© Springer Science+Business Media LLC 2018
Frank W. Drislane and Peter W. Kaplan MBBS (eds.)Status EpilepticusCurrent Clinical Neurologyhttps://doi.org/10.1007/978-3-319-58200-9_2525. Consequences of Nonconvulsive Status Epilepticus: Experimental and Clinical Evidence
(1)
Departments of Neurology and Pediatrics, University of Virginia, 800394, Charlottesville, VA 22908, USA
(2)
Neurology & Neurotherapeutics, University of Texas Southwestern Medical Center, 8813, 5323 Harry Hines Blvd, Dallas, TX 75390, USA
Keywords
Nonconvulsive status epilepticusMechanisms of actionPreclinical studiesRetrospective studiesProspective studiesElectroencephalographySubarachnoid hemorrhageEpilepsyMorbidity“Data! Data! Data!” he cried impatiently. “I can’t make bricks without clay”.
Sir Arthur Conan Doyle, The Adventure of the Copper Beeches.
Introduction
Axis I of the recently updated International League Against Epilepsy (ILAE) classification of status epilepticus (SE) [1] divides SE into two forms: convulsive SE and nonconvulsive SE. Nonconvulsive SE (NCSE), i.e., prolonged self-sustaining seizures without prominent motor activity, is further subdivided into NCSE with coma and NCSE without coma. NCSE without coma includes both generalized (e.g., typical absence SE) and focal (e.g., NCSE with impaired consciousness, previously known as complex partial SE, CPSE) types.
The predominant feature of the majority of NCSE episodes is an ictal alteration of mental status characterized as lethargy, hallucinations, confusion, mood disturbances, bizarre behavior, or coma. A review of the semiologic description of 105 episodes of SE in the literature found that an alteration of mental status was present in 82% of those episodes [2]. Episodes in which the patient’s consciousness is preserved in the absence of prominent motor symptoms would be classified as focal NCSE without impaired awareness, previously known as simple partial SE. During a prolonged seizure of NCSE, motor manifestations may not be prominent, but subtle head and eye deviation, twitching movements, and myoclonia may be observed. Further, autonomic manifestations such as changes in heart rate and blood pressure may occur.
The ILAE definition of SE [1] includes two time points, t1 and t2. t1 represents when treatment should be initiated because after this time point the probability that the seizure will terminate without intervention is low. t2 is defined as the time point at which long-term consequences resulting from the episode of SE may occur. For generalized tonic-clonic SE, these time points are defined as t1 = 5 min and t2 = 30 min. Although t1 (10 min) and t2 (>60 min) values are provided for focal SE with impaired awareness, and an estimated t1 (10–15 min) value is provided for absence SE, the ILAE Task Force on Classification of Status Epilepticus noted that evidence in support of these values (or indeed, those for all forms of NCSE), are lacking and that additional studies are needed to begin to define these time points better.
The exact incidence of all forms of NCSE is not known and depends on the population studied, the setting, and the operational duration used to define an episode of NCSE [3, 4]. It is also clear that the era during which the study was performed is important, as the introduction of, and increasing use of, portable continuous electroencephalography (CEEG) technology in emergency departments and intensive care units has resulted in a greater recognition of NCSE [4, 5].
Recognition of NCSE often requires heightened clinical suspicion. NCSE can be preceded by a convulsive seizure that evolves to a stage of electromechanical dissociation, the so-called subtle status epilepticus [6], but frequently it occurs without an earlier convulsive seizure. In the emergency department and critical care settings, an alteration of mental status unresponsive to appropriate therapy for the underlying medical condition should lead to the use of EEG to evaluate for the possibility of NCSE [4].
Further discussion of the evaluation and treatment of NCSE are beyond the scope of this chapter. The primary question to be addressed here is one of prognosis: Does NCSE with coma, as observed in the critical care setting, lead directly to neuronal injury or change in neuronal function? The answer to this question may assist in optimizing management of this condition [7], especially given recent concerns that a higher risk of mortality is associated with the administration of anesthetic therapies [8–14]. The significant challenge of integrating evidence from heterogeneous preclinical and clinical studies has caused considerable debate on whether this form, or indeed any form, of NCSE has direct negative consequences for brain function, or whether the NCSE itself is a consequence of the primary insult and without its own independent influence on prognosis [15–17]. It has long been assumed that outcomes of NCSE are etiology-dependent rather than due to the NCSE per se. Hence, the current consensus is that the prognosis of NCSE with coma is typically worse in patients with acute neurologic illnesses compared to that in patients with prior epilepsy [18–20].
Consequences of Nonconvulsive Status Epilepticus: Preclinical Animal Models
Studies investigating the mechanisms and negative consequences of prolonged seizures and mechanisms of epileptogenesis have long depended on preclinical animal models in which seizures are induced in naïve animals using a chemoconvulsant such as the anticholinergic agent pilocarpine, electrical stimulation, or hyperthermia. Although this induction frequently induces a convulsive seizure, several of the induction techniques have been modified to induce prolonged seizures without a major motor component.
Several of these preclinical models replicate key features of absence SE [21–23] or CPSE [24–27]. A model for NCSE in the critically ill, however, is still lacking. Despite this absence, a review of the preclinical literature is instructive when considering the question of the consequences of NCSE in this population as there are several examples in which it has been demonstrated that prolonged seizures, not accompanied by convulsive movements or secondary systemic changes (hyperpyrexia, lactic acidosis, hypotension, hypoxia, and hypoglycemia), are capable of producing neuronal injury.
The classic example of this finding was a study performed by Meldrum and colleagues [28] in which seizures were induced using the GABA antagonist bicuculline in paralyzed, artificially ventilated baboons. In these animals, with minimal secondary physiologic changes (e.g., a mild increase in temperature and slight decrease in arterial oxygen tension in several of the animals), ischemic neuronal injury was observed in the neocortex, thalamus, and hippocampus, in several animals in which the seizure lasted longer than approx. 3.5 h [28, 29]. An important observation was that while injury was present, it was to a lesser degree than that observed following convulsions in the same model [29], suggesting that the neuronal injury was the result of both the prolonged, recurrent neuronal activation that occurs during the seizure and the secondary systemic changes that occur in response to the seizure.
This finding in the paralyzed, ventilated baboon was subsequently confirmed by Nevander and colleagues using a flurothyl-induced model of SE in ventilated and well-oxygenated rats [30]. Histologic studies completed 1 week after prolonged seizures of 15–120 min or longer showed seizure duration-dependent neuropathologic changes—including infarction of the substantia nigra and pars reticulata following SE for 30 min. After 45–120 min of seizures, damage was observed in layers 3 and 4 of the neocortex. Seizure duration of 60–120 min resulted in neuronal damage that extended to the amygdala, thalamus, and hippocampal CA4 and CA1 regions. Interestingly, CA3 and dentate granule cells remained unaffected. For seizure duration beyond 120 min, there was damage in both the neocortex and ventral posterior thalamic nuclei, together with evidence of neuronal necrosis at sites close to the ventricles.
A limitation of studies in which prolonged seizures are induced using a systemic chemoconvulsant is the potential that the injury is a direct result of the chemoconvulsant and not the seizure. Electrical stimulation to induce the seizure avoids this limitation of chemoconvulsants, and there are several examples in which prolonged electrical stimulation in the absence of convulsive seizures was correlated with neuronal injury. For example, Sloviter and colleagues performed electrical stimulation of the angular bundle of the perforant pathway in adult rats for a period of 24 h [25]. During the stimulation, the animals were anesthetized, and although occasional wet dog shakes (automatisms resulting from activation of the dentate gyrus) were observed, convulsions did not occur. Histologic examination showed extensive dorsal hippocampal damage on the stimulated side, including in the hilar interneurons in the dentate gyrus and CA3 and CA1 pyramidal neurons.
Similarly, Thompson, Wasterlain and colleagues [26] demonstrated that prolonged focal electrical stimulation in young animals resulted in neuronal death. Focal NCSE was induced in developing animals (postnatal day 14–15—which approximates the brain development of a human toddler) by stimulating the perforant pathway for 16 h. During the stimulation, the animals exhibited wet dog shakes and hind limb scratching. Forelimb clonic movements were observed only rarely. The day after stimulation, there was significant necrotic death of interneurons in the hilar region and of pyramidal cells in the CA1 and CA3 regions of the hippocampus ipsilateral to stimulation. This necrotic cell death was also present in the contralateral CA1 and CA3 regions and extended to the ipsilateral and contralateral subiculum and prepiriform cortex.
Brandt, Löscher and colleagues [27] electrically stimulated the basolateral amygdala to induce prolonged focal nonconvulsive seizures, focal nonconvulsive seizures intermittently interrupted by generalized convulsive seizures, and prolonged generalized convulsive seizures. Histologic studies performed 48 h after NCSE showed that in animals with NCSE lasting longer than 1 h, ipsilateral neurodegeneration was apparent in many regions, including in the amygdala, piriform cortex, entorhinal cortex, endopiriform nucleus, and mediodorsal thalamus, whereas no damage was found in the hippocampus or substantia nigra. As in the studies of Meldrum and colleagues reviewed above, the injury in NCSE was more restricted and less severe than that found when convulsive seizures were observed. Further, although there was a high probability (90%) of developing chronic epilepsy in those animals with convulsive seizures, about 30% of the animals in the focal NCSE group developed chronic epilepsy. Although the electrically induced focal NCSE that occurred in this study and in that of Thompson and colleagues [26] are more directly comparable to CPSE than to focal NCSE in critically ill patients (in whom the episode of NCSE occurs in the setting of traumatic brain injury, subdural hemorrhage, or stroke), these findings raise concern that prolonged excessive neuronal activity in a focal region of the brain has the capacity to directly induce cell death.
A focal preclinical model that may be considered a better reflection of central nervous system consequences of nonconvulsive seizures in the critically ill is one in which nonconvulsive seizures were induced by an ischemic injury resulting from either permanent or transient (2 h) occlusion of the right middle cerebral artery (MCA) in adult rats [31]. In this model, the nonconvulsive seizure begins over the ischemic right hemisphere and may then spread to involve the nonischemic left hemisphere. The seizures lasted on average 60 s, and recurred on average 10 times, in the first two hours of occlusion. The seizures were accompanied by periodic discharges and intermittent EEG rhythmic activity that commenced at approximately 1 h after the occlusion and continued for up to the next 72 h. Studies using this model provided confirmatory evidence that nonconvulsive seizures per se following MCA occlusion contribute to neuronal injury and functional morbidity. The authors found that treatment with antiseizure drugs, either immediately before or within 30 min of MCA occlusion, reduced the incidence of seizures after stroke. Importantly, the reduction in seizures was correlated with reduced brain infarct volume and mortality and improved neurologic recovery [32, 33].
Consequences of Nonconvulsive Status Epilepticus: Clinical Studies
Norbert Weiner, often credited as the father of Cybernetics (i.e., systems theory), is frequently quoted as having said, “the best material model of a cat is another, or preferably the same, cat.” The preclinical models reviewed to this point demonstrate that persistent abnormal neuronal activity may result in neuronal death in the absence of convulsive movements. It is fully appreciated, however, that focal limbic SE, with or without secondarily generalized convulsive seizures, in the freely moving or paralyzed, ventilated animal as induced by either a chemoconvulsant or electrical stimulation, may not be the best model for the cat of interest, which is NCSE in the critically ill patient. Therefore, it is important to review those studies performed in the ‘same cat,’ i.e., human patients.
The literature is replete with examples demonstrating that critically ill children and adults with nonconvulsive seizures and NCSE are at higher risk for morbidity and mortality [34–48]. Many of these studies suggest that outcome is strongly influenced by the underlying etiology rather than by the NCSE per se [18, 49–58].
Despite this association of underlying etiology with outcome, several recent studies suggest that the NCSE itself may also directly negatively affect outcome. For example, Payne and colleagues [59] rigorously addressed the relationship of electrographic seizures to declining neurologic function. They prospectively studied continuous video-EEG records (21–56 h) of 259 critically ill children (ages 0.3–9.7 years) and found that electrographic seizures occurred in 93 of the children (36%), of whom a third had a prior diagnosis of epilepsy: 14% of the subjects with seizures had exclusively convulsive seizures, 47% a combination of convulsive and nonconvulsive seizures, and 40% exclusively nonconvulsive seizures. Among these children, electrographic SE was found in 23 (9% of the entire study population). Among all subjects, 67% had a neurologic decline (from the time of admission) by at least 1 point on the pediatric cerebral performance category (PCPC) scale. Most importantly, for the question of whether NCSE worsens prognosis, the study showed that an increased electrographic ‘seizure burden,’ greater than 20% per hour (i.e., >12 min of seizure activity per hour), significantly accelerated neurologic decline and worsened PCPC scores (although without an impact on mortality). Children with PCPC worsening had a mean seizure burden of 15.7% versus 1.8% for those without PCPC worsening. Seizure duration was independently associated with neurologic decline regardless of the diagnostic category, although subjects with systemic diseases and acute seizures appeared to fare worse. This study provided evidence that electrographic seizure burden per se is strongly associated with increased morbidity in critically ill children, implying the need for aggressive antiseizure drug therapy. Still, as 80% of the children in this study received antiseizure drugs before and during EEG monitoring without a change in the association between seizure burden and neurologic outcomes as determined by the PCPC scale, it remains an open question whether earlier and more aggressive treatment, including the use of more sedating agents, could improve outcomes [43, 60].
While the study by Payne and colleagues looked at short-term outcome, Abend and colleagues [42] reported the long-term outcomes in children admitted to the Pediatric ICU and diagnosed with NCSE. At after-discharge follow-up (median duration 2.6 years) for 60 children, they found that NCSE (but not isolated electrographic seizures) in children with previously normal developmental baselines was associated with an unfavorable developmental outcome, including decreased quality of life. Only 13% of children with NCSE had a favorable outcome whereas 64% of the subjects who did not have seizures (or had isolated electrographic seizures, but not SE) had a relatively favorable developmental outcome and PCPC scores. Further, among children without a prior history of epilepsy, having electrographic SE was associated with an increased risk for the development of epilepsy (69%) compared to the risk for those who had isolated electrographic seizures (38%) or no seizures (21%), consistent with earlier data [61]. The study was expanded to assess neurobehavioral outcomes in a smaller cohort measuring adaptive and daily skills, emotional problems, competencies, and real-world behavioral manifestation of executive skills. Patients with NCSE (32 patients; age range: 2.0–9.8 years) had worse adaptive behavior compared to those with no seizures. There was also a trend toward worse behavioral-emotional and executive skills, but the smaller sample size probably precluded statistical significance. Interestingly, in this expanded study, the authors did not find significant differences in worsened outcomes between patients with ‘electrical seizures’ and ‘electrical SE’ (the terminology adopted in the studies). This finding may be the result of inadequate seizure burden stratification in the study, as NCSE can involve multiple brief seizures rather than just a single prolonged seizure. While this caveat means that we still do not know whether the negative outcomes are due to seizures per se or their underlying triggers, the data suggest the need for expeditious treatment of electrographic seizures and the potential for NCSE per se to at least contribute to or exacerbate poor outcomes.
In adults, Punia and colleagues [62] retrospectively studied 1163 consecutive patients admitted to the ICU who had CEEG monitoring. Of the 200 patients they detected having periodic lateralized epileptiform discharges (PLEDs) or nonconvulsive seizures or both on the EEG, they obtained follow-up on 118 patients. The predominant etiologies in these patients were stroke (28%), hemorrhage (26%), and tumor (14%). They found that 24% of patients in the PLEDs-only group had seizure recurrence after discharge and, even after excluding those with a prior history of epilepsy, patients who had nonconvulsive seizures in the ICU (with or without PLEDs) had a 5 times greater likelihood of seizure recurrence than those with PLEDs alone [63].
Recently, Claassen and colleagues [64] reported the relationship between seizure burden, as defined by duration of seizures on CEEG, and functional and cognitive outcomes 3 months after subarachnoid hemorrhage (SAH). They studied the records of 420 SAH patients admitted from 1996 to 2013 who underwent CEEG for an average duration of 96 h. They found that 50 patients (12%) had seizures on EEG, including NCSE in 46 patients (92%) and nonconvulsive seizures in 4 (8%). NCSE had an average duration of 6 h. At 3 months, 178 patients (58%) were either dead or disabled and, after adjusting for other predictors of outcome, seizures were associated with a threefold greater probability of worsened functional outcome (modified Rankin Scale) compared to that for patients without seizures. Among patients with seizures, the magnitude of association of NCSE to poorer outcomes was stronger. Importantly, the authors found a direct association of seizure burden to negative functional outcome. Every additional hour of NCSE was associated with a 10% greater likelihood of functional disability or death at 3 months. Cognitive outcomes were measured by telephone interview (TICS, a scale that ranges from 0 to 51) 3 months after SAH in a third of the patients (121), of whom 11 (9.1%) had had at least one seizure on the CEEG. Although nonconvulsive seizures were not independently associated with worsened cognitive outcome, seizure burden was, as there was a small but measurable decrease of 0.19 points (95% CI −0.33 to −0.05) in the TIC score for every 1 h of seizure activity. Overall, the study demonstrated that both nonconvulsive seizures and NCSE result in poorer functional outcomes with increasing seizure burden, independently worsening cognitive outcome.
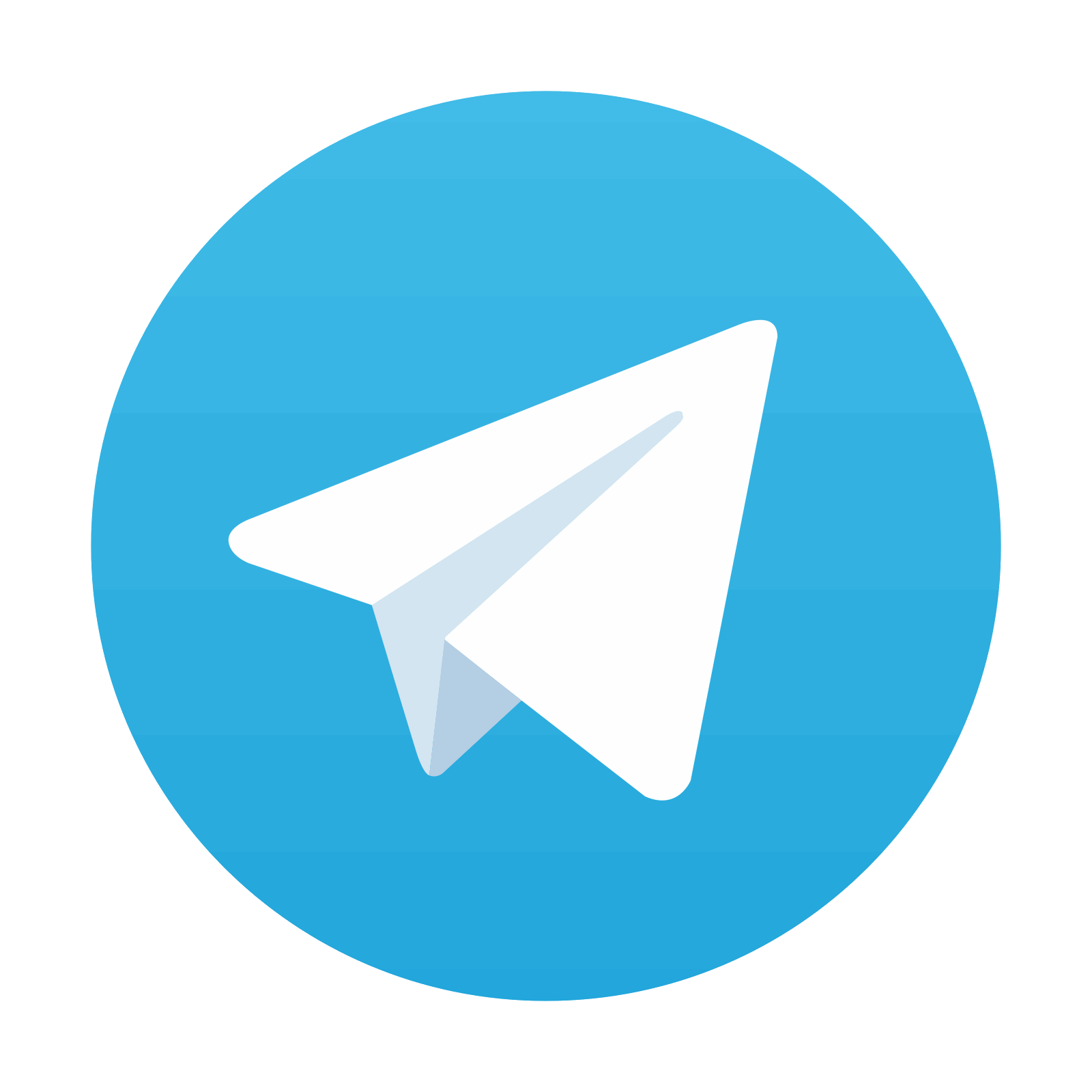
Stay updated, free articles. Join our Telegram channel

Full access? Get Clinical Tree
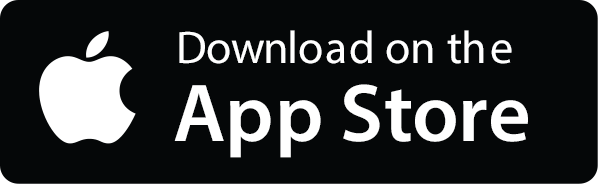
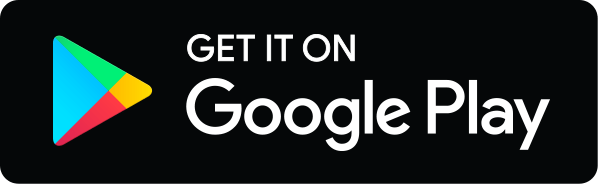