Fig. 16.1
Crosstalk in bimanual visuomotor learning (modified from [7]). (a) Participants performed bimanual reaching movements and the cursor’s position reflected the movement of the right hand (this was explicitly mentioned to them). (b) The clockwise visual rotation of the cursor was gradually increased, leading to the movement direction of the right hand rotating to the opposite direction. However, at the same time, the movement direction of the left hand was also rotated, indicating that the movement error of the cursor was implicitly assigned to the left hand’s movement controller
16.1.3 Limitation of Motor Learning System
The error-based correction of motor commands is a powerful scheme, but the crosstalk described above is an example showing that the correction often leads to an erroneous situation. Another famous limitation of the motor learning system is its inability to assign distinct motor memories for identical movements. For example, when participants were asked to perform reaching movements under the presence of the clockwise or counterclockwise force field, it was difficult to adapt to these opposing force fields simultaneously even if the directions of the force fields were cued by the color of the target [8, 9] (however, Osu et al. [9] reported that simultaneous adaptation was possible when the opposing force fields were provided in a random order).
This difficulty seems strange considering the flexibility of our motor learning system. Thus, how the motor system overcomes this limitation is one of the unsolved problems in the field of research on motor control and learning [3]. In the following sections, I will demonstrate that the motor learning system actually has the flexibility to adapt to distinct conditions according to distinct behavioral contexts.
16.2 Distinct Motor Memories for Unimanual and Bimanual Movement
In 2006, we demonstrated an example of flexible switching among motor memories [10]. In the experiment, the participants adapted the reaching movements of one arm to a force field and we examined the aftereffects when they performed the same unimanual or bimanual reaching movement (Fig. 16.2a, b). Notably, the kinematics of the learned arm were identical regardless of whether the movements were unimanual or bimanual. If there is only one memory for the learned arm, then we should observe the same aftereffects, reflecting the amount of motor learning. However, the aftereffect was significantly smaller (by approximately 30–40 %) for bimanual movements (Fig. 16.2c). We performed a similar experiment in which the force field applied to one arm was learned bimanually and again found a reduction of the after effect for unimanual movement, indicating that the motor memories for identical movements could be partially switched according to whether the opposite arm is stationary (i.e., unimanual movement) or moving (i.e., bimanual movement) (Fig. 16.2d).
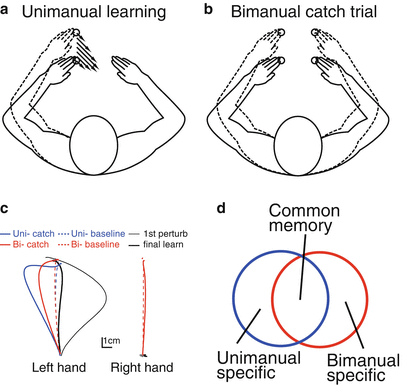
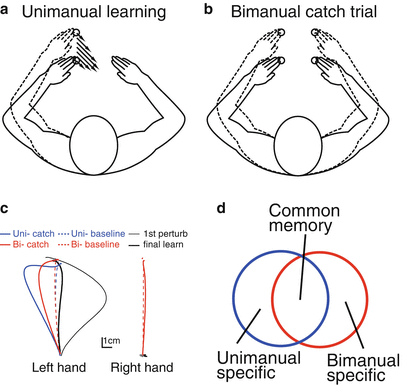
Fig. 16.2
Partial learning transfer between unimanual and bimanual movements (modified from [10]). A velocity-dependent force field to the left arm was learned unimanually (a) and then we interleaved catch trials in which the force field was suddenly turned off to compare the aftereffects between unimanual and bimanual catch trials (b). The aftereffect of the unimanual movement was significantly greater than that of the bimanual movement (c), indicating the motor memories for identical left reaching movements were partially segregated between unimanual and bimanual movements (d)
We predicted that such a partially segregated structure of motor memories enabled participants to adapt the identical movements to conflicting force fields if each of the force fields was associated to a unimanual or bimanual movement. This is because memories specific for unimanual or bimanual movements can be formed and retained for each force field. To examine this hypothesis, participants performed unimanual and bimanual reaching movements in an alternate fashion, and one arm was exposed to either a clockwise or a counterclockwise force field depending on whether the participants were performing a unimanual or bimanual movement (Fig. 16.3a, b). The movement error gradually decreased with training, and a significant aftereffect was observed (Fig. 16.3c), indicating that distinct motor memories enable adaptation of the same left arm movement to conflicting force fields if each force field is associated only with a unimanual or bimanual movement.
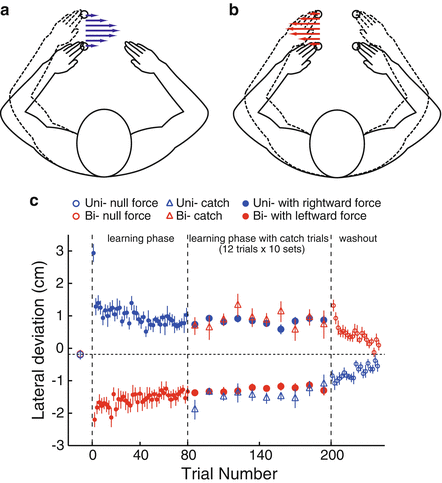
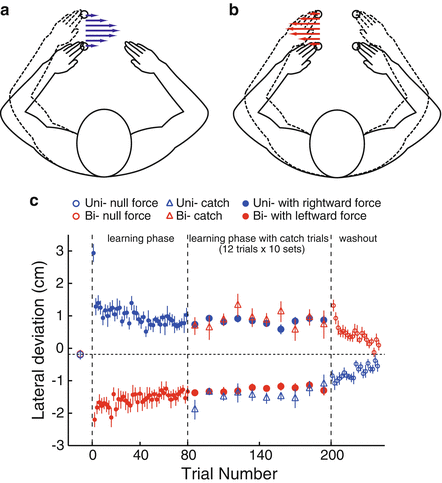
Fig. 16.3
Partially segregated motor memories enable adaptation to two conflicting force fields (modified from [10]). Rightward (a) and leftward (b) force fields were applied to the left arm moving alone (unimanual movement) and together with the right arm (bimanual movement), respectively. (c) The movement error gradually decreased with training, and significant aftereffects were observed, indicating the simultaneous learning of two opposing force fields
16.3 Other Examples of Context-Dependent Memory Formation
After our findings on motor memory switching between unimanual and bimanual movements, a number of examples of motor memory switching have been reported. For example, the movement of a limb can be performed in a discrete way (i.e., discrete movement) or in a continuous rhythmic way (i.e., rhythmic movement). However, even if the kinematics of movements are almost identical between discrete reaching movements and one cycle of rhythmic movement, the motor memory is not transferrable between them. We had participants adapt their out-and-back reaching movements to a visual rotation (i.e., the movement of the cursor was rotated by 30° around the starting position) while performing the movements in a discrete way [11]. After they completed the adaptation, we asked them to perform identical movements in a rhythmic way. Not surprisingly, they could transfer the adaptation to the rhythmic movements; no substantial movement errors were observed (Fig. 16.4a). However, after the participants adapted to the same visual rotation through rhythmic movements, significant movement errors appeared (Fig. 16.4b). A similar observation was reported by Howard et al. for adaptation to a novel force field [12], indicating that the motor memory was partially distinct for identical movements performed either discretely or rhythmically.
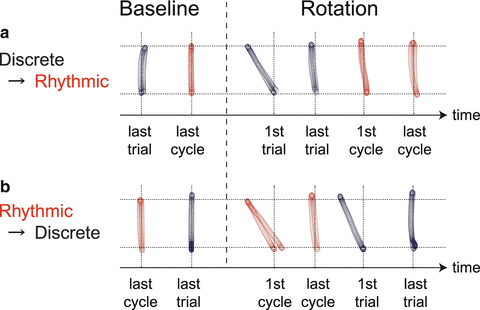
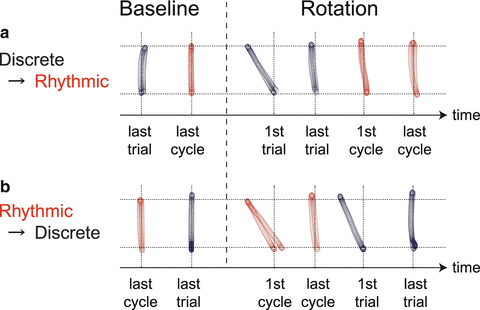
Fig. 16.4
Asymmetric transfer of visuomotor learning (modified from [11]). (a) When the visual rotation of 30° was learned through discrete movements, the motor learning effect was fully transferred to the rhythmic movement. (b) However, after adaptation to the same condition through rhythmic movements, significantly large errors appeared once again
Another important factor for distinct motor memory formation might be movement plans. We have developed an experimental paradigm to produce a strange situation wherein participants performed physically identical reaching movements according to distinct movement plans [13]. In this experiment, participants reached toward one of two targets alternately (Fig. 16.5a). A key feature of this experiment was, when reaching toward the right target, a clockwise visual rotation was applied to the cursor representing the handle position, and when reaching toward the left target, a counterclockwise visual rotation was applied to the cursor. A gradual increase in rotation implicitly brought the directions of reaching movements closer and closer until they were finally not discernible (Fig. 16.5b). The participants strongly believed that they reached toward distinct targets, although, in fact, the movements were physically almost identical. After this strange situation was created, we examined whether the participants could adapt these identical movements to conflicting force fields if the movement plans were different. Indeed, the conflicting force fields were learnable, indicating that distinct motor memories were formed and retrieved for physically identical movements according to distinct movement plans.
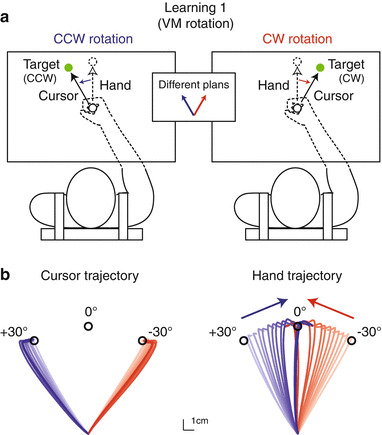
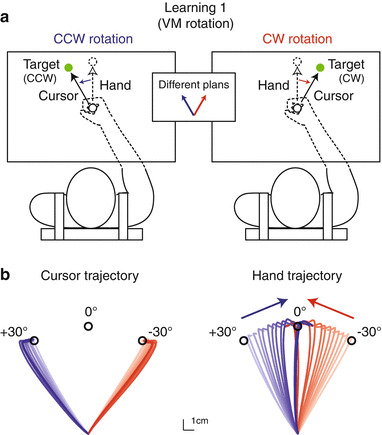
Fig. 16.5
Physically identical movements performed by distinct movement plans (modified from [11]). (a) Clockwise (CW) and counterclockwise (CCW) visual rotations were applied to the cursor when participants reached toward CW and CCW targets, respectively. (b) Gradual increase in visual rotations led the directions of physical movement of the hand to become closer and finally almost identical (right panel). Note that participants strongly believed that they kept reaching toward distinct targets (left panel indicates the cursor’s movements)
The importance of movement plans for distinct motor memories formation and retrieval has been recently reported [14, 15]. Ingram et al. (2010) have developed a new experimental task wherein participants grasped and rotated the handle of a virtual object, and the translational force field was imposed when trying to rotate the object [14]. Thus, participants needed to compensate for the force to the handle accompanied with the rotation of the object. They provided clear experimental evidence that distinct motor memories can be developed for physically identical rotation movements according to the configuration of the virtual object, i.e., how participants plan to move the object from a particular configuration to another.
16.4 Possible Neuronal Mechanisms of Motor Memory Switching
Coming back to the topic of motor memory switching between unimanual and bimanual movements, there are two issues we need to address: first, how these switchings are implemented in the brain and, second, why the brain uses the identical motor memories inconsistently. In this chapter, I will discuss the first topic.
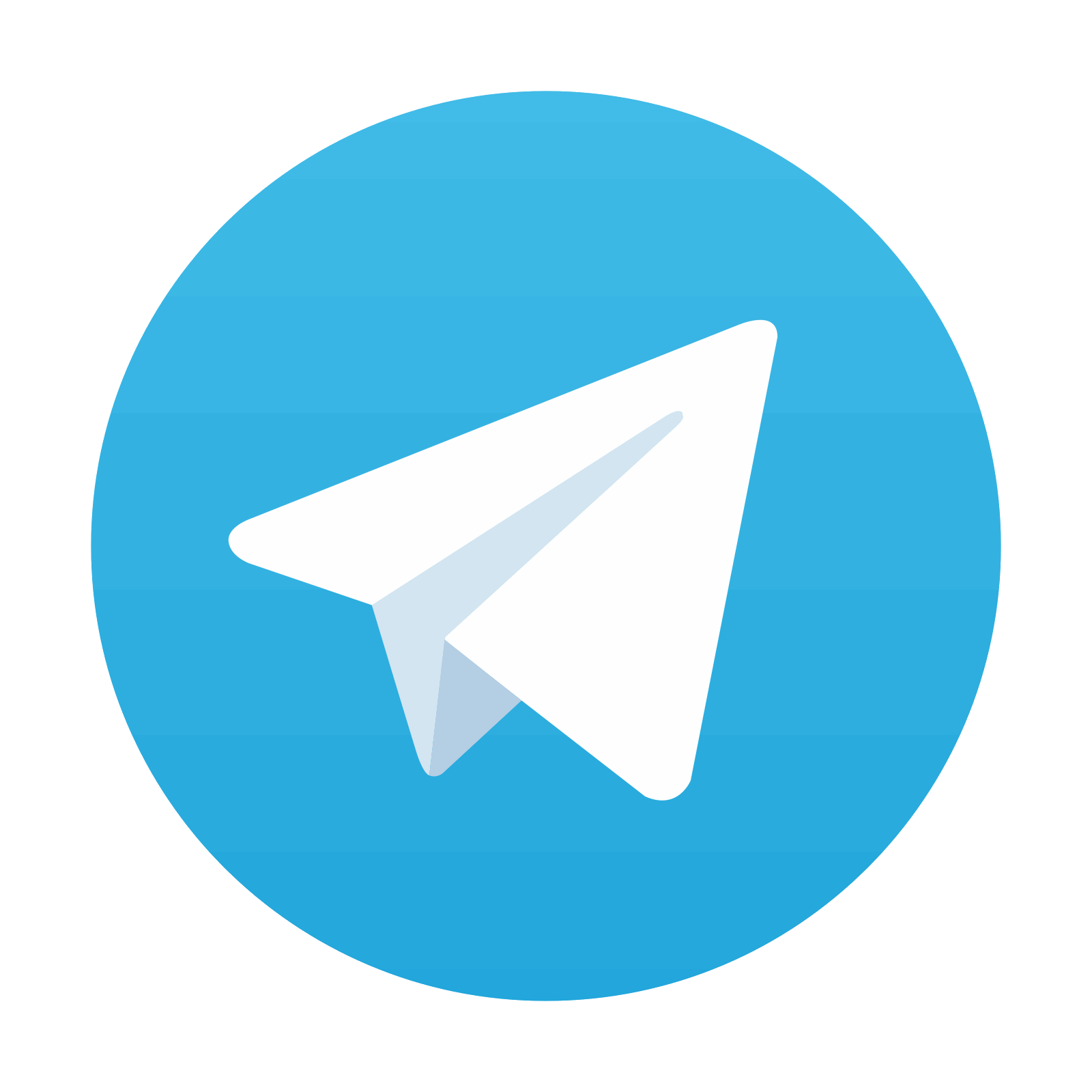
Stay updated, free articles. Join our Telegram channel

Full access? Get Clinical Tree
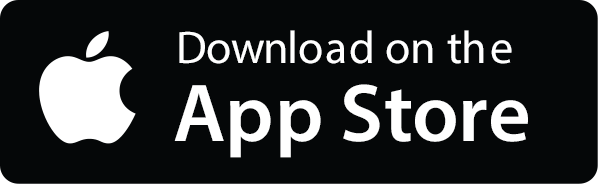
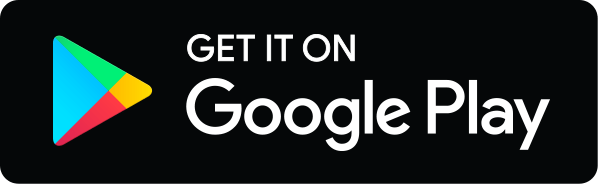