7 Cortical Resection in Frontal, Parietal, and Occipital Lobe Extratemporal epilepsy has been dichotomized from temporal lobe epilepsy historically in large part on account of the considerably more extensive experience and literature associated with the latter. The clinical importance of frontal, parietal, and occipital lobe epilepsy nonetheless is sufficient to warrant full consideration, exploration, and intervention in these epilepsies, and this chapter explores the preoperative evaluation, surgical treatment, and outcomes associated with the spectrum of these seizure disorders. Seizure disorders involving the frontal lobes have been thought more likely to be frequent, brief in duration, often nocturnal, and associated with status epilepticus and secondary generalization. In a study of the clinical expression of frontal lobe seizures using video electroencephalogram (EEG) analysis of more than 400 seizures of frontal lobe origin, Jobst et al found that indeed they tended to be frequent (7.1 per week), brief (mean duration 48.3 seconds), preponderantly nocturnal in 58% of patients, associated with status in more than half, and associated with generalized seizures in a quarter of patients.1 Categorization into various subtypes, reflecting the sometimes distinguishable features associated with the different regions of the frontal lobe, has also been popular, although the same analysis by Jobst et al showed that although such expressions of topography followed trends, such clinical features were often nonspecific. Seizures arising from the frontal convexity may present with focal clonic activity. Tonic seizures are most often associated with supplementary motor area seizure origin,1,2 but they can also be observed with frontopolar onset or with propagation to the supplementary motor area from other areas such as the medial parietal cortex.3 Seizures with oroalimentary automatisms suggestive of temporal lobe epilepsy as well as seizures with hyperactive automatisms can originate in the orbitofrontal region.1,4 Hyperactive or frenetic motor automatisms, however, are nonspecific with respect to location within the frontal lobe. Interestingly, two-thirds of frontal lobe seizures are associated with aura, most often nonspecific in nature.1,5 The traditional evaluation methods of history and neurological exam, imaging studies, scalp EEG, and neuropsychological testing are standard, and with current radiographic tools, including high field-strength magnetic resonance imaging (MRI), ictal and interictal single photon emission computed tomography (SPECT), positron emission tomography (PET), and magnetoencephalography (MEG), sufficient information to enable therapeutic surgical intervention may well be present. Interictal and ictal scalp EEG can be without definite abnormalities, especially in seizures originating at the medial frontal surface of the cortex.5 This can lead to misdiagnosis of frontal lobe seizures as nonepileptic psychogenic seizures.6 Neuropsychological testing may indicate dysfunction of executive function with impulsitivity and impersistence.7 However, neuropsychological testing can rarely lateralize or localize to subregions of the frontal lobes.7 MRI, ictal and interictal SPECT, PET, and MEG are additional diagnostic tools to localize the seizure onset zone. In those patients in whom imaging does not localize a potentially pathological substrate, further investigation by intracranial recording will likely be required. Should initial evaluation suggest nonlocalizable or multifocal epilepsy, further evaluation by an intracranial study can be avoided. EEG recording within the cranium has far greater sensitivity and specificity than its scalp-based counterpart. Its sensitivity will be considerably more spatially limited, of course, and coverage of the brain will always be incomplete. Judgment with respect to how and where an electrode array shall be developed is essential and ideally multidisciplinary. A variety of intracranial electrodes have been developed over many decades, each with respective advantages. Depth electrodes can record well from tissue at essentially any depth, and stereotactic placement can assure accurate placement, at least with respect to preoperative planning. Some groups have used these exclusively with great success, and the Talairach school is a good example.8 Subdural electrodes, in the form of strips, typically containing four to 12 contacts in a linear array, can be positioned through bur holes and better sample activity on the cortical surface. Subdural grid electrodes, consisting of anywhere from two to eight rows each containing two to eight contacts, obviously require a craniotomy for their insertion, but provide more complete coverage of a specific cortical surface; they also provide a convenient means by which to perform cortical mapping outside the operating room. In practice, most centers utilize all of these types of electrodes, usually in combination. An example of a typical implantation using depth, strip, and grid electrodes is seen in Fig. 7.1. Coverage of the frontal convexity, and particularly of the central region, is well accomplished using large subdural grid arrays; a typical array may consist of one or two 4 x 8 grids or an 8 x 8 grid. Subfrontal coverage is usually achieved using a 4 x 5 grid. Additional coverage, and particularly over the frontal pole, can be obtained with multiple 1 x 8 strips. Medial frontal coverage is less often accomplished using interhemispheric grids, on account of the more difficult exposure required, but in our experience it has been of great utility. Subdural grids in these locations also enable thorough extraoperative functional mapping. Alternatively, multiple depth electrodes may be employed to cover the entire lobe, including access to orbitofrontal, insular, and medial regions, with less extensive exposures. Additional types of electrodes, including minimally invasive transphenoidal and foramen ovale electrodes as well as PEG electrodes embedded in the cranium itself, have seen effective utilization as well. The efficacy of such intracranial electrode studies in general has been repeatedly demonstrated, and the associated morbidity has been low. A recent review of the experience at the Montreal Neurological Institute over more than two decades revealed the ability to support an indication for surgery in nearly 80% of patients and to exclude a surgical option in 16.5%.9 Fig. 7.1 Intracranial electrode implantation in a 22-year-old male with suspected frontal lobe epilepsy. (A) Computed tomography (CT) scan reformatted in midsagittal plane, demonstrating a double-sided 3 x 8 subdural grid electrode within the interhemispheric fissure. (B) CT scan sagittally reformatted in plane of left frontal depth electrodes. (C) CT thresholded to show intracranial electrode contacts co-registered with surface-rendering of MRI. (D) EEG recording showing seizure onset in region of contacts RFG28-RFG29 of the right frontal convexity subdural grid electrode. Resection of the seizure focus in the frontal lobe, as with other sites, offers the greatest chance of a seizure-free outcome. Published series of frontal resection report a wide range of good outcome,1,5,10–15 but the diagnostic tools available today enable success in the great majority of patients. Diagnostic imaging of the underlying pathological and pathophysiological substrate, often coupled with intracranial electrode investigation, enables tailored resection. The most common underlying pathological substrates for intractable frontal lobe epilepsy are focal cortical dysplasias, low grade tumors, and posttraumatic encephalomalacia.16 Subtle dysplasias are not always readily identifiable on routine MRI scanning and may require high field MRI scanning or special reformatting.17,18 In MRI-normal or nonlesional epilepsy, ictal SPECT, PET, and/or intracranial electrode investigation may also enable good surgical outcomes.19,20 Coregistration of preoperative studies with the operative field allows preoperative planning of these resective strategies and most importantly their accurate execution. In those instances with lesional substrates, completeness of resection of structural pathology has been associated with better seizure outcome.21 Actual resection follows standard microsurgical technique. Adequate exposure of the extent of intended cortical resection is essential, and in those patients in whom eloquent cortex—subserving language and motor function in particular with respect to the frontal lobe—is either involved or adjacent to the resection, recognition of that functional localization is critical. The gold standard of functional mapping has been intraoperative mapping by electrical stimulation technique. For language, this requires an awake craniotomy; for motor function, the ability to map in the anesthetized patient is commonplace. Patients in whom an intracranial electrode investigation has been performed using subdural grid electrodes may be mapped extraoperatively using those same grids, a practice advantaged by the greater ease and less constrained nature of the setting. Traditionally 5-second long 50 Hz stimulation is used for functional mapping intra- and extraoperatively. Due to the propensity for seizures and after-discharges functional motor mapping with high frequency pulse trains and electromyography (EMG) control can be of advantage for motor mapping. Co-registration of imaging studies, including both structural and functional investigations, enables additional localization to be incorporated into the surgical field. Integration of a surgical plan including identification of both the tissue to be resected as well as that to be preserved delineates the cortical margins of the resection. Corticotomy incisions along the boundary take into account the sulcal topography, and attendance is given to the preservation of vascular structures that may be en passage. The most compelling instance of the latter in frontal lobe resection is that of medial resections, such as that involving the supplementary motor area, where anterior cerebral artery supply to regions posterior to the resection cannot be sacrificed. Resection is generally performed with the aid of the operating microscope, and a combination of en bloc resection (valuable in pathological diagnosis for both therapeutic and prognostic purposes) and subpial dissection is often employed for the removal of tissue. Resections into the ventricular system are avoided, if possible, for minimalization of morbidity including hydrocephalus and subdural hygromas. Hemostasis and closure follow standard surgical techniques. A considerable surgical experience has now accumulated in the literature, and from these series good outcomes (Engel class I or II) range from as low as 26% to as high as 80%.1,5,12,14,21–25 Patient selection represents the variable most responsible for such variation, but recent surgical cases have tended to be at the higher end of that range.1,15,25 Other predictors for a good surgical outcome have been identified. Focal ictal b discharges on scalp EEG,16,21 focal confined lesions,14,24 and slow intracranial spread patterns26 have been identified as favorable prognostic factors. Unilateral frontal resection is generally well tolerated, with low surgical and neurological morbidity.27 Supplemental motor area (SMA) resections are often accompanied by well-described post-resection syndrome characterized by decreased spontaneous motor function of the contralateral limbs and, with language-dominant side resection, expressive speech.28,29 These may last from several days to several weeks but rarely present long-term difficulties. Various nonresective surgical strategies have also evolved. There are several reasons why these might be employed, but the most common indications have been in instances where a seizure focus could not be identified or where a focus involved eloquent, nonresectable tissue. Principles of less invasiveness or potentially lower risk may also play a role in selection of a particular procedure. Corpus callosotomy evolved from the observation that generalization of seizure activity used the callosal commissure as the route of propagation. Seizure-free outcome is not a realistic expectation of the operation, but as a palliative procedure when others are either not options or have failed, it remains a valuable option.30,31 Of those seizure types most likely to respond, drop attacks have consistently been singled out. As the majority of these have been presumably of frontal lobe origin, anterior section alone may be effective in this application.12 Surgery has been facilitated by standard microsurgical technique and more recently image guidance. The interhemispheric approach, with the patient positioned either supine or lateral (with gravity assisting retraction of the hemisphere on the side of approach), is now widely used for many other procedures, notably for resection of third ventricular tumors, and will be familiar to most neurosurgeons. Actual callosal division is best performed in the midline, enabling avoidance of ventricular entry, protection of the fornix, and assurance of commissural division. Blunt or aspiration technique is readily performed, and most centers advocate division of the anterior two-thirds to three-quarters of the callosum as an initial procedure as this tends to optimize the efficacy/morbidity ratio. Other commissural structures, targeted in the procedure’s earliest descriptions, are no longer divided. The morbidity of anterior section is low, and the neuropsychological consequences rarely exceed the benefits in this patient population.32 As a palliative procedure, Engel classification of outcome is less useful, but recent surgical series have shown 48 to 78% a significant reduction in seizures with callostomy.30,33 In cases in which seizure onset has been localized but lies within unresectable, functional cortex, multiple subpial transection (MST) has been advocated. Technically, the procedure has not changed greatly from its earliest descriptions, with division of the cortical thickness across the width of the gyrus involved with repeat transections at 5-mm inter-vals.34–36 Most reports of MST have combined the technique with resection, rendering interpretation of its efficacy difficult.37,38 A meta-analysis of several centers reported an excellent outcome in 68 to 87% if subpial transections were combined with resection and in 62 to 71% if MST alone was performed.38 Motor and speech function are generally preserved, but deficits were reported in 19% of patients in the above mentioned meta-analysis.35,38 Vagal nerve stimulation (VNS) not surprisingly given its relative noninvasiveness has been widely utilized in difficult cases in which the seizure-free allure of resective procedures is not applicable. Technically, the procedure requires placement of bipolar electrode coils around the vagal nerve in the neck (usually performed on the left side, in an attempt to minimize the risk of cardiac arrhythmia) and then tunneling the electrode to a subcutaneous pocket below the left clavicle where the pulse generator is implanted. Battery life between 3 and 6 years can be expected, and battery replacement requires only a minor, outpatient procedure. In general, 50% of patients have 50% fewer seizures with VNS implantation.39 There is no particular epilepsy syndrome that responds more favorably than others, but VNS is effective in frontal lobe epilepsy. Deep brain stimulation has been performed in much smaller numbers generally as part of investigational trials. The equipment and methodology have been directly adopted from surgery for movement disorders and utilize standard stereotactic procedures. Numerous targets have been implanted, the most widely used now being the subthalamic nucleus and the anterior nucleus of the thalamus. Chabardes et al has reported a good outcome in 3 of 5 patient with stimulation of the subthalamic nucleus.40 Other groups report good results with stimulation of the anterior nucleus of the thalamus and the centromedian nucleus of the thalamus.41,42 An implantation effect with improvement of seizures regardless of whether the stimulator is on or off has also been observed.41,43 A large-scale randomized study is currently underway to evaluate the utility of stimulation of the anterior thalamic nucleus in localization-related epilepsy.44 Theoretically, stimulation techniques might take advantage of the relatively infrequent occurrence of seizure activity and the frequent ability to detect early the EEG activity associated with the event. Such a conceptual approach underlies NeuroPace, Inc.’s Responsive Neruostimulator (RNS™) system (NeuroPace, Inc., Mountain View, CA), which is currently being evaluated in a multicenter pivotal clinical trial.45 This system presently consists of two depth or subdural strip electrodes and a cranially implanted, programmable pulse generator with the ability to run a seizure-detection algorithm, and when triggered through such a detection the system sends an electrical stimulus to abort the electrographic seizure. Electrical stimulation has been shown to suppress or alter seizures in patients with subdural electrodes during a preoperative intracranial evaluation,46 and results of a Phase I and II trial have been promising. Without the benefit of immediate motor behavior, seizure onset in the parietal lobe can be less obvious. Further, rapid spread of the epileptic discharge to the temporal or frontal lobes and subsequent first recognized seizure expression reflective of those secondary sites may be misleading. Nevertheless, early sensory symptoms may be highly suggestive of localization. Somatosensory seizures may have their origin in any portion of the parietal cortex. Most commonly but not exclusively contralateral, any or multiple sensory modality may be involved. Elementary paresthesias are most common47,48 with initial involvement of a limb or face and often with spread to contiguous regions of the body, analogous to the well-recognized Jacksonian march of motor seizures. Actual motor activity has been reported to occur in approximately half of such sensory seizures.48–50 Seizures expressed as the sensation of pain, although long recognized, were often thought to be uncommon until Mauguiere and Courjon in 1978 reported them to be the second most common type of somatosensory seizures.48,51 Thermal sensations, usually described as burning but occasionally as cold, have also been reported.49,52 Other seizure manifestations of parietal epilepsy include ideomotor apraxia (ascribed to involvement of the second sensory area) and sexual phenomena (also associated with the temporal and frontal lobes, but when arising from the parietal lobe believed to represent involvement of the para-central lobule adjacent to the primary somatosensory cortical representation for the genitalia). Disturbances of body image have been described in association with the inferior parietal lobule and the superior aspect of the postcentral gyrus. Ictal anosognosia, apraxia, acalculia, alexia, aphemia, and confusional states may be associated with parietal epilepsy.53 Gustatory seizures may be either temporal or parietal in origin, with the parietal operculum involved in the latter. All of these seizures may progress to clonic or tonic motor activity depending on the intracranial spread pattern.54,55 Just as in the frontal lobe epilepsies, evaluation of the medically intractable seizure patient with possible parietal seizure onset may require an intracranial implant for more complete characterization of the seizure onset and possible functional mapping (see Fig. 7.2). The methodology for such implantation in the parietal region is the same as that previously described. However, without a clear lesion it can be difficult to determine a seizure’s onset zone in parietal lobe epilepsy as seizures manifestations can be subtle and often a result of propagation.
Frontal Lobe Epilepsy
Diagnosis
Intracranial Investigation
Operative Techniques
Nonresective Strategies
Parietal Lobe Epilepsy
Diagnosis
Operative Techniques
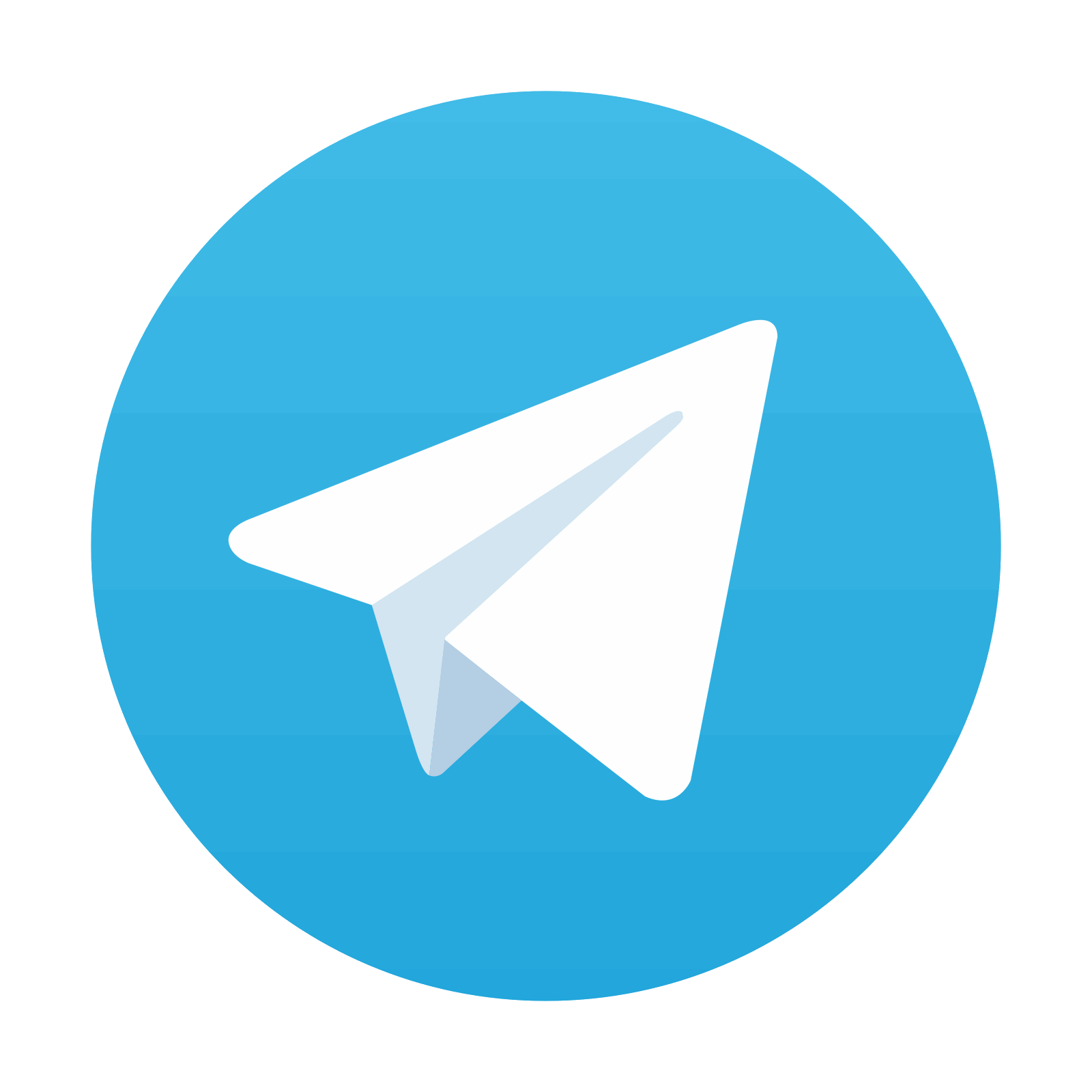
Stay updated, free articles. Join our Telegram channel

Full access? Get Clinical Tree
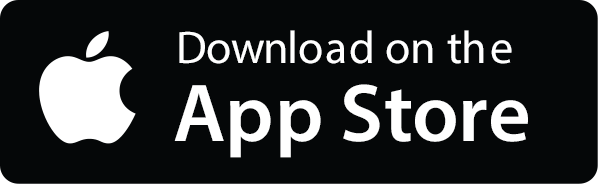
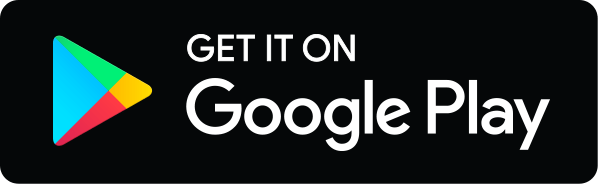