectroscopy (NIRS) identified alterations in brain perfusion in 70% of patients, allowing corrective interventions in 74%. Such interventions were shown to improve neurologic outcomes (6% complication rate vs. 26% in the no-intervention group) and resulted in a decreased hospital length of stay. With the continued focus on improving neurodevelopmental outcomes in infants with CHD, intraoperative monitoring will continue to be an important tool in maximizing intraoperative cerebral protection.
Near-Infrared Spectroscopy
NIRS is a noninvasive bedside monitor that uses nonpulsatile oximetry to monitor hemoglobin oxygen saturation in a localized tissue bed. When used on the head, NIRS provides real-time information on the cerebral cortex oxygenation. NIRS has been shown to correlate well with superior vena cava oxygen saturation, highlighting its utility as a cerebral monitor. NIRS appears useful in both cyanotic and acyanotic heart disease, with optimal correlation at superior vena cava oxygenation concentrations of 40% to 60%, a point at which it is critical to intervene and improve oxygenation to protect brain function and homeostasis. As an intraoperative neuromonitoring system, NIRS has been shown to be a valuable tool in the assessment of hypocarbia and hypoxia and can alarm the surgical team to low cerebral perfusion. Whether or not intraoperative NIRS results in measurable improvements in neurodevelopmental outcomes remains uncertain; one study suggested that the only measurable negative outcome correlated with the NIRS intraoperative nadir was receptive language delay. Another report showed that neonates with values 20% to 40% below baseline during CHD surgery had increased neurologic dysfunction 12 months after surgery. NIRS as a marker of cerebral functioning is well supported by the literature, with its use in multimodal neuromonitoring providing significant benefit to both the infant with CHD as well as the surgical and clinical care teams.
Amplitude-Integrated Electroencephalography
As a marker of cerebral activity, amplitude-integrated EEG (aEEG) provides clinicians with valuable bedside information about the background electrical activity and can identify abnormal activity consistent with seizures. In a study of neonates with cyanotic and acyanotic CHD, aEEG patterns before and after the initiation of prostaglandin treatment showed abnormal voltage patterns in 60% of neonates at some point in the first 72 hours versus only 13% that continued to be abnormal at 72 hours after administration of prostaglandin. Severely abnormal patterns of burst suppression, continuous low voltage, or flat tracings were identified in 15% of neonates with no difference between the acyanotic and cyanotic groups. Abnormal aEEG patterns directly correlated with preoperative brain MRI injury scores; infants with severely abnormal aEEGs had the highest MRI injury scores. Severely abnormal aEEG background activity was associated with brain atrophy in 80% of cases with fewer numbers of focal infarcts and/or WMI. Sleep-wake cycling was present in up to 60% of neonates in the first 72 hours and was not associated with brain injury, although those with brain atrophy were less likely to have sleep-wake cycling present. Electrical seizures were identified in 19% of neonates; 11 of 12 were characterized as having acyanotic CHD. Clinical seizures strongly correlated with aEEG, with subclinical seizures identified in 2 of 12 neonates with seizures. Three neonates with suspected clinical seizures and aEEG patterns not consistent with a seizure were identified on conventional EEG to have focal electrographic seizure activity. This reflects a difficulty of aEEG as it has poor sensitivity for focal seizures not localized near the electrodes and showcases the importance of conventional EEG for further characterization of suspected seizures.
Continuous Electroencephalography
Neonates with CHD are at risk of seizures in both the preoperative and postoperative periods. The American Clinical Neurophysiology Society guideline on neonatal EEG monitoring in infants with CHD recommends consideration for continuous EEG (cEEG) monitoring during the postoperative period where seizure rates are 5% to 20%. HLHS has been shown to carry a greater risk of seizures in the postoperative period with 18% having seizures, compared with 4% in tetralogy of Fallot (TOF), 8% in TGA, and 12% with other biventricular defects. In a study of cEEG in the postoperative period at a single center, 8% of all infants with CHD had electrographic seizures with a median onset time at 20 hours (interquartile range 15–34 hours) after return to the cardiac intensive care unit. Clinical seizures with an electrographic correlate occurred in 15% of patients, with 85% of seizures occurring subclinically and identified purely on the basis of cEEG changes. Status epilepticus, classified as a single seizure lasting more than 30 minutes or recurrent seizures lasting for at least 30 minutes in a 60-minute period, occurred in 62% of those with seizures or 5% of all infants with CHD. Younger age at surgery, longer CPB and DHCA times, single-ventricle physiology and arch obstruction, delayed sternal closure, postoperative cardiac arrest, and extracorporeal membrane oxygenation were all factors that increased the likelihood of seizures. For every 10-minute increase in DHCA time, the odds ratio of developing seizures was 1.47, indicating an association with increased surgical time and subsequent brain dysfunction and injury. All infants with seizures were identified on neuroimaging as having either diffuse or multifocal lesions on either head ultrasound or brain MRI. Electrical seizure activity itself was associated with a 14-fold increase in the likelihood of an abnormal MRI scan. Given the strong association between seizures and CHD, cEEG should be considered in all neonates with CHD, particularly in the postoperative period, to better identify seizures, allowing for timely treatment, and assist in the identification of underlying brain injury.
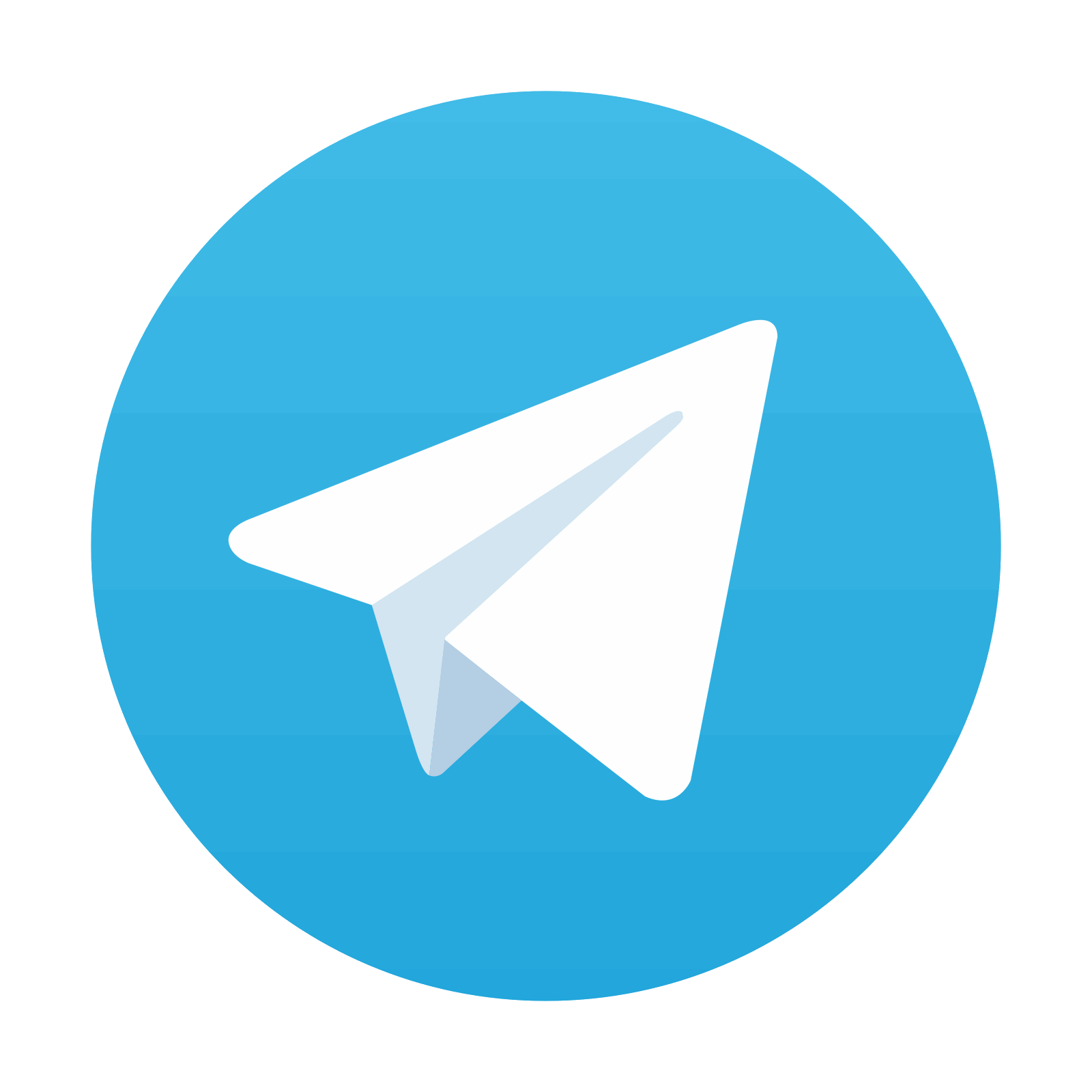
Stay updated, free articles. Join our Telegram channel

Full access? Get Clinical Tree
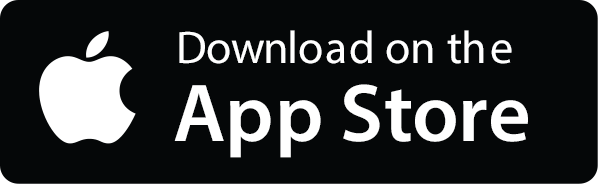
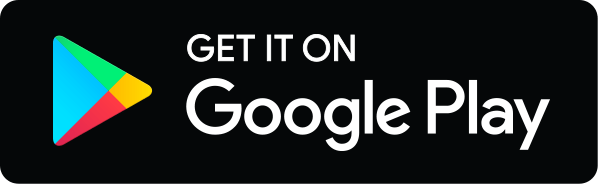