11 Corticography Detecting the intrinsic electrical activity of the brain remarkably followed, rather than preceded, electrically stimulating the brain. In the late 19th century, individuals such as Richard Caton, Gustav Fritsch, and Edward Hitzig electrically stimulated the cerebral cortex of man and animals, and began to demonstrate that such stimulation could induce motor activity. It was soon apparent that cortex capable of transducing such stimuli into motor actions was surprisingly localized. By the early 1900s, some surgeons were reporting the use of electrical stimulation to map the motor cortex.1 In the next decades, this technique spread among neurosurgeons, with Wilder Penfield being one of the early pioneers of mapping wider expanses of cortex via stimulation. In parallel, in 1920s Germany, Hans Berger began to report his recordings of the spontaneous electrical activity of the brain using scalp electrodes, now known as electroencephalography (EEG).2 Berger even performed the first direct cortical recordings with silver needle electrodes on patients who had undergone craniotomies for tumors. The extension from intraoperative stimulation to direct intraoperative cortical recording (electrocorticography, ECoG) was quickly made.3 Also in the early 1930s, the aberrant electrical activity detectable on scalp EEG during seizures and even interictally was noted by neurologists such as Gibbs et al.4 Penfield recruited the neurologist and electroencephalographer Herbert Jasper to the Montreal Neurological Institute, where these two men took their place among the pioneers who synthesized the techniques of EEG, ECoG, and brain stimulation for the examination of cortex in what was to become standard practice worldwide as functional surgery for epilepsy.5 ECoG reached what was to be its apogee in utility, until recent years, in the early 1950s. Early resections for temporal lobe epilepsy had focused on the lateral temporal cortex.6 However, these early series of surgical outcomes were decidedly underwhelming, with success rates of around 50% of patients being rendered seizure free. Stimulation and ECoG played a pivotal role in demonstrating that stimulation of mesial structures (amygdala and hippocampus) could recapitulate the electrographic and clinical manifestations of seizures, and that epileptiform discharges were present in these mesial temporal structures. After this discovery, resections were expanded to include the mesial temporal structures as well, specifically the amygdala and hippocampus, resulting in a much greater proportion of seizure freedom for patients.7 Once this crucial role of mesial structures in temporal lobe epilepsy was realized, the role for intraoperative ECoG waned. As early as 1958, prominent neurosurgeons such as Murray Falconer suggested that with “adequate” preoperative studies, intraoperative ECoG was “unnecessary” for the surgical treatment of temporal lobe epilepsy.8 Since the 1950s, ECoG and functional brain stimulation continue to be used during evaluation for epilepsy surgery, as well as to map brain function during surgery and other treatments for tumors, movement disorders, or other brain disorders. Cortical recording has also extended beyond the operating room, employed via chronic implantation of sub-dural electrodes for localization of epileptic networks spanning days to weeks. In addition, recording of field potentials, small collections of neurons (multi-unit), and even single neurons (single-unit) is performed routinely during placement of brain stimulating electrodes to treat an expanding array of brain disorders. This chapter, however, will be restricted to intraoperative ECoG, focusing on use in epilepsy surgery to guide resection. The use of intraoperative ECoG for epilepsy surgery has certainly waxed and waned over the years, and practices vary greatly from center to center worldwide. It is difficult to be optimistic about its traditional role in epilepsy surgery because the only consensus about its use is that the evidence supporting it is divergent, though at this chapter’s end we will discuss new exciting applications of these techniques that may herald their return to mainstream clinical practice. The bulk of this chapter will focus on specific advice for implementation, offering a fair exposition of the evidence regarding the role of ECoG during routine clinical practice, and leaves the decision on its applicability to the individual surgeon, for each particular case. Many facets of intraoperative planning must be considered and coordinated prior to surgery. Choice of anesthetic needs to be made with some forethought, depending on such general factors as need for awake-patient testing. More specifically, choice of anesthetic must be coordinated with the goals of ECoG. Anesthetics have drastically different effects on ECoG. Some decrease the interictal epileptiform discharge (IED) frequency, whereas others increase the rate, some even increasing the likelihood of seizure. The effect depends significantly on the dose of the anesthetic (as well as other factors such as CO2 tension), often with increased discharge rate at moderate dosages, then decreased rate as global cerebral activity is generally depressed. Dramatic depression of discharge rates would obviously hamper their detection and localization by ECoG. On the other hand, agents that increase IEDs have been advocated to shorten the time of required monitoring. However, one must recognize some studies have raised suspicion that the discharges elicited by these “activating” agents may be falsely localizing. Of note, Modica and colleagues present an overview of much contradictory evidence on the pro- and anticonvulsant effects of anesthetics, which, although dated, remains useful because the evidence remains conflicting.9 The practice of purposefully activating the EEG with anesthetic agents to enhance recordings of interictal epileptiform activity has fallen out of favor in recent years. Procedures in which EEG recording is essential in the operating room are usually done with agents such as fentanyl, nitrous oxide, and on some occasions, with propofol as an ancillary agent, provided it is turned off for >15 minutes prior to cortical mapping/ECoG. In its early years, traditional ECoG employed spherical metal electrodes supported by thin, flexible metal cylinders mounted to a frame that was typically clamped to the patient’s (exposed) calvaria. This set-up had the advantage of easy adjustment of electrode position and ease of visualization of underlying anatomy. Disadvantages included the fact that the relative location of electrodes were not fixed or standardized, leading to ambiguity of amplitude measurements with bipolar montages. These electrodes could be reused with appropriate sterilization. Currently, most ECoG is performed with strips or grids of inert metal disk electrodes embedded at fixed intervals in flexible transparent Silastic sheets. Typical grids have platinum or stainless steel electrodes of a diameter of 4.0 mm (exposed diameter 2.3 mm), in a square lattice separated by 10.0 mm center-to-center distance. Dimensions of subdural electrode strips and grids vary, depending upon the coverage required, and usually range from one row of four contacts (i.e., 1 x 4) to sheets of 64 electrodes in a single square array of eight contacts in each row and column (i.e., 8 x 8) (Fig. 11.1), to custom grid arrays in asymmetric sizes, depending upon the application. Choosing the right electrode configuration permits adequate movement (with vascular pulsations) and permits underlying anatomy to be fairly visualized. However, precise placement at multiple anatomical landmarks is not generally possible. Subdural strip or grid electrodes are used only once (disposable), and as such can become expensive for frequent use. It is also important to note that depth electrodes can be used to map epileptiform activity and for brain stimulation. As in the calculations described below, electrode surface area, impedance, and placement are key to interpreting information gathered by passive recording and through electrical stimulation. Because ECoG is generally performed when localization of the epileptic network is nebulous, a large extended craniectomy is often required, unless stereotaxic placement of depth electrodes is used, which can be done through bur holes. After opening the dura and exposing the pial surface, subdural electrode strips or grids are placed with visual guidance over the regions/lobes of interest. A reference electrode is typically placed on the skin of the neck in the midline, though contacts on the grid or additional strips may be used as an electrical reference and isoground. Traditionally, the electroencephalographer does not significantly alter the electrical characteristics of the recording apparatus from routine scalp EEG recordings, though more recent research suggests that mapping of high-frequency oscillations may be of some utility (see section on non-lesional extratemporal epilepsy below). For most current digital data acquisitions, the pre-digitization analog low-pass (anti-aliasing) filter remains at 70 to 100 Hz. Most centers use digitization rates of 200 to 400 samples per second (per channel). The absolute voltages recorded (relative to the “machine” reference) are not so different from typical scalp recordings to necessitate changes to digitizer voltage input scale. On the other hand, being recorded directly from cortex and cleared of many sources of artifact, the relative voltages (especially in bipolar montages) are much larger, on the order of hundreds of microvolts (μV). Therefore, the voltage scale of the display must be adjusted accordingly, and the display sensitivity is usually decreased to 50 to 100 μV per millimeter (from 7 μV per millimeter on typical scalp EEG). As with scalp EEG, attention must be paid to electrode placement, particularly to having good contact with the brain surface, no air bubbles beneath the electrodes, and electrodes of importance should be kept off blood vessels, to insure good signal quality. Electrode impedance should be less than or equal to 10 kΩ, and preferably in the 5 to 10 kΩ range. Fig. 11.1 An example of an electrode grid, arranged in a square lattice 8 x 8, with labeled contacts 1 through 64. In typical situations, there will be many electrodes simultaneously implanted during evaluation for epilepsy surgery, in cases where localization of epileptic networks is the motivation for electrode placement and ECoG, more than can be usefully displayed at one time. Montages should be created in which spatially close electrodes (or electrode pairs, in bipolar montages) are visually close as well. Montages can be created that, for example, display electrodes (or electrode pairs) over individual lobes. As in scalp EEG, referential montages are useful in spatially restricted events of interest, whereas bipolar montages can be very useful in identifying relative maxima of spatially broad events. As with other types of electroencephalography, montages should be balanced and symmetrical when possible, so that electrode distances are comparable and signal amplitude is comparable from channel to channel. A flowchart representing the authors’ basic protocol for recording intraoperative ECoG is shown in Fig. 11.2. The surgeon must be aware of (and pay special attention to) the stimulation protocol to prevent irreversible injury to the cortex. Several parameters are key. Some basic relations to consider:
Equipment and Procedure
Preoperative Planning
Inhaled Anesthetics
Analgesics (Opioids)
Sedatives
Recommendations
Recording
Stimulation
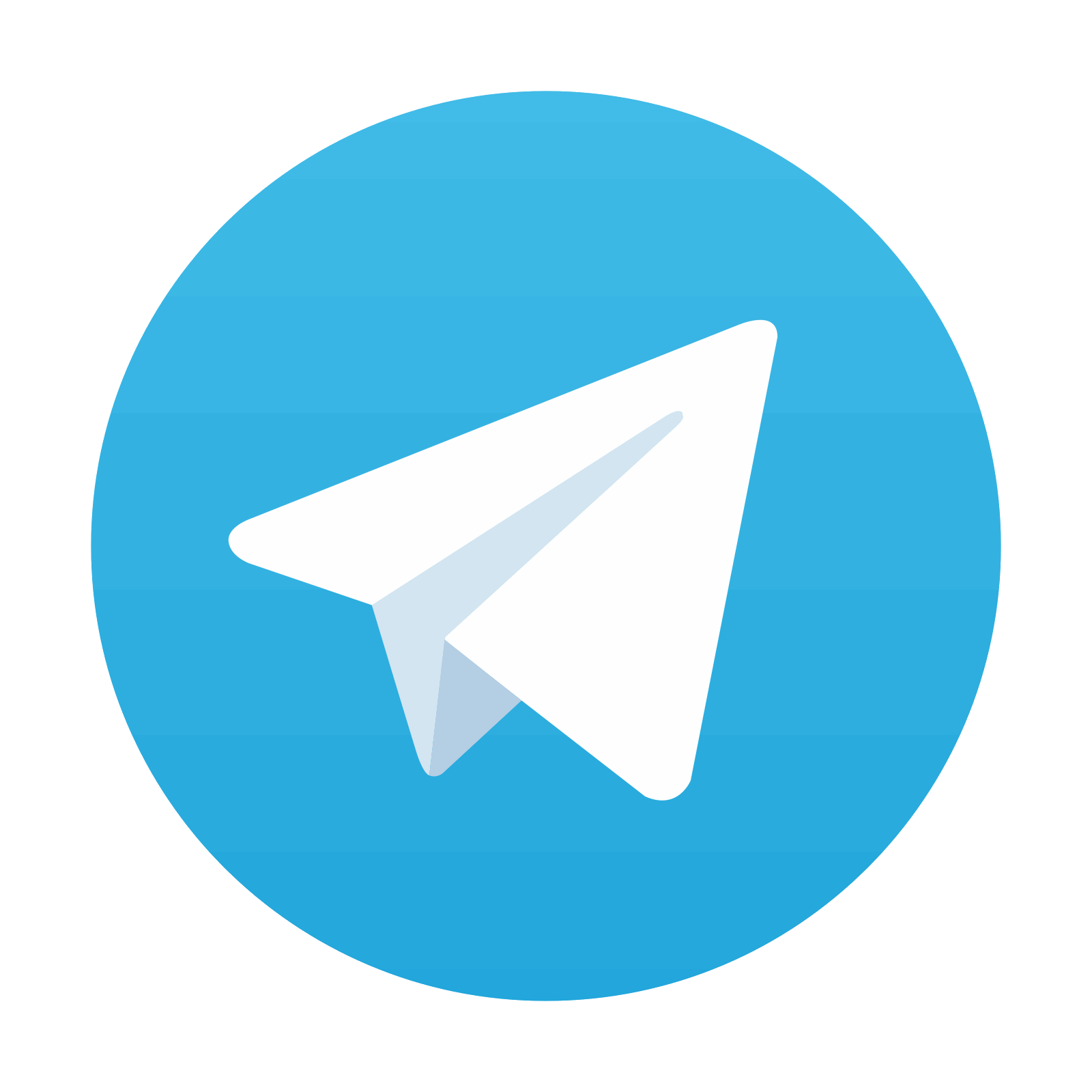
Stay updated, free articles. Join our Telegram channel

Full access? Get Clinical Tree
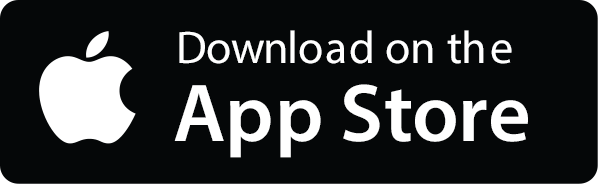
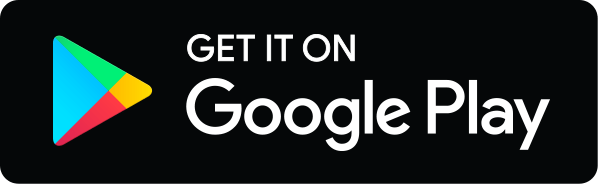