Origin, Components, and Functions
▶Fig. 4.2 is a schematic dorsal view of the brainstem, in which the motor and parasympathetic cranial nerve nuclei are shown on the right and the somatosensory and special sensory nuclei are shown on the left. Lateral views showing the anatomical relations of the motor and parasympathetic nuclei, and of the somatosensory and special sensory nuclei, are found in ▶Fig. 4.3 and ▶Fig. 4.4, respectively.
Fig. 4.2 Cranial nerve nuclei, dorsal view (schematic drawing). The somatosensory (blue) and special sensory (light blue) nuclei are shown on the left side of the figure, and the motor (red) and parasympathetic (green) nuclei on the right. The motor nuclei of the branchial arch nerves are shown in bright red.
The origin, components, and function of the individual cranial nerves are listed in ▶Table 4.1. ▶Fig. 4.5 provides a synoptic view of the sites of emergence of all 12 cranial nerves from the brainstem, their functional components, and their peripheral sites of origin and termination. All 12 cranial nerves are seen in the figure, from I (olfactory nerve) to XII (hypoglossal nerve); it should be borne in mind, however, that the second cranial “nerve”—the optic nerve—is actually not a peripheral nerve at all, but rather a tract of the central nervous system.
Recall that spinal nerve fibers can be classified as somatic afferent, somatic efferent, visceral afferent, and visceral efferent. The classification of cranial nerve fibers is a little more complicated, for two reasons. Some of the cranial nerve fibers are special sensory fibers arising from the sense organs of the head (vision, hearing, taste, smell). Furthermore, some of the efferent cranial nerve fibers arise in nuclear areas that are embryologically derived from the branchial arches; these fibers innervate muscles of branchial origin.
There results a sevenfold classification of cranial nerve fibers, as follows:
Somatic afferent fibers (pain, temperature, touch, pressure, and proprioceptive sense from receptors in the skin, joints, tendons, etc.).
Visceral afferent fibers, which carry impulses (pain) from the internal organs.
Special somatic afferent fibers carrying impulses from special receptors (eye, ear).
Special visceral afferent fibers carrying impulses related to taste and smell.
General somatic efferent fibers carrying motor impulses to the skeletal musculature (oculomotor, trochlear, abducens, and hypoglossal nerves).
Visceral efferent fibers innervating the smooth muscles, the cardiac musculature, and the glands (both sympathetic and parasympathetic).
Special branchial efferent fibers innervating muscles that are derived from the mesodermal branchial arches, i.e., the motor portions of the facial nerve (second branchial arch), glossopharyngeal nerve (third branchial arch), and vagus nerve (fourth branchial arch and below).
The cranial nerves exit from the skull through the openings (foramina, fissures, canals) depicted on the left side in ▶Fig. 4.6. The cut-off nerve stumps in their corresponding openings are shown on the right.
Olfactory System (CN I)
The olfactory pathway (▶Fig. 4.7 and ▶Fig. 4.8) is composed of the olfactory epithelium of the nose, the olfactory nerve (CN I), the olfactory bulb and tract, and a cortical area (the paleocortex) extending from the uncus of the temporal lobe across the anterior perforated substance to the medial surface of the frontal lobe under the genu of the corpus callosum.
The olfactory epithelium occupies an area of about 2 cm2 in the roof of each nasal cavity, overlying portions of the superior nasal concha and of the nasal septum. It contains receptor cells, supportive cells, and glands (Bowman’s glands) that secrete a serous fluid, the so-called olfactory mucus, in which aromatic substances are probably dissolved. The sensory cells (olfactory cells) are bipolar cells whose peripheral processes terminate in the olfactory hairs of the olfactory epithelium.
Olfactory nerve and olfactory bulb. The central processes (neurites) of the olfactory cells coalesce into bundles containing hundreds of unmyelinated fibers surrounded by a Schwann-cell sheath. These fila olfactoria, about 20 on either side, are, in fact, the olfactory nerves (CN I is thus composed of peripheral nerve fibers, but is not a single peripheral nerve in the usual sense). They pass through small holes in the cribriform (“sievelike”) plate and enter the olfactory bulb, where they form the first synapse of the olfactory pathway. Although it is not physically located in the cerebral cortex, the olfactory bulb is actually a piece of the telencephalon. Within it, complex synapses are made onto the dendrites of mitral cells, tufted cells, and granule cells.
Olfactory pathway. The first neuron of the olfactory pathway is the bipolar olfactory cell; the second neurons are the mitral and tufted cells of the olfactory bulb. The neurites of these cells form the olfactory tract (second neuron), which lies adjacent to and just below the frontobasal (orbitofrontal) cortex. The olfactory tract divides into the lateral and medial olfactory striae in front of the anterior perforated substance; another portion of it terminates in the olfactory trigone, which also lies in front of the anterior perforated substance. The fibers of the lateral stria travel by way of the limen insulae to the amygdala, semilunar gyrus, and ambient gyrus (prepyriform area). This is the site of the third neuron, which projects to the anterior portion of the parahippocampal gyrus (Brodmann area 28, containing the cortical projection fields and association area of the olfactory system). The fibers of the medial stria terminate on nuclei of the septal area below the genu of the corpus callosum (subcallosal area) and in front of the anterior commissure. Fibers emerging from these nuclei project, in turn, to the opposite hemisphere and to the limbic system. The olfactory pathway is the only sensory pathway that reaches the cerebral cortex without going through a relay in the thalamus. Its central connections are complex and still incompletely known.
Connections of the olfactory system with other brain areas. An appetizing aroma excites the appetite and induces reflex salivation, while a foul smell induces nausea and the urge to vomit, or even actual vomiting. These processes also involve the emotions: some odors are pleasant, while others are unpleasant. Such emotions probably come about through connections of the olfactory system with the hypothalamus, thalamus, and limbic system. Among its other connections, the septal area sends association fibers to the cingulate gyrus.
The main connections of the olfactory system with autonomic areas are the medial forebrain bundle and the striae medullares thalami (▶Fig. 6.9). The medial forebrain bundle runs laterally through the hypothalamus and gives off branches to hypothalamic nuclei. Some of its fibers continue into the brainstem to terminate in autonomic centers in the reticular formation, the salivatory nuclei, and the dorsal nucleus of the vagus nerve. The striae medullares thalami terminate in the habenular nucleus; this pathway then continues to the interpeduncular nucleus and the brainstem reticular formation (▶Fig. 6.9).
Disturbances of smell. Disturbances of smell can be classified as either quantitative or qualitative. Quantitative disturbances of smell include hyposmia (diminished smell) and anosmia (absence of smell). They are always due either to peripheral damage of the olfactory nerve (e.g., because of rhinitis, trauma with disruption of the nerve fibers in the cribriform plate, or side effects of medication) or to central damage of the second neuron in the olfactory bulb and/or tract (olfactory groove meningioma is a classic cause). Qualitative disturbances of smell, also known as parosmias, may consist of an unpleasant cacosmia (e.g., fecal odor) or of hyperosmia (abnormally intense smell). They are usually due to central dysfunction, as in temporal lobe epilepsy.
Visual System (CN II)
Visual Pathway
The retina (▶Fig. 4.9a) is the receptor surface for visual information. Like the optic nerve, it is a portion of the brain, despite its physical location at the periphery of the central nervous system. Its most important components are the sensory receptor cells, or photoreceptors, and several types of neurons of the visual pathway. The deepest cellular layer of the retina contains the photoreceptors (rods and cones); the two more superficial layers contain the bipolar neurons and the ganglion cells.
Rods and cones. When light falls on the retina, it induces a photochemical reaction in the rods and cones, which leads to the generation of impulses that are ultimately propagated to the visual cortex. The rods were long thought to be responsible for the perception of brightness and for vision in dim light, while the cones were thought to subserve color perception and vision in bright light. More recent research, however, has cast doubt on these hypotheses. The underlying mechanisms of these processes are probably much more complex but cannot be discussed here in any further detail.
The fovea is the site of sharpest vision in the retina and contains only cones, which project onto the bipolar cells of the next neuronal layer in a one-to-one relationship. The remainder of the retina contains a mixture of rods and cones.
The retinal image of a visually perceived object is upside-down and with left and right inverted, just like the image on the film in a camera.
Optic nerve, chiasm, and tract. The retinal bipolar cells receive input onto their dendrites from the rods and cones and transmit impulses further centrally to the ganglion cell layer. The long axons of the ganglion cells pass through the optic papilla (disk) and leave the eye as the optic nerve, which contains about 1 million fibers. Half of these fibers decussate in the optic chiasm: the fibers from the temporal half of each retina remain uncrossed, while those from the nasal half of each retina cross to the opposite side (▶Fig. 4.9b).
Thus, at positions distal (posterior) to the optic chiasm, fibers from the temporal half of the ipsilateral retina and the nasal half of the contralateral retina are united in the optic tract.
A small contingent of optic nerve fibers branches off the optic tracts and travels to the superior colliculi and to nuclei in the pretectal area (see ▶Fig. 4.26). These fibers constitute the afferent arm of various visual reflexes, and, in particular, of the important pupillary light reflex, which will be discussed further below.
Lateral geniculate body, optic radiation, and visual cortex. The optic tract terminates in the lateral geniculate body, which contains six cellular layers. Most of the optic tract fibers end here, forming synapses with lateral geniculate neurons. These, in turn, emit fibers that run in the hindmost portion of the internal capsule (▶Fig. 3.2) and then form a broad band that courses around the temporal and occipital horns of the lateral ventricle, the so-called optic radiation (of Gratiolet; see ▶Fig. 4.10). The fibers of the optic radiation terminate in the visual cortex, which is located on the medial surface of the occipital lobe, within, above, and below the calcarine fissure (Brodmann area 17). Fibers derived from the macula occupy the largest area of the visual cortex (▶Fig. 4.11). Area 17 is also known as the striate cortex because it contains the stripe of Gennari, a white band composed of horizontally running fibers, which can be seen with the naked eye in sectioned anatomical specimens.
Somatotopic organization of the visual pathway. Although the fibers of the visual pathway partially decussate in the optic chiasm, a strict point-to-point somatotopic organization of the individual nerve fibers is preserved all the way from the retina to the visual cortex (▶Fig. 4.11).
Visual information is transmitted centrally as follows. An object located in the left visual field gives rise to images on the nasal half of the left retina and the temporal half of the right retina. Optic nerve fibers derived from the nasal half of the left retina cross to the left side in the optic chiasm to join the fibers from the temporal half of the right retina in the right optic tract. These fibers then pass to a relay station in the right lateral geniculate body, and then by way of the right optic radiation into the right visual cortex. The right visual cortex is thus responsible for the perception of objects in the left visual field; in analogous fashion, all visual impulses relating to the right visual field are transmitted through the left optic tract and radiation into the left visual cortex (▶Fig. 4.9b).
Visual fibers derived from the macula are found in the temporal portion of the optic disk and in the central portion of the optic nerve (▶Fig. 4.12). Damage to these fibers can be seen by ophthalmoscopy as atrophy of the temporal portion of the disk (temporal pallor).
Lesions along the Visual Pathway
Optic nerve lesions. The optic nerve can be damaged at the papilla, in its anterior segment, or in its retrobulbar segment (i.e., behind the eye). Lesions of the optic disk (e.g., papilledema, caused by intracranial hypertension and by a variety of metabolic disorders) can be seen by ophthalmoscopy. Lesions of the anterior segment of the optic nerve are often due to vasculitis (e.g., temporal arteritis). Retrobulbar lesions are a cardinal finding in multiple sclerosis (retrobulbar neuritis). Lesions at any of these sites can cause long-term impairment or loss of vision in the affected eye. Brief episodes of visual impairment in a single eye, lasting from a few seconds to several minutes (“transient monocular blindness”), are designated amaurosis fugax and are generally caused by microembolism into the retina. In such cases, the ICA is often the source of emboli and should be investigated for a possible stenosis.
Lesions of the optic chiasm. Lesions of the optic chiasm, such as those produced by a pituitary tumor, craniopharyngioma, or meningioma of the tuberculum sellae, generally affect the decussating fibers in the central portion of the chiasm. The result is partial blindness for objects in the temporal half of the visual field of either eye, i.e., bitemporal hemianopia (the “blinker phenomenon,” where the reference is to a horse’s blinkers). Fibers in the lower portion of the chiasm, derived from the lower portion of the chiasm, are commonly affected first by such processes; thus, bitemporal upper quadrantanopsia is a common early finding. Only color vision may be impaired at first.
Less commonly, however, a lesion of the chiasm can cause binasal hemianopia, e.g., when a tumor has grown around the chiasm and compresses it from both sides (thus mainly affecting the laterally located, uncrossed fibers derived from the temporal halves of the two retinas, which are responsible for perception in the nasal hemifield of each eye). Aneurysms of the ICA and basilar meningitis are further possible causes, but the binasal hemianopia in such cases is rarely pure.
Bitemporal and binasal hemianopia are both termed heteronymous, because they affect opposite halves of the visual fields of the two eyes: the former affects the right hemifield of the right eye and the left hemifield of the left eye, while the latter affects the left hemifield of the right eye and the right hemifield of the left eye.
Optic tract lesions. Optic tract lesions, on the other hand, cause homonymous hemianopia, in which the hemifield of the same side is affected in each eye. When the fibers of the right optic tract are interrupted, for example, no visual impulses derived from the right side of either retina can reach the visual cortex. The result is blindness in the left half of the visual field of each eye (▶Fig. 4.9b and ▶Fig. 4.19c). Optic tract lesions are usually caused by a tumor or basilar meningitis, and less often by trauma.
Because an interruption of the optic tract also affects the optic nerve fibers traveling to the superior colliculi and to the pretectal area, it impairs the pupillary light reflex in response to light falling on the side of the retina ipsilateral to the lesion. In theory, this hemianopic light reflex test could be used to distinguish optic tract lesions from lesions located more distally in the visual pathway. In practice, however, it is very difficult to shine a light onto one half of the retina exclusively, and the test is of no use in clinical diagnosis.
Lesions of the optic radiation. A lesion affecting the proximal portion of the optic radiation also causes homonymous hemianopia, which, however, is often incomplete, because the fibers of the optic radiation are spread over a broad area (▶Fig. 4.9). Homonymous upper quadrantanopsia implies a lesion in the anterior temporal lobe, affecting the part of the radiation known as Meyer’s loop (▶Fig. 4.10). Homonymous lower quadrantanopia implies a lesion in the parietal or occipital portion of the optic radiation.
This 19-year-old female high-school senior, previously in excellent health, noted a visual disturbance in which she had blurry vision whenever she looked in certain directions. Within 24 hours, this blurriness spread over the entire right hemifield. She consulted her family doctor, who referred her to hospital.
The admitting neurologist’s visual field examination revealed right homonymous hemianopia sparing the uppermost portion of the right hemifield. The remainder of the neurological examination was normal, as were the general physical examination and all routine laboratory tests.
Further tests were performed, including an MRI scan of the head, a CSF examination, and recording of the VEP. All of these tests confirmed the clinical suspicion of an autoimmune inflammatory disease affecting the CNS (multiple sclerosis) producing a lesion along the course of the left optic tract (▶Fig. 4.13). The patient was given cortisone bolus therapy and her symptoms resolved within 3 days.
Fig. 4.13 Inflammatory lesion of the left optic tract in a patient with multiple sclerosis, as revealed by MRI. (a) The T2-weighted coronal image shows a hyperintense lesion along the course of the left optic radiation above the choroidal fissure, sparing only the basal portion of the optic tract (arrow). (b) The T1-weighted coronal image after intravenous administration of contrast material reveals enhancement at this site, indicating a focus of acute inflammation.
Eye Movements (CN III, IV, and VI)
Three cranial nerves innervate the muscles of the eyes: the oculomotor nerve (CN III), the trochlear nerve (CN IV), and the abducens nerve (CN VI) (▶Fig. 4.14 and ▶Fig. 4.15).
The nuclei of the oculomotor and trochlear nerves lie in the midbrain tegmentum, while the nucleus of the abducens nerve lies in the portion of the pontine tegmentum underlying the floor of the fourth ventricle.
The discussion of eye movements in this chapter will begin as simply as possible, i.e., with the movements of a single eye induced by impulses in each of the individual nerves to the eye muscles. It should be borne in mind from the outset, however, that eye movements are usually conjugate, i.e., they usually occur in the same direction (mostly horizontally or vertically) in both eyes at once. Conjugate horizontal movements, in particular, involve simultaneous movement of the two eyes in opposite senses with respect to the midline: one eye moves medially, while the other moves laterally. Conjugate movements thus depend on the precisely coordinated innervation of the two eyes and of the nuclei of the muscles subserving eye movement on the two sides. The complex central nervous connections enabling such movements will be dealt with later in this chapter. Finally, the nerves innervating the eye muscles also take part in a number of reflexes: accommodation, convergence, the pupillary light reflex, and the visual defense reflex. These reflexes will also be discussed in this chapter.
Oculomotor Nerve (CN III)
The nuclear area of the oculomotor nerve lies in the periaqueductal gray matter of the midbrain, ventral to the aqueduct, at the level of the superior colliculi. It has two major components: (1) a medially situated parasympathetic nucleus, the so-called Edinger–Westphal nucleus (or accessory autonomic nucleus), which innervates the intraocular muscles (the sphincter pupillae muscle and the ciliary muscle); and (2) a larger and more laterally situated nuclear complex for four of the six extraocular muscles (the superior, inferior, and medial rectus muscles and the inferior oblique muscle). There is also a small nuclear area for the levator palpebrae muscle (cf. Warwick’s diagram of the simian oculomotor nuclear complex, ▶Fig. 4.16).
The motor radicular fibers that emerge from these nuclear areas travel ventrally together with the parasympathetic fibers; some of them cross the midline, while others do not. The combined motor and parasympathetic fibers traverse the red nucleus and finally exit the brainstem in the interpeduncular fossa as the oculomotor nerve.
The oculomotor nerve first runs posteriorly between the superior cerebellar and posterior cerebral arteries (▶Fig. 4.17), in close apposition to the tentorial edge, then penetrates the dura mater, traverses the cavernous sinus, and enters the orbit through the superior orbital fissure (▶Fig. 4.15 and ▶Fig. 4.17). The parasympathetic portion of the nerve branches off at this point and travels to the ciliary ganglion, where the preganglionic fibers terminate and the ganglion cells give off short postganglionic fibers to innervate the intraocular muscles.
The somatic motor fibers of the oculomotor nerve divide into two branches, a superior branch supplying the levator palpebrae and superior rectus muscles, and an inferior branch supplying the medial and inferior recti and the inferior oblique muscle.
Trochlear Nerve (CN IV)
The nucleus of the fourth cranial nerve lies ventral to the periaqueductal gray matter immediately below the oculomotor nuclear complex at the level of the inferior colliculi. Its radicular fibers run around the central gray matter and cross to the opposite side within the superior medullary velum. The trochlear nerve then exits the dorsal surface of the brainstem (it is the only cranial nerve that does this), emerging from the midbrain tectum into the quadrigeminal cistern. Its further course takes it laterally around the cerebral peduncle toward the ventral surface of the brainstem, so that it reaches the orbit through the superior orbital fissure together with the oculomotor nerve. It then passes to the superior oblique muscle, which it innervates. The eye movements subserved by this muscle include depression of the eye, internal rotation (cycloinversion), and slight abduction.
Abducens Nerve (CN VI)
The nucleus of the sixth cranial nerve lies in the caudal pontine tegmentum, just beneath the floor of the fourth ventricle. The radicular fibers of the seventh cranial nerve (the facial nerve) loop around the nucleus of the abducens nerve at this site. The radicular fibers of the abducens nerve traverse the pons and exit from the brainstem at the pontomedullary junction. The abducens nerve then runs along the ventral surface of the pons lateral to the basilar artery, perforates the dura, and joins the other nerves to the eye muscles in the cavernous sinus. Within the sinus, the third, fourth, and sixth cranial nerves are in a close spatial relation with the first and second branches of the trigeminal nerve, as well as with the ICA (▶Fig. 4.17). Moreover, the nerves in the cavernous sinus lie very near the superior and lateral portions of the sphenoid and ethmoid sinuses (▶Fig. 4.15).
▶Fig. 4.18 depicts the actions of the individual eye muscles in the six diagnostic directions of gaze. ▶Fig. 4.19 shows the abnormalities of eye position and the types of diplopia that are caused by palsy of each of the three nerves subserving eye movements.
Pareses of the Eye Muscles
Weakness of one or more of the extraocular muscles impairs movement of the affected eye and restricts its ability to gaze in a particular direction or directions. The corneal light reflex test, in which the examiner notes the position of the light reflex on both corneas from a point source held in front of the eyes in the midline, often reveals a mild asymmetry, indicating a mild deviation of the visual axis of the eye at rest. A diplopia test with red–green spectacles, or with a light wand, reveals that the image arising from the affected eye is the one that lies in the direction in which that eye is normally moved by the paretic muscle. The two images are farthest apart when the patient gazes in this direction; the more peripheral image is the one arising from the affected eye (▶Fig. 4.19).
Horizontal deviations of eye position are designated esotropia (inward deviation) and exotropia (outward deviation), while vertical deviations are designated hypertropia and hypotropia (upward and downward deviation, respectively).
A lesion in the nucleus of one of the cranial nerves that subserve eye movements causes approximately the same deficit as a lesion of the peripheral nerve itself. Nuclear lesions can usually be clinically distinguished from nerve lesions because of further deficits due to damage of brainstem structures adjacent to the affected nucleus.
Oculomotor Nerve Palsy
A complete oculomotor nerve palsy produces the following constellation of findings (▶Fig. 4.19):
Ptosis, caused by paralysis of the levator palpebrae muscle and unopposed contraction of the orbicularis oculi muscle, which is innervated by the facial nerve (the lid space may be slightly open because of contraction of the frontalis muscle).
Fixed position of the eye, looking downward and outward, caused by unopposed contraction of the lateral rectus and superior oblique muscles (innervated by CN VI and IV, respectively).
Dilation of the pupil, caused by loss of contraction of the sphincter pupillae muscle, innervated by the parasympathetic portion of the oculomotor nerve; the pupillary light and accommodation reflexes are absent (the latter because of simultaneous loss of contraction of the ciliary muscle).
An isolated paralysis of the intraocular muscles, i.e., the sphincter pupillae muscle and the ciliary muscle, is called internal ophthalmoplegia. The globe remains fully mobile, but there is an absolute paralysis of the pupil, i.e., both the direct and the consensual light reflexes are absent, and loss of accommodation causes blurry vision. Internal ophthalmoplegia is due to selective damage of the parasympathetic fibers of the oculomotor nerve.
External ophthalmoplegia is present when the motility of the globe is restricted but the autonomic (parasympathetic) innervation of the eye is preserved.
Oculomotor nerve palsies account for about 30% of all palsies affecting the muscles of eye movement (abducens nerve palsies are more common, accounting for 40–50% of cases). Ptosis is more common with lesions of the (peripheral) nerve itself, while it is rarer with lesions of its nuclear complex within the brainstem. Once the nerve emerges from the brainstem, the pupillomotor fibers lie in the outer portion of the nerve, directly beneath the epineurium, and are thus more vulnerable than the other fibers of the nerve to compression by trauma, tumors, or aneurysms. For the same reason, the pupillomotor fibers are less commonly damaged by vascular lesions, such as those caused by diabetes. The more common causes of isolated oculomotor nerve palsy are aneurysms (~30%), tumors (~15%), and vascular lesions (including diabetes, ~15–20%).
Trochlear Nerve Palsy
Trochlear nerve palsy paralyzes the superior oblique muscle. The affected eye deviates upward and slightly inward, i.e., medially, toward the side of the normal eye (▶Fig. 4.19). The deviation is most evident, and the diplopia most extreme, when the patient looks downward and inward. Another way of bringing out the upward-and-inward deviation of the affected eye and the resulting diplopia is by having the patient tilt the head to the affected side while fixating on an object with the normal eye (Bielschowsky’s test).
The more common causes of trochlear nerve palsy are trauma (30–60% of cases), vascular lesions, and tumors.
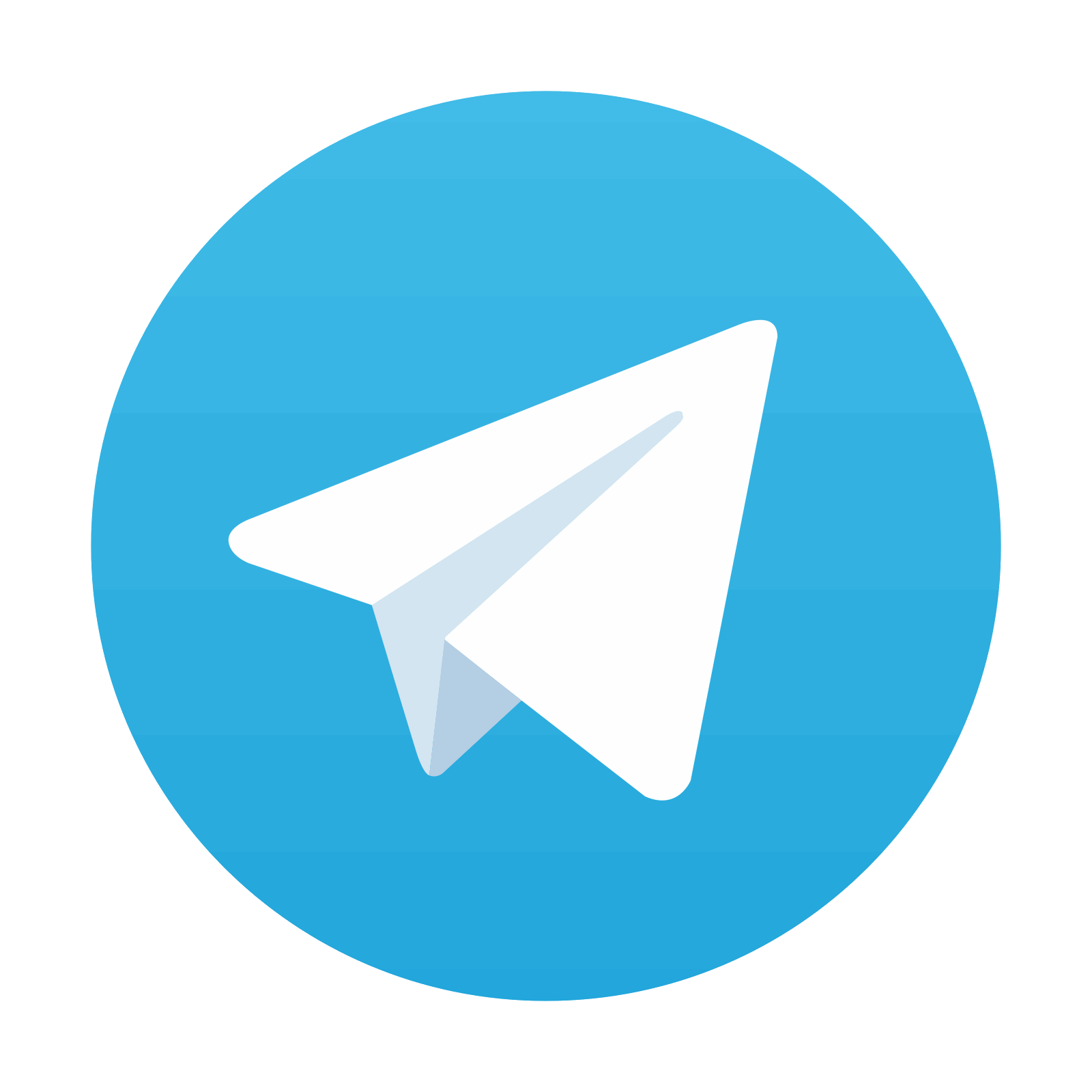
Stay updated, free articles. Join our Telegram channel

Full access? Get Clinical Tree
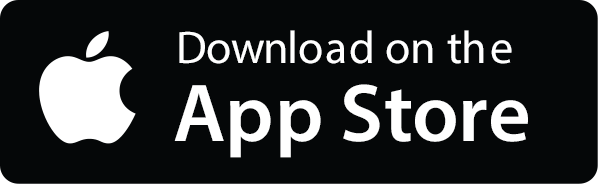
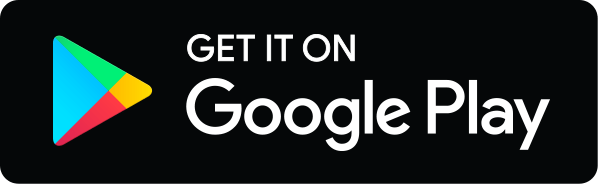