h1 class=”calibre8″>15 Craniovertebral Injuries in Pediatric Patients
Abstract
The craniovertebral junction has a unique and complicated anatomy. The patterns of injury in this location include longitudinal subluxation with failure of stabilization, translational atlantoaxial subluxation, atlantoaxial rotatory fixation, and fractures. However, ligamentous injuries in the absence of fractures are most commonly seen in pediatric patients. The diagnosis and treatment of craniovertebral injuries in pediatric patients are multimodal, requiring an appreciation for the unique anatomy, mechanisms of injury, and developmental considerations of this population.
Keywords: atlantoaxial rotatory fixation, fracture, ligamentous injury, longitudinal, subluxation, translational atlantoaxial
15.1 Craniovertebral Anatomy
The occiput articulates with the cervical spine at C1, the atlas. The bilateral anterior and posterior arches form the ring of the atlas, with the center of each arch indicated by a tubercle (i.e., anterior and posterior tubercles). The lateral edges of the central canal are formed by the lateral masses; the foramen transversarium and the transverse processes are encountered with further lateral progression.
The cervical spine imparts mobility to the head, serves as the point of attachment for key muscles of the back and neck, protects essential vasculature, and houses nerves that innervate critical musculature. Although the cervical spine is not a load-bearing structure at rest, it may function as a shock absorber in cases of blunt force to the skull.
Cephalad to the lateral masses, the atlas contains two large facet joints for the occipital condyles. The occipital condyles are located on the inferior portion of the skull at the occipital bone. Of note, the foramen magnum and medial portion of the posterior fossa are also constituents of the occipital bone. In addition, the basion and opisthion are important landmarks demarcating the midpoint of the anterior and posterior aspects of the foramen magnum, respectively.
The atlanto-occipital joint allows for most of the vertical motion of the head. The posterior surface of the anterior tubercle contains a facet joint to articulate with the dens of C2, the axis. The inferior articulating facets of the atlas and the superior articulating facets of the axis form a synovial joint. The atlantoaxial junction allows for rotational movement of the head and is the only set of two contiguous, unfused vertebrae that does not contain an intervertebral disc. Any load to the atlantoaxial junction is thus transmitted through the lateral masses—unlike in caudal spinal levels, where the load is transmitted through the intervertebral discs and vertebral bodies. 1,2,3,4
An understanding of the anatomy and function of the ligaments that span from the occiput to the axis is critical for understanding the motion of the head and traumatic injury. The apical ligament of the dens is a fibrous midline structure connecting the cephalad portion of the dens to the anterior margin of the foramen magnum. The bilateral alar ligaments span superolaterally from the dens to the occipital condyles, restricting excessive rotation of the head. The transverse ligament of the atlas attaches to the medial surfaces of the C1 lateral masses, forming a strong band that prevents dissociation of the dens. The midline of the transverse ligament has both a superior projection, which connects to the foramen magnum, and an inferior projection, which connects to the body of the axis. The entire structure is known as the cruciform ligament, or cruciate ligament, of the atlas. Furthermore, a ligament known as the tectorial membrane descends from the clivus and travels along the anterior aspect of the central canal, eventually becoming the posterior longitudinal ligament. 5
The axis and each subsequent caudal vertebra down to the sacrum contains an intervertebral disc and bilateral synovial joints articulating two adjacent vertebrae. The foramen transversarium of the first six cervical vertebrae house the vertebral arteries that arise from the first portion of the subclavian artery. The vertebral arteries are divided into four segments: V1 is preforaminal, spanning from its origin at the subclavian artery to the C6 foramen transversarium; V2 is foraminal and extends through the foramina transversaria from C6 to C2; V3 is extradural, spanning from C2 to the dura and V4 is intradural, combining with its contralateral artery to form the basilar artery at the anterior surface of the pons. Caudally, the vertebral arteries have two branches that combine in the midline to form the anterior spinal artery. The dorsal spinal arteries are branches from the posterior inferior cerebellar arteries or, less commonly, direct branches from the vertebral arteries. The single anterior spinal artery and dual posterior spinal arteries supply most of the spinal cord. In the cervical spine, segmental spinal arteries arise from the vertebral and cervical arteries, from posterior intercostal arteries in the thoracic spine, and from lumbar arteries in the abdomen. Branches of the segmental spinal arteries — the anterior and posterior radicular arteries — supply the anterior and posterior nerve roots. The segmental spinal arteries further branch into segmental medullary arteries that join the anterior spinal artery. The largest of the segmental medullary arteries is the artery of Adamkiewicz.
Motor innervation from the cervical spine is pivotal for upper extremity mobility and respiration. The phrenic nerve, from C3 to C5, provides motor innervation to the diaphragm. The brachial plexus is from C5 to T1. In addition, C5 and C6 form most of the axillary nerve, C5 to C7 form the musculocutaneous nerve, C6 to T1 form the median nerve, C5 to T1 form the radial nerve, and C8 and T1 form the ulnar nerve. Abduction of the arm is primarily conducted by the C5 nerve, flexion of the elbow by C6, extension of the elbow by C7, flexion of the digits by C8, and adduction and abduction of the digits by T1. 1
15.2 Embryology and Development
The pattern of ossification that the atlas and axis undergo in early life is an essential consideration in assessing the extent of trauma to the craniovertebral junction. The occipital and first three cervical somites give rise to the craniovertebral junction, with the four occipital somites forming most of the skull base. 2 During embryonic development, the atlas contains a posterior midline synchondrosis between the paired neural arches and paired neurocentral synchondroses between the bilateral neural arches and anterior arch. Ossification of the anterior arch occurs between 3 months and 1 year of age, and ossification of the posterior arch is complete by 3 years of age. The neurocentral synchondroses fuse at about 7 years of age.
The axis undergoes three phases of ossification, with the first at 4 months of fetal life, the second at 6 months of fetal life, and the third at about 3 to 5 years of postnatal life. The first wave of ossification involves the bilateral neural arches and the centrum. The second involves bilateral ossification centers at the basal dental segment. The third ossification center is at the apical dental segment at the tip of the dens. 2
The axis has paired primary ossification centers that fuse in the midline of the dens, as well as primary ossification centers at the neural arch and the body. In addition, a secondary ossification center is at the apex of the dens.
15.3 Mechanisms of Injury
Four primary injury patterns occur in the craniocervical junction of pediatric patients. These patterns are longitudinal subluxation with failure of stabilization, translational atlantoaxial subluxation, atlantoaxial rotatory fixation, and fractures. 2
15.3.1 Longitudinal Subluxation
Longitudinal subluxation of the atlanto-occipital joint occurs because of ligamentous injury, often in high-speed motor vehicle collisions, and can lead to severe damage at the cervicomedullary junction. Atlanto-occipital dislocation (AOD) consists of a disarticulation of the occipital condyles from the atlas. AOD is almost always fatal if left untreated, and instrumented arthrodesis is necessary in all patients who survive. AOD accounts for 35% of fatal cervical spine injuries that result from motor vehicle crashes. 6,7 Three main categories of AOD are anterior displacement of the occiput (type I) (▶ Fig. 15.1a), vertical distraction of the occiput with respect to the atlas (type II) (▶ Fig. 15.1b), and posterior displacement of the occiput with respect to the atlas (type III) (▶ Fig. 15.1c). 2,8
Fig. 15.1 Traynelis classification of atlanto-occipital dislocations. (a) Type I illustrates an anterior displacement (arrow) of the occiput. (b) Type II shows a vertical displacement (arrow). (c) Type III involves posterior displacement (arrow) of the occiput.
(Used with permission from Barrow Neurological Institute, Phoenix, AZ.)
The transverse ligament and the apical ligament of the dens are the strongest ligaments in the atlanto-occipital junction and impart most of its stability. 5,6 Largely due to ligament laxity and the horizontal plane of articular surfaces, AOD is three times more common in pediatric patients than in adults. 2,6,9,10,11,12 Additional predisposing factors may include smaller atlanto-occipital joints and a greater ratio of head weight to body weight, which increases the likelihood of instability at that location. 2,6,7 The diagnosis of AOD is made on the basis of measurement of the basion–dens interval in the sagittal plane or the C1–condyle interval (CCI)—as will be discussed in detail later in this chapter. 2,9
15.3.2 Translational Atlantoaxial Subluxation
Translational atlantoaxial subluxation is due to ligamentous instability between C1 and C2, resulting in anterior displacement of the atlas, relative to the axis. This subluxation is often attributed to injuries of the transverse ligament and may result in spinal cord compression anteriorly from the dens and posteriorly from the posterior arches of the atlas. 2,13 Translational atlantoaxial subluxation is often accompanied by wide fractures of the C1 ring and can be classified into two broad categories based on location. Differentiating type I from type II injuries is crucial in guiding treatment. Type I translational atlantoaxial subluxation necessitates internal fixation, whereas 74% of patients with type II injuries recover with nonoperative conservative management of the injuries. 2,14 Type I injuries are ligamentous, occurring at the midpoint of the transverse ligament (type IA) or at its periosteal insertion site (type IB). Type II injuries occur at the insertion sites of the transverse ligament to the C1 lateral masses and consist of a C1 comminuted fracture at the lateral masses (type IIA) or an avulsion fracture of the C1 tubercle of the lateral mass (type IIB).
15.3.3 Atlantoaxial Rotatory Subluxation
Atlantoaxial rotatory subluxation (AARS) involves varying degrees of atlantal dissociation and rotation and is best described by the Fielding and Hawkins classification, types I to IV (▶ Fig. 15.2). 15 The gold standard for imaging of AARS is three-dimensional computed tomography (CT) that can aid significantly in differentiating each type of AARS. Patients with AARS present with the head tilted toward and rotated away from the affected side. The acute presentation of this deformity must be differentiated from muscular torticollis as well as from possible trauma. In AARS, a reflexive sternocleidomastoid spasm occurs at the side of the chin, whereas in muscular torticollis, a sustained spasm occurs contralaterally.
Fig. 15.2 Fielding and Hawkins classification of atlantoaxial rotatory subluxation. Type I is defined by an intact transverse ligament, type II by disruption of the transverse ligament alone, type III by disruption of the transverse and alar ligaments, and type IV by posterior rotatory displacement of the atlas on C2 in patients with odontoid hypoplasia.
(Used with permission from Barrow Neurological Institute, Phoenix, AZ.)
As described by various authors, the subluxation that occurs in AARS is due to resistance in the natural rotation of the atlantoaxial junction. 2,16,17 The first 23 degrees of rotation in either direction occurs with independent motion of the atlas, relative to the axis. After the initial 23 degrees, tightening of the alar ligaments and nonlinear rotation of the dens accompany the subsequent 42 degrees of rotation. Any rotation after 65 degrees occurs with concurrent motion of the atlas and axis.
Type I Atlantoaxial Rotatory Subluxation
In type I AARS, the atlas has isolated rotation greater than 45 degrees, with the dens functioning as a pivot joint in the absence of any translational displacement. Type I is the most common type of AARS and may be treated by reduction and immobilization alone.
Type II Atlantoaxial Rotatory Subluxation
In type II AARS, the atlas is rotated, with one of the lateral articular processes functioning as the pivot joint and the contralateral articular process being disarticulated. Type II AARS involves atlantal rotation exceeding 40 degrees and a defect of the transverse ligament only. Due to the disruption of the anatomic pivot joint in the atlantoaxial junction, type II AARS typically includes 3 to 5 mm of anterior displacement of the atlas relative to the axis. If diagnosed within 14 days, type II AARS may be treated by reduction and halo immobilization. However, diagnosis more than 14 days after an injury necessitates occipitoatlantoaxial or atlantoaxial fusion.
Type III Atlantoaxial Rotatory Subluxation
Type III AARS involves rotation of the atlas, with disarticulation of both lateral masses and anterior displacement of the atlas exceeding 5 mm relative to the axis. In addition, disruptions of both the alar and transverse ligaments are implicated in type III AARS. This category of AARS is treated with reduction and fusion. 2,15
Type IV Atlantoaxial Rotatory Subluxation
Type IV AARS is similar to type III in that both lateral masses are disarticulated, but type IV involves posterior displacement of the atlas relative to the axis, because of fracture or damage of the dens. 2,15 Similar to type III AARS, type IV is treated with reduction and fusion. 2,15
15.3.4 Fractures in the Craniovertebral Junction
The craniovertebral junction is susceptible to many fracture patterns because of its complex anatomy and range of motion. Traumatic injury in this location, specific to children, often involves a synchondrosis, which typically fuses by 5 to 7 years of age. 2,18 True fracture of the odontoid synchondrosis results in anterior displacement of the dens with no abnormalities in the atlantodental interval. 2,18,19 Although nondisplaced fractures may be difficult to detect, both displaced and nondisplaced synchondrosis fractures are associated with spinal cord injury 53% of the time. 19,20 Prevertebral soft-tissue abnormality on magnetic resonance imaging (MRI) and evidence of synchondrosis widening on CT may be beneficial to diagnose a synchondrosis fracture. Fractures of the dens, as would be seen in adults, may occur in older children and are the most common fractures of the axis. In accordance with the Anderson and D’Alonzo classification, 21 types I, II, and III odontoid fractures occur at the tip of the dens, at the base, and through the body of C2, respectively.
The bilateral neurocentral synchondroses close between 3 and 6 years of age and should not be mistaken for a hangman fracture (i.e., a pars interarticularis fracture) on plain radiographs. Moreover, fractures of the neurocentral synchondroses are analogous to odontoid epiphysiolysis and can be adequately treated with external immobilization. 2,22
Fractures of the atlas represent about 15% of fractures in the cervical spine and may accompany ligamentous injuries such as atlanto-occipital dislocation. 23,24 Fractures of the atlas are best appreciated on CT imaging and may include the anterior arch, posterior arch, lateral masses, or combined arch fractures known as the Jefferson fracture. In the absence of instability, isolated C1 fractures may be conservatively treated with halo immobilization. 23
Pars Interarticularis Fracture
Pars interarticularis fractures, also known as hangman fractures, are injuries that result from hyperextension. Isolated pars fractures do not usually result in neurological injury because the canal typically widens. These fractures are best characterized by anterior or posterior displacement of C2 fragments, based on the Effendi et al 25 classification as modified first in 1985 by Levine and Edwards 26 and then in 2011 by Joaquim and Patel. 23
Type I pars interarticularis fracture
Type I pars interarticularis fractures are hairline fractures of the pars. They result in minimal (< 3 mm) or no displacement or angulation of the axis.
Type II pars interarticularis fracture
Type II pars interarticularis fractures have more than 3 mm of anterolisthesis of C2 with respect to C3, in addition to severe angulation. Angulation with minimal translation is characterized as type IIA.
Type III pars interarticularis fracture
Type III fractures are relatively rare. They involve anterior displacement, with unilateral or bilateral dislocated facet joints and severe angulation. Present in cases of high-velocity trauma with hyperextension and distraction, this type of fracture is a spondylolisthesis of C2 with bilateral pars fractures. As with most traumatic injuries of the craniovertebral junction, surgical indications for C2 pars fractures are dependent on the amount of instability present. Halo traction reduction or rigid orthosis is indicated for pars fractures in the absence of severe angulation or displacement. Surgery is indicated for wide fractures, severe angulation, and facet joint dislocation. 23,27
15.4 Special Considerations
Special attention should be paid to pediatric patients with disorders that may involve the craniovertebral junction. Common examples of these disorders are Down syndrome, Morquio syndrome (and other mucopolysaccharidoses), Klippel–Feil syndrome, and others such as Chiari malformation type I, rheumatoid arthritis, and skeletal dysplasias (all of which are beyond the scope of this discussion).
15.4.1 Down Syndrome
Down syndrome, or trisomy 21, is the most frequent chromosomal disorder. 28 Craniovertebral ligament laxity, particularly of the transverse ligament, may lead to progressive atlantoaxial instability in patients with Down syndrome. As a result, 10 to 30% of patients with Down syndrome have widening of the atlantodental interval that exceeds 5 mm. 29,30,31 In addition to atlanto-occipital hypermobility, 32 patients with Down syndrome may be predisposed to os odontoideum or odontoid hypoplasia with canal stenosis. 32,33,34,35
15.4.2 Morquio Syndrome
Patients with Morquio syndrome (mucopolysaccharidosis type IV) have a mutation in the gene responsible for the production of N-acetylgalactosamine-6-sulfatase (type IVA) or in the gene responsible for beta-galactosidase (type IVB), nearly always resulting in involvement of the craniovertebral junction. 36 Patients with Morquio syndrome are predisposed to atlantoaxial instability from dens hypoplasia, similar to a subset of patients with Down syndrome, but they may also have retrodental soft-tissue masses resulting in canal stenosis. 37
15.4.3 Klippel–Feil Syndrome
This syndrome involves three classic diagnostic criteria: a short neck, a low hairline, and limited neck mobility; all three conditions are present in 50% of patients. 2,38 Klippel–Feil syndrome is a complex disorder that involves vertebral fusion and accelerated spondylosis. Abnormal fusion of the cervical vertebrae increases the moment arm, which may predispose the cervical spine to instability. Patients with Klippel–Feil syndrome, especially those with a traumatic injury, should be carefully evaluated because of the high risk of occipitoatlantoaxial instability and vertebral artery insult. 39,40
15.4.4 Other Disorders
DiGeorge syndrome is a rare congenital anomaly that affects the craniovertebral junction and many other organ systems. More than one-half (59%) of the patients with DiGeorge syndrome have an open posterior arch of C1, 58% have a dysmorphic dens, and 34% have fusion of C2–C3. 2,41 Notable conditions that may predispose patients to craniovertebral instability include Goldenhar syndrome (oculo-auriculo-vertebral syndrome), spondyloepiphyseal dysplasia congenita, osteogenesis imperfecta, and juvenile idiopathic arthritis.
Os odontoideum is an anatomic abnormality wherein a hypoplastic dens is separated from an ossicle of smooth corticated bone of the C2 body. The two variants of os odontoideum are characterized as orthotopic and dystopic. Differentiating between the two has treatment implications. A dystopic os odontoideum is migrated anteriorly and is functionally fused to the basion, whereas an orthotopic os odontoideum moves alongside the anterior arch of C1. 42 An orthotopic os odontoideum can be reduced for normal alignment with the dens.
15.5 Diagnosis of Craniovertebral Injury
Imaging is the mainstay of pediatric craniovertebral injury assessment. Although plain radiographs precede other imaging techniques, the effective diagnosis of craniovertebral injury involves a combination of imaging techniques and multimodal evaluation. In a notable study by Woodring and Lee 43 of 216 patients with traumatic injury to the cervical spine, 61% of fractures and 36% subluxations/dislocations were undetected on anteroposterior and lateral radiographs and on radiographs of the dens. Alternatively, high-resolution CT imaging offers the most meaningful evaluation of osseous structures and interosseous spaces. However, MRI is superior in assessing soft-tissue structures, ligaments, spinal cord, and nerve roots. 2,44
When requesting imaging for pediatric patients with suspected craniovertebral trauma, one should consider the mechanisms of trauma and the most common injuries. Most patients under the age of 10 years who have a craniovertebral injury have ligamentous injury in the absence of fractures. In contrast, in older pediatric patients, fractures are seen in 80% of those who have cervical trauma; the rest have ligamentous injury alone, without a fracture. 45,46 Although radiographic evidence of abnormality or fractures may be absent, further evaluation with MRI is warranted for young children because upper cervical spinal cord injury may occur after trauma. 2
AOD is a potentially life-threatening traumatic condition that warrants careful evaluation. As proposed by Pang, the interval between the CCI should be carefully examined as a site of injury when there is suspicion of traumatic AOD. On a coronal CT, a CCI of 4 mm or greater is an indicator of AOD, with both specificity and sensitivity of 100%. 8,9 Normal CCI joint space is less than 2 mm. The Pang CCI method is preferred over other methods, such as the Sun interspinous ratio, the dens–basion interval, and the Powers ratio, which have sensitivities of 25, 50, and 67%, respectively. 8,9,47
The atlantodental distance can provide insight into translational atlantoaxial subluxation. In children, a horizontal distance exceeding 4 mm on plain radiographs is cause for concern. Additional imaging modalities such as CT and MRI can be used to further identify traumatic injuries of the C1 lateral mass or transverse ligament, respectively. Hyperintensity of the ligament or loss of continuity would be best appreciated on MRI. As previously described, distinguishing type I from type II translational atlantoaxial subluxation injuries is essential in determining further treatment. 2,14,48
Stenosis of the cervical spine is not an uncommon condition, especially in older or larger children. Cervical spinal stenosis may contribute to neurapraxia of the cervical spinal cord in young athletes. Cervical spinal stenosis can be assessed by measuring the anteroposterior diameter of the central canal. A normal diameter is considered to be 17 mm, relative stenosis is 10 to 13 mm, and absolute stenosis is any diameter less than 10 mm. However, the diameter of the canal decreases caudally so that the normal value of C1 is 23 mm, C2 is 20 mm, C3 to C6 is 17 mm, and C7 is 15 mm. A more reliable method is the Torg (i.e., Torg–Pavlov) ratio, wherein the diameter of the central canal is compared to the diameter of the respective vertebral body. 49 A ratio less than 0.8 has high sensitivity but low specificity for cervical spinal stenosis. 49,50
15.6 Treatment
Upper cervical spinal cord injury, or cervicomedullary injury, accompanied by craniovertebral trauma may be life-threatening. In the acute setting, the mean arterial pressure should be elevated to maintain cord perfusion. 51 Although the data on pediatric patients are limited, the current recommendation is not to administer methylprednisolone to treat spinal cord injury, in accordance with the Third National Acute Spinal Cord Injury Randomized Controlled Trial with adult participants. 52
Traction with reduction is a particularly powerful tool to use for realigning the craniovertebral junction in chronic injuries. In acute injury to the craniocervical junction, especially with instability, traction is contraindicated as it could lead to stretching of the neural elements and neurological injury. Pharmacologic muscle relaxation in conjunction with traction restored the alignment in 60% of children who had a presumed “irreducible lesion.” 53 Because of the stretch on the vasculature and spinal cord, intraoperative traction should always be accompanied by adequate neuromonitoring.
Posterior fixation comprises most cases of surgery for craniovertebral injuries (▶ Fig. 15.3, ▶ Fig. 15.4, ▶ Fig. 15.5, and ▶ Fig. 15.6).
Fig. 15.3 Occipitocervical fusion using bone grafts from the ribs, which are wired to the occiput and the cervical laminae.
(Used with permission from Barrow Neurological Institute, Phoenix, AZ.)
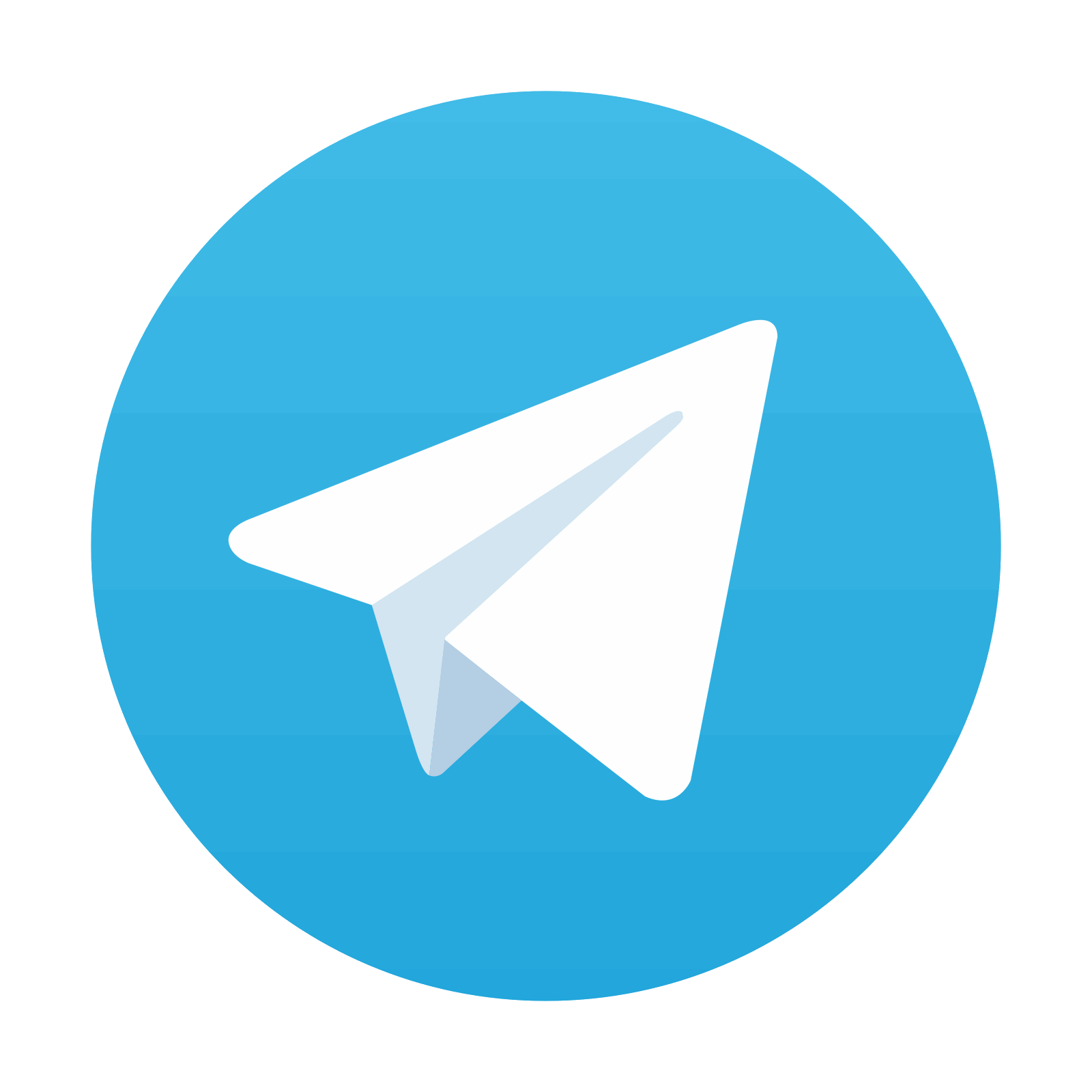
Stay updated, free articles. Join our Telegram channel

Full access? Get Clinical Tree
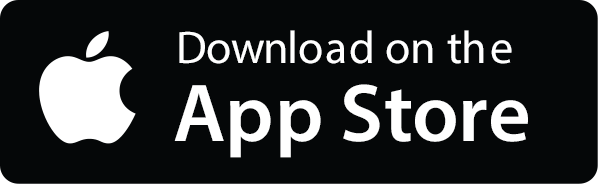
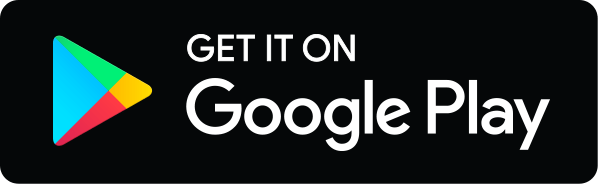