A 60-year-old man presents to the emergency department with progressive dysarthria and confusion over the prior 24 hours. His wife states that in the past month her husband complained of episodic headaches that occasionally were associated with nausea. On examination, he is obtunded and moaning to painful stimulation. He has a left gaze preference, but localizes equally with his arms and legs. The tone in his legs is increased with bilateral upgoing toes. His noncontrast head computed tomography (CT) reveals a left frontal lesion with mass effect and midline shift (Figure 15-1). He is admitted to the neurologic intensive care unit (NeuroICU) for further evaluation and management.
There is a mismatch between the neurologic examination and imaging findings; therefore, alternate causes for altered mental status must be explored. The frontal left hypodensity with a surrounding hyperdensity should not account for such a degree of obtundation. Depending on the clinical scenario, this may take the form of obtaining further history or diagnostic tests.
Upon further questioning, his wife states that over the last 2 weeks he has been more forgetful, with fluctuating irritability that lasts anywhere from minutes to hours. She denies any rhythmic jerking of his arms or legs or loss of consciousness, incontinence, or tongue biting. Upon arrival to the NeuroICU, magnetic resonance imaging (MRI) with and without gadolinium is performed, and he is subsequently connected to continuous electroencephalographic (cEEG) monitoring (Figure 15-2).
The goal of neuromonitoring is to identify secondary brain injury as early as possible and prevent permanent injury by triggering timely interventions. Ideally, such monitoring should be highly sensitive and specific, noninvasive, widely available, and relatively inexpensive; pose no risks to patients; have high inter- and intrarater reliability; and have good temporal and spatial resolution. Limitations of cEEG monitoring include high cost, vulnerability to artifact and medications, poor spatial resolution, and poor inter- and intra-rater reliability. On the other hand, it is noninvasive (as long as limited to surface EEG monitoring), has great temporal resolution, and allows assessment of neuronal activity.
To rule out subclinical or nonconvulsive seizures in patients with
persistently impaired mental status after a convulsive seizure or convulsive status epilepticus (SE)
ongoing or frequently recurring movements, including isolated eye movements that may or may not represent seizures
impaired mental status and a history of epilepsy
unexplained impaired brain function after basic evaluation (laboratory and imaging)
acute brain injury with stupor or coma, including postanoxic coma, sepsis
unexplained fluctuations in mental status including those secondary to hypoxia and increased intracranial pressure (ICP; ie, hydrocephalus, intracranial hemorrhage, and intraventricular hemorrhage)
To characterize paroxysmal clinical events including posturing, rigidity, tremors, chewing, or even autonomic spells such as sudden hypertension, tachycardia, bradycardia, or apnea
Epileptiform activity or periodic discharges on initial EEG (often performed for ≤ 30 minutes)
To detect cerebral ischemia (ie, patients with subarachnoid hemorrhage (SAH) at risk for delayed cerebral ischemia)
To guide medication titration and quantify seizure frequency in patients with refractory status epilepticus (RSE).
Nonconvulsive seizures (NCSs) and nonconvulsive status epilepticus (NCSE) are frequently seen in the ICU setting. the presentation can be similar to that of the patient described in this vignette. Even in the medical ICU population after excluding all patients with any clinical suspicion of seizures, approximately 8% of comatose patients have electrographic seizures.1,2 Electrographic seizures can be detected by cEEG in 20% to 48% and NCSE in 14% pf patients in the aftermath of clinically successfully treated convulsive SE.3,4 In the acute brain injury setting electrographic seizures are even more frequent, but the incidence clearly depends on the underlying etiology. However, no population-based studies are available to date, and thus the real incidence is unknown. In the NeuroICU setting, subclinical seizures were seen in 18% of a cohort of 570 consecutive patients who underwent cEEG for the detection of subclinical seizures or to evaluate an unexplained decrease in consciousness.5 In this study, seizures were more frequently recorded in younger patients with a history of epilepsy, patients who had convulsive seizures prior to start of cEEG monitoring, and those who were comatose at the time cEEG was started. When stratified by etiology, either pediatric seizures, nonconvulsive seizures, or NCSE can be seen in 25% to 50% of those undergoing monitoring (Table 15-1). Although the majority of seizures are recorded in the first 24 hours, frequently 48 hours or more of cEEG are needed to record the first seizure in comatose patients (Figure 15-3).5
Diagnosis | PEDs (N = 1096) | Surface EEG NCS (N = 581) | NCSE (N = 581) | TCME NCS (N = 40) |
---|---|---|---|---|
CNS infection | 23% | 9% | 17% | na |
Toxic-metabolic encephalopathy | 26% | 13% | 8% | na |
Epilepsy-related seizures | 11% | 11% | 20% | 75% (3/4) |
Brain tumor | 17% | 11% | 12% | na |
Postneurosurgery | 13% | 15% | 8% | na |
Subarachnoid hemorrhage | 16% | 5% | 13% | 55% (11/20) |
Traumatic brain injury | 13% | 10% | 8% | 50% (3/6) |
Intracranial hemorrhage | 17% | 4% | 9% | 29% (2/7) |
Unexplained decrease in loss of consciousness | 10% | 10% | 5% | na |
AIS | 16% | 2% | 7% | 50% (1/2) |
Sepsis | 25% | 11% | 11% | na |
Figure 15-3.
Time to detect the first seizure in 110 of 570 critically ill patients who had seizures recorded on cEEG. (Reproduced with permission from Claassen J, Mayer SA, Kowalski RG, et al. Detection of electrographic seizures with continuous EEG monitoring in critically ill patients. Neurology. 2004;62(10):1743-1748.)

Concerns for increased ICP leads to administration of a mannitol bolus that did not result in a change in the patient’s clinical findings. The EEG has now changed to an evolving ictal pattern in the left hemisphere consistent with NCSE (Figure 15-4). The patient is given a loading of fosphenytoin, 20 mg/kg, but seizures persisted despite repeated boluses of lorazepam. He is intubated and started on a continuous infusion of midazolam (for details, refer to the SE protocol in Chapter 3, Status Epilepticus).
In addition to looking at the raw EEG data, the EEG recordings can be subjected to quantitative analysis. A number of different quantification methods are available with most commercially available EEG software. Quantification techniques include the calculation of power spectra by means of Fast Fourier Transform (FFT), which then can be used to calculate ratios of fast to slow activity or other parameters. These can be displayed as numbers or graphically as compressed spectral arrays (CSAs), histograms, or staggered arrays, which have the potential to unmask subtle background changes when trended over time. The quantitative electroencephalogram (qEEG) parameters may include total power, frequency activity totals (eg, total or percentage alpha power), spectral edge frequencies (eg, frequency below which 50% of the EEG resides), amplitude-integrated interval, frequency ratios (eg, alpha over delta ratio), and brain symmetry index.6 Other more automated EEG data reduction display formats include the cerebral function monitor (CFAM), EEG density modulation, automated analysis of segmented EEG (AAS-EEG) and bispectral index (BIS) monitor.7-11 However, qEEG should always be interpreted in the context of the raw EEG, since artifacts, medications, and other clinical events may cause findings that are very similar to epileptiform findings (Figure 15-5). This is particularly true for software packages that display “user friendly” composite scores, and any outputs that are based on unpublished algorithms (“proprietary information”) should be viewed with great caution. The qEEG parameters are particularly useful for seizure quantification and to estimate effects of seizure treatment once the qEEG “footprint” of a seizure has been identified.
For continued NCSE a midazolam drip is rapidly titrated up until seizures are controlled. The next morning, while receiving midazolam the patient undergoes tumor resection with no complications. Postoperatively midazolam is weaned and on postoperative day 2, he is transferred to the medical unit with signs of a mild residual neglect. Biopsy reveals glioblastoma multiforme.
Figure 15-5.
A quantitative electroencephalogram (qEEG) taken from a different patient than the case presented in the text. A 14-minute qEEG recording with multiple nonconvulsive seizures and brief potentially ictal rhythmic discharges (B(i)RDs) are maximal on the right as evident on amplitude integrated EEG (aEEG; higher amplitude on the right) with each seizure. The standard spectrogram and the asymmetry spectrogram both demonstrate involvement of all frequencies. Note the 5 episodes labeled as “artifact” in which the aEEG tracing jumps up in a manner almost identical, though more prominent over the left hemisphere, to the prior and subsequent seizures and B(i)RDS. However, review of the raw EEG revealed muscle artifact. (Reproduced with permission from Hirsch LJ, Brenner RP. Atlas of EEG in Critical Care. Hoboken, NJ: Wiley-Blackwell; 2010. Figure 7.10, page 238.)

In addition to seizure quantification, qEEG is useful for trending patterns over time, as the transitions may not be as evident on review of the raw data. The qEEG and raw EEG in Figure15-6 illustrate resolution of NCSE over an extended period of time.
Figure 15-6.
Resolution of partial nonconvulsive status epilepticus shown on compressed spectral array (CSA). CSA showing gradual resolution of nonconvulsive status epilepticus over a few hours. CSA is particularly useful for recognition of such long-term trends. Arrows indicate approximate time periods in the CSA from which the EEG samples are taken. Y-axis: frequency, 0 Hz at bottom, 60 Hz at top. X-axis: time (approximately 4 hours). Color scale (z-axis) power of given frequency (scale in upper right; μV/Hz). Z-axis: voltage, measured in μV/Hz.

In addition to the quantitative measures described above, seizure detection programs using specialized EEG signal processing software can be used for screening large cEEG datasets, allowing the reviewer to hone in on marked segments (Figure 15-7, row 1: Detected Seizure Probability). These programs may be based on a simple FFT-based frequency analysis, recognize specific wave form patterns, or incorporate a complex learning algorithm that recognizes more combinations and sequential developments of typical waveform. Based on the FFT analysis of EEG, CSA graphs can be generated to determine the occurrence of subclinical seizures once the “CSA signature” or footprint of a seizure in an individual patient has been determined.12 This can be used to quickly screen a 24-hour recording and quantify the frequency of seizures.
Others have investigated the utility of the cerebral function analyzing monitor (CFAM) to detect seizures, but sensitivity was particularly low for partial seizures.12 Other algorithms were limited by a high false-positive rate with good sensitivity.12 While promising, commercially available automated seizure detection software has been developed for use in epilepsy monitoring units and programmed to identify seizures from healthy patients. However, seizures in brain-injured and often medically sick comatose patients have rather different patterns, which are typically less organized and have a slower maximum frequency and longer duration, with unclear onset and offset. Thus, in order to utilize seizure detection programs in the NeuroICU, new software will need to be developed and programmed to more accurately identify complex ictal patterns. Automated seizure detection software based on multichannel, digitized real-time FFT (2 seconds per epoch, 2-minute averaging), and trends of total EEG power has been used by some with reasonable success to screen for seizures11,12 and save review time without compromising sensitivity.13
A 57-year-old woman is admitted to the NeuroICU with aneurysmal subarachnoid hemorrhage (SAH) from a right posterior communicating artery aneurysm (Hunt Hess grade 4, Fisher 3, modified Fisher 3) that is clipped. Her immediate postoperative noncontrast head CT does not reveal any infarcts. Her Glasgow Coma Score (GCS) is 14, and she is subsequently extubated. Transcranial Doppler flow velocities are mildly elevated on post-bleed day 6 without any new neurological findings. On post-bleed day 7, her GCS dropped to 12 and her CT scan reveals an acute stroke in the left internal capsule (Figure 15-8). She receives a digital subtraction angiogram, which demonstrates severe vasospasm in the distal right middle cerebral and left vertebral arteries. The tortuosity of the vessel precluded angioplasty, but she is treated with intra-arterial verapamil and papaverine. Later that day, her clinical status deteriorated to a GCS of 7 with a new onset of left hemiparesis. Unfortunately, her clinical status continued to deteriorate, and she died on post-bleed day 8 after CT showed widespread infarction.
Figure 15-8.
Computed tomography (CT) of the head performed on SAH days 2, 7 and 8. On day 2, thick subarachnoid blood is noted along with the site of the craniotomy after clipping. On day 7, a hypodensity in the right internal capsule is noted. On day 8, hypodensities are noted throughout the cerebral hemispheres affecting different vascular territories including the right and left middle cerebral arteries as well as the right posterior cerebral artery. (Reproduced with permission from Claassen J, Hirsch LJ, Kreiter KT, et al. Quantitative continuous EEG for detecting delayed cerebral ischemia in patients with poor grade subarachnoid hemorrhage. Clin Neurophysiol. 2004;115(12):2699-2710.)

Symptomatic vasospasm and the associated delayed cerebral ischemia (DCI) are common complications that occur in 20% to 40% of patients with SAH.14 A number of treatments are available, but making the diagnosis of DCI can be challenging, particularly in those with a limited neurological examination. Ideally, timely recognition of ischemia leads to interventions that prevent infarction. In addition to recording ictal activity, cEEG may detect decreased cerebral perfusion in the setting of vasospasm after SAH. EEG is very sensitive to ischemia and may reveal changes at a reversible stage of reduced cerebral blood blow and neuronal dysfunction (25-30 ml/100 g/min; Table 15-2). The raw EEG progresses through predictable stages with increasing degrees of hypoperfusion, including loss of fast activity, increased slowing, and background attenuation.15,16
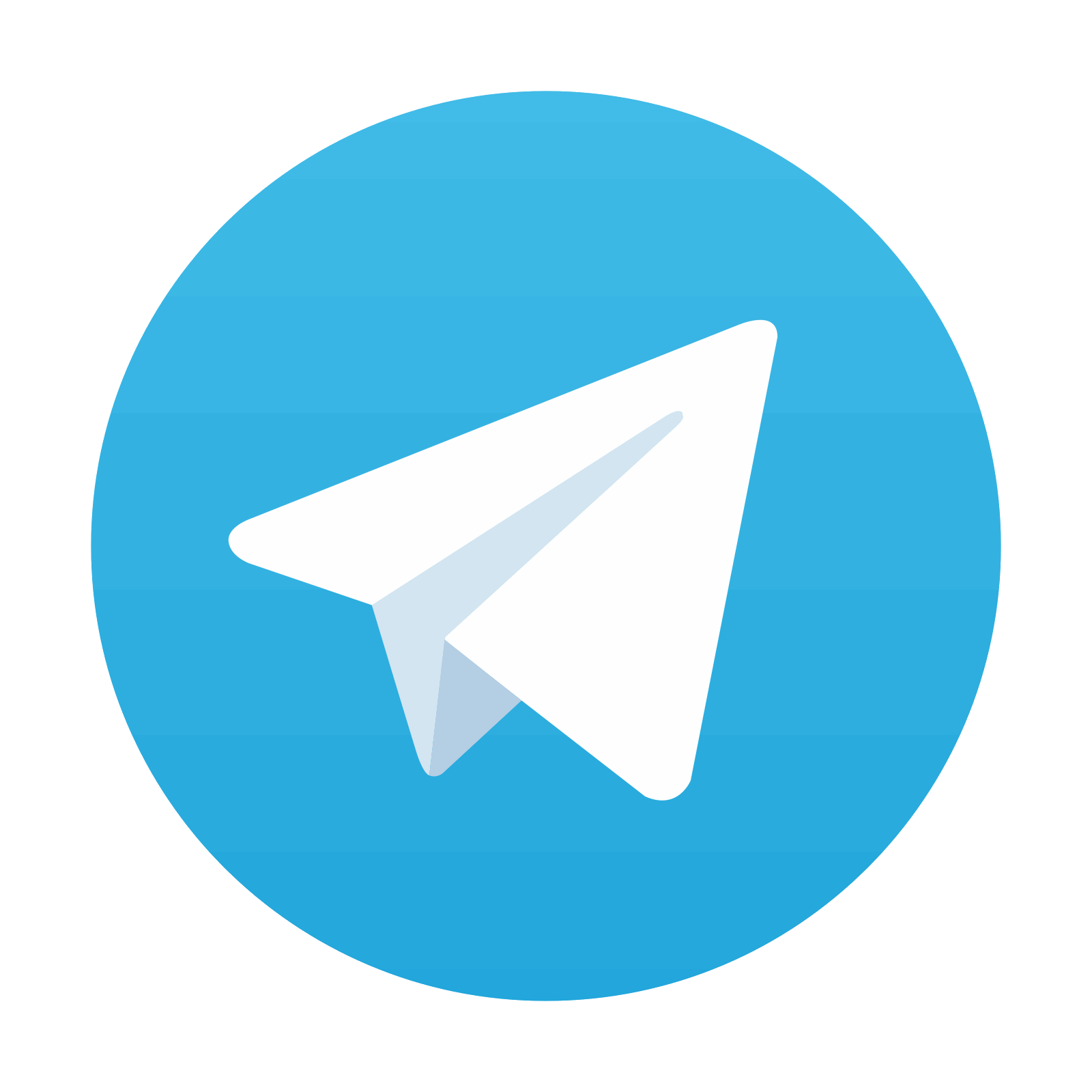
Stay updated, free articles. Join our Telegram channel

Full access? Get Clinical Tree
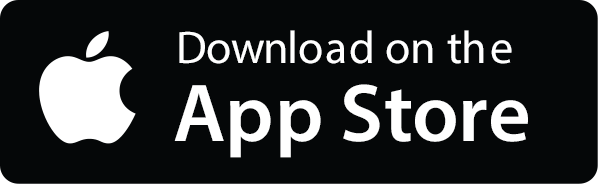
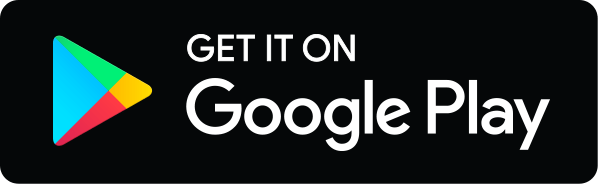