List of abbreviations
AGP
α1-acid glycoprotein
AMPK
adenosine monophosphate-activated protein kinase
AQP4
aquaporin 4
BBB
Basso, Beattie, Bresnahan
ERKs
extracellular signal-regulated kinases
GFAP
glial fibrillary acidic protein
GSK-3β
glycogen synthase kinase 3 beta
HO-1
hemeoxygenase 1
ICE
interleukin 1β converting enzyme
IL
interleukin
IP
intraperitoneal
JAK-STAT
Janus kinase—signal transducer and activator of transcription
MAPK
p38 mitogen-activated protein kinase
MDA
malondialdehyde
MD-2
myeloid differentiation protein 2
NF-H
neurofilament-H
NF-κB
nuclear factor kappa B
NPC
neural progenitor cell
Nrf2/ARE
nuclear erythroid 2-related factor 2/anti-oxidant response element
NSC
neural stem cells
ROS
reactive oxygen species
SCI
spinal cord injury
SC-NPCs
spinal cord neural progenitor cell
SOD
serum superoxide dismutase
SOX-9
sex-determining region Y-box transcription factor 9
STAT
signal transducer and activator of transcriptions
TGF-β
transforming growth factor beta
TNF-α
tumor necrosis factor alpha
Introduction
Traumatic spinal cord injury (SCI) causes necrosis of the central nervous system and subsequent permanent neurological deficit. The mechanism of SCI broadly comprises two stages. First, a primary injury, attributable to the mechanical insult itself and structural damage. A secondary injury is a series of systemic and local neurochemical and physiological changes following the primary injury. The secondary injury occurs via subsequent edema, ischemia, inflammation, cytokine production, free radical damage, glial scar formation, apoptosis, and necrosis ( ). Primary injury is immediate and irreversible; in contrast, a secondary injury worsens with time and necessitates therapeutic intervention ( ; ; ). The secondary injury develops within hours or days after SCI, causing neurochemical alterations that lead to neurologic functional impairments. Therapeutic modalities that promote recovery could be better understood with detailed knowledge of SCI pathophysiology ( ). Neurochemical alterations in SCI include increases in the excitatory amino acids, elevation in the calcium influx, stimulation of the calcium-dependent enzymes, generation of reactive oxygen species (ROS), and release of cytokines leading to neuroinflammation ( ). These neurochemical alterations affect the neuron and astrocyte activity, induce demyelination, modulate leukocyte infiltration, and activate macrophages ( ; ). In addition, secreted inflammatory cytokines and growth factors generally up-regulate the pro-survival molecules, such as nuclear factor kappa B (NF-κB) ( ). Thus, the key obstacle in the treatment and recovery process from catastrophic SCI is gliosis caused by the up-regulation of inflammation. Moreover, several studies have demonstrated the potential therapeutic role of curcumin in alleviating the second injury process.
Curcumin [1,7-bis (4-hydroxy-3-methoxyphenyl)-1,6- heptadiene-3, 5-dione] is a yellow extract obtained from Curcuma longa and is commonly used in India as a seasoning and food-coloring agent ( Fig. 1 ). It is a polyphenol compound that possesses non-steroidal anti-inflammatory properties. Recent studies indicate that it may have anti-oxidant ( ), anti-inflammatory ( ; ; ), neuroprotective ( ) and anti-apoptotic effects ( ) for SCI. Curcumin has emerged as a promising therapeutic drug in SCI treatment with a tendency to reduce the formation of glial scar and suppress the expression of glial fibrillary acidic protein (GFAP), thus contributing to a more favorable recovery environment ( ). In a recent study, curcumin inhibited the hypoxia-induced up-regulation of GFAP and neurofilament-H (NF-H) following hypoxia and down-regulated the expression of pro-inflammatory cytokines, such as tumor necrosis factor alpha (TNF-α) and interleukin 1 (IL-1) ( ).

Structure, pharmacology, and biological targets
Curcumin is a polyphenol substance that has been widely used for medicinal purposes, religious rituals, and local cuisines in the Indian subcontinent. The molecule is symmetric in structure. The keto-enol tautomer in the center, the flexible α,β-unsaturated β-diketo linker, and the terminal o-methoxyphenolic groups comprise the three main components of curcumin molecule ( Fig. 2 ).

Curcumin has a complex pharmacophore that could act as an anti-oxidant, chelate metals, and Michael reaction ( ). Further, curcumin is a hydrophobic molecule comprising two ferulic acid residues linked by a methylene bridge and has high affinity for cellular membranes ( ). Curcumin can participate in hydrogen bonding interactions with its β-diketone moiety and the substituents on the aromatic rings. The aromatic rings can also form π-π Van der Waals interactions ( ). Structure-activity relationships demonstrate that the β-diketone (keto-enol) moiety serves as a chelator for cationic metals present in the protein-binding sites and as a Michael reaction acceptor for nucleophilic groups, such as reduced selenocysteine and sulfhydryl, that form covalent bonds with curcumin ( ). The phenolic hydroxyl group is essential for the anti-oxidant action of curcumin ( ; ). This group and the methylene hydrogen are crucial for free radical scavenging activity, wherein ROS and nitrogen species are subjected to electron transfer or H-atom abstraction ( ). The diversity of the interactions that curcumin offers may explain its binding to multiple proteins ( Fig. 3 ).

Molecular docking studies suggest that curcumin adopts different conformations for the maximization of these interactions, primarily via the α,β-unsaturated β-diketone moiety, and generally favors hydrophilic pockets near the cysteine residues ( ; ). Curcumin shares two important characteristics with the phorbol ester pharmacophore owing to the presence of the hydroxyl and carbonyl groups ( ). Removing the methylene group and carbonyl group and cutting off the pharmacophore produces a more potent molecule 1,5-bis (4-hydroxy-3-methoxiphenyl)-1,4-pentadiene-3-1 that maintains all curcumin activity ( ; ).
Curcumin regulates about 100 biological targets ( ) via various mechanisms, including changing of the activity of cellular proteins via changes in the phosphorylation status ( ). Generally, curcumin demonstrates its effects at concentrations above the micromolar range. This weak binding affinity has facilitated several attempts to optimize the activity of curcumin using a structural-based drug design.
Anti-inflammatory effects
After primary SCI, therapeutic strategies and outcomes depend on how we achieve reduction of inflammation and glial scar. Curcumin is a well-known anti-inflammatory molecule that evokes global inhibition of the inflammation network by suppressing transcription factors, such as NF-κB and signal transducer, as well as the activator of transcriptions (STAT) in the upstream signaling pathways of inflammatory mediators, such as prostaglandins, cytokines, and chemokines ( ). Curcumin may also bind directly to inflammatory mediators and enzymes in downstream inflammation pathways, such as interleukin 1β converting enzyme (ICE), TNF-α, TNF-α converting enzyme (TACE), p38 mitogen-activated protein kinase (MAPK), myeloid differentiation protein 2 (MD-2), α1-acid glycoprotein (AGP), and glycogen synthase kinase 3 beta (GSK-3β) ( ; ). Curcumin inhibited the expression of pro-inflammatory cytokines and suppressed reactive gliosis. Moreover, curcumin inhibited the generation of transforming growth factor beta (TGF-β)1, TGF-β2, and sex-determining region Y-box transcription factor 9 (SOX-9); decreased the deposition of chondroitin sulfate proteoglycan by inhibiting the transforming growth factors and transcription factor; and improved the microenvironment that enabled nerve growth ( ). Occurring concurrently with acute inflammation and preceding fibrosis, spinal cord edema plays a crucial role in neurologic damage and patient symptoms; this is a primary reason behind the clinical usefulness of corticosteroids in SCI patients ( ). An experimental rat model that was administered 40 mg/kg curcumin intraperitoneally (IP) showed reduced hemorrhage, edema, and neutrophil infiltration of the traumatic spinal cord. Curcumin also inhibited the SCI-associated aquaporin 4 (AQP4) overexpression and GFAP as well as repressed the unusual activation of the Janus kinase-signal transducer and activator of transcription (JAK-STAT) signaling pathway ( ). Studies on the effect of curcumin on inflammation, fibrosis, and edema after SCI have been summarized in Table 1 .
Study | Animals/SCI | Curcumin administration | Description of study | Results |
---|---|---|---|---|
( ) | SD rats, N = 128 or clipping | 200 mg/kg IP daily For 8 weeks | Sham ( n = 32) SCI only ( n = 32) SCI & Hyperglycemia ( n = 32) SCI & Hyperglycemia & Curcumin ( n = 32) | Curcumin regulate SOD activity increased, MDA level decreased ED-1 macrophage marker level decreased in the SCI-hyperglycemia-curcumin group Reduction in IL-6, IL-8, and TNF-a levels, the phosphorylated-ERKs Lower lesion volume, Higher spared tissue |
( ) | Wistar rats, N = 131 or balloon compression | Curcumin, 6 mg/kg; EGCG 17 mg/kg IP daily & Curcumin, 60 mg/kg; EGCG 17 mg/kg) IM weekly For 28 days | Behavioral examinations: saline ( n = 10), curcumin ( n = 13), EGCG ( n = 19), curcumin + EGCG ( n = 9) Cytokine group studies: Saline ( n = 20), curcumin (n = 20), EGCG ( n = 20), curcumin + EGCG ( n = 20) | Curcumin and EGCG alone or in combination increased axonal sprouting, decreased glial scar formation, and altered the levels of macrophage inflammatory protein 1-alpha, IL-1β, IL-4, and IL-6 |
( ) | Wistar rats, N = 135 or balloon compression | 60 mg/kg IT weekly & 6 mg/kg IP daily For 28 days | Saline ( n = 34), Curcumin ( n = 27), MSC ( n = 28), Curcumin + MSC ( n = 26) | Curcumin + MSC facilitated axonal sprouting, and modulated expression of pro-regenerative factors and inflammatory responses |
( ) | SD rats, N = 280 or blunt dissection and clipping | 100 mg/kg IP daily For 7 days | Sham group ( n = 70), SCI group ( n = 70), SCI + curcumin group ( n = 70), SCI + DMSO group ( n = 70) | Curcumin regulate both the NF-κb and SOX9 signaling pathways and reduce the expression of intracellular and extracellular glial scar components through dual-target regulating both inflammation and fibrosis after SCI in the rat |
( ) | SD rats, N = 48 or clipping | 100 mg/kg IP at 15 min after SCI | Sham group ( n = 16), SCI group ( n = 16), SCI + curcumin group ( n = 16). N = 16 per group | Curcumin markedly down regulated the levels of TLR4/NF-κB inflammatory signaling pathway. Significantly ameliorated SCI induced spinal cord edema, and apoptosis |
( ) | Wild-type C57BL/6JNarl mice, N = 18 or weight drop | 40 mg/kg IP at 30 min after SCI | Sham control ( n = 6), SCI ( n = 6), SCI + curcumin ( n = 6) | Curcumin can attenuate the down-regulation of CISD2 (CISD2 exerts anti-apoptotic and anti-inflammatory effects in neural cells) in SCI and LPS-treated astrocytes |
Anti-oxidant effects
Several trials have assessed the anti-oxidant properties of curcumin for SCI. There is a close association between SCI and free radical production, owing to inflammation ( ). In an experimental study on the anti-oxidant effect of curcumin on SCI in rats, serum superoxide dismutase (SOD) level in the curcumin group (dose: 200 mg/kg/d orally) was higher than that in the control group and methylprednisolone group (dose: 30 mg/kg intraperitoneally). The malondialdehyde (MDA) level in the curcumin group was lower than in that the control group. Further, curcumin effectively protects the spinal cord tissues against oxidative damage ( ). Curcumin acts as a free radical scavenger by donating protons to ROS and nitrogen species and quenches them via electron transfer and H-atom abstraction ( ). Consequently, oxidative damage, such as lipid peroxidation is inhibited in the brain and the tissues ( ; ). Curcumin stimulates the intracellular anti-oxidant defense response via the indication of expression of several anti-oxidants, detoxification, and cytoprotective proteins via the unsilencing of relevant genes on the activation of the nuclear erythroid 2-related factor 2 or anti-oxidant response element (Nrf2/ARE) pathway ( ). Curcumin can boost the activities of anti-oxidant enzymes, such as plasma catalase, erythrocyte SOD, and plasma glutathione peroxidase ( ). We have presented the anti-oxidant properties of curcumin as found in SCI studies in Table 2 .
Study | Animals or cells/SCI | Curcumin administration | Description of study | Results |
---|---|---|---|---|
( ) | Wistar rats, N = 40 or ischemia by clamping the aorta | 100 mg/kg IP at 30 min before ischemia | Sham ( n = 10), Ischemia-reperfusion ( n = 10), Curcumin ( n = 10), Solvent ( n = 10) | Curcumin regulates MDA levels in the spinal cord decreased SOD and GPx levels increased |
( ) | HA-sp astrocytes | Cells were treated with curcumin for 24 h | H 2 O 2 was used to induce oxidative stress in astrocytes 50 μM of H 2 O 2 or 1 μM of curcumin or 50 μM of H 2 O 2 + 1 μM of curcumin | Curcumin inhibits oxidative stress-induced cytoskeleton disarrangement, and impedes the activation of astrocytes by inhibiting up-regulation of GFAP, vimentin and Prdx6 Inhibition of oxidative stress-induced inflammation, apoptosis and mitochondria fragmentation after curcumin treatment |
( ) | Wistar rats, N = 40 or weight drop | 300 mg/kg Single IP injection Directly after SCI | Control ( n = 8), SCI alone ( n = 8), Methylprednisolone sodium succinate (30 mg/kg) ( n = 8), Curcumin + DMSO (300 mg/kg) ( n = 8), DMSO alone (0.1 mg/kg) ( n = 8) | Decreased lipid peroxidation and MDA levels in curcumin group |
( ) | Wistar rats, N = 24 or weight drop | 200 mg/kg/d orally | SCI + no medication ( n = 8), SCI + curcumin (200 mg/kg/d orally) ( n = 8), SCI + methylprednisolone (30 mg/kg, IP) ( n = 8) | SOD level in the curcumin group was higher than in the control group and methylprednisolone group MDA level in the curcumin group was lower than in the control group |
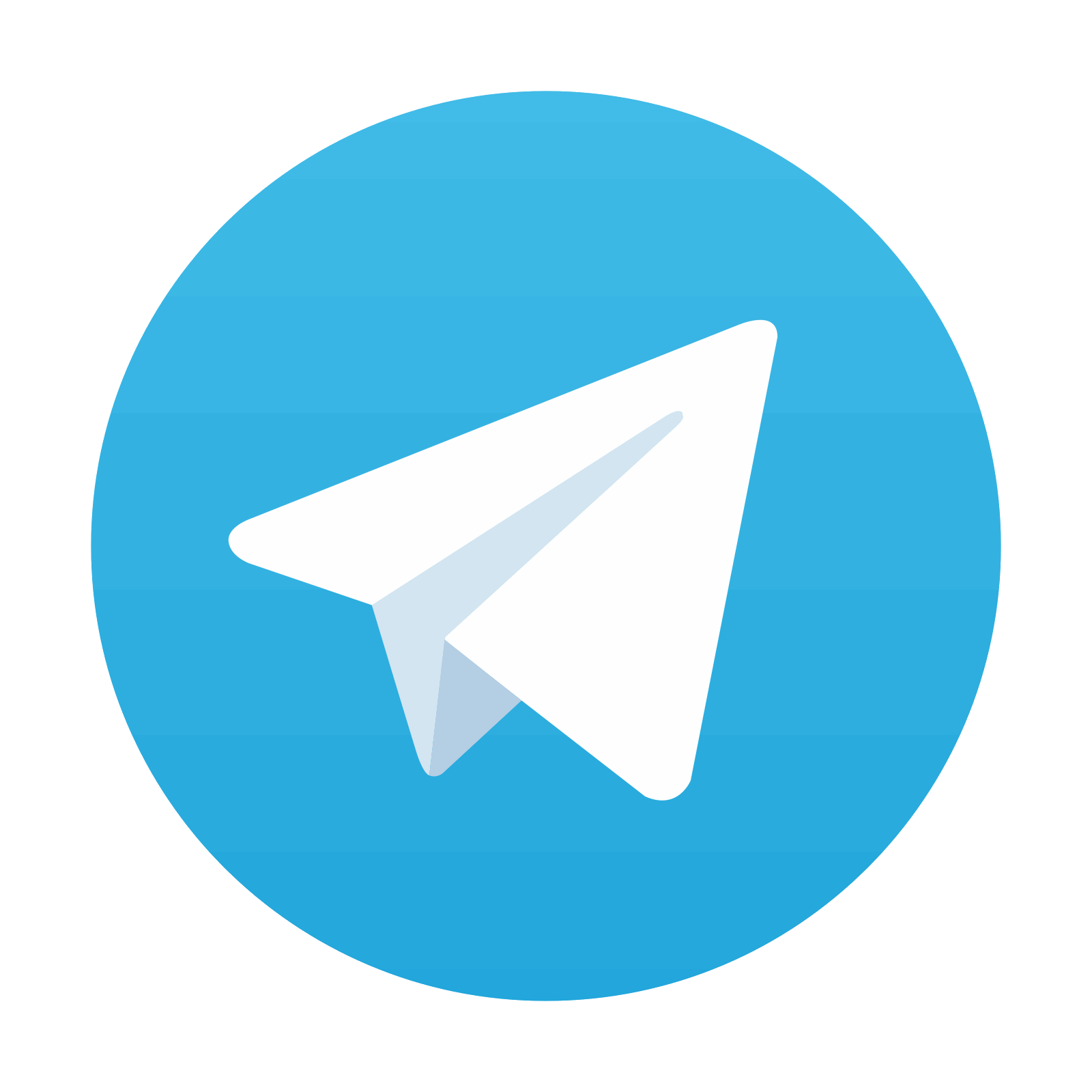
Stay updated, free articles. Join our Telegram channel

Full access? Get Clinical Tree
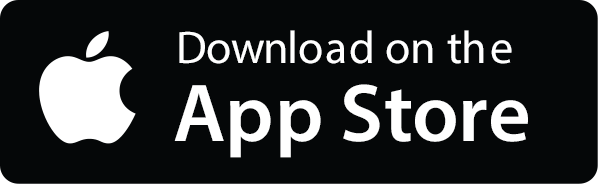
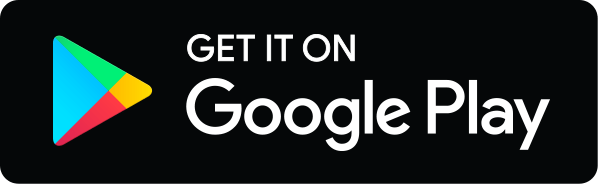
